Volatile composition of Tempranillo, Moribel, and Tinto Fragoso grape musts.
Abstract
This chapter synthetizes the main results that our research group has obtained about the specific influence of a commercial Saccharomyces cerevisiae strain on the aromatic profile of fermented musts from four minority grape varieties (Vitis vinifera L.) cultivated in Castilla-La Mancha (Spain), that is, Moribel, Tinto Fragoso, Albillo Dorado and Montonera del Casar. In addition, wines made from the grape cultivars Tempranillo and Airén were evaluated. To determine the main yeast-derived odor relevant in these grape varieties, the aromatic profiles of grape cultivars and the resulting wines were studied by gas chromatography coupled to mass spectrometry and wines were subjected to Napping, a rapid sensory evaluation method. The results revealed wine sensory differences which are consequence of different aromatic profiles of wines produced with these grape cultivars. The combination of quantitative chemical analysis of volatile compounds together with sensory analysis of wines point out different patterns of aroma compound formation and release. Thus, the yeast strain used in the fermentation step is one of the main factors that affect the sensory properties of wines.
Keywords
- Saccharomyces cerevisiae
- yeast
- grape
- wine
- volatile compounds
- aroma
- gas chromatography
- sensory analysis
1. Introduction
Wine is a beverage obtained usually from grapes via the alcoholic fermentation process carried out by yeasts. The use of commercial
Spain is the country with the biggest vineyard surface in the world and has a great varietal biodiversity [8], despite the disappearance of many grape varieties (
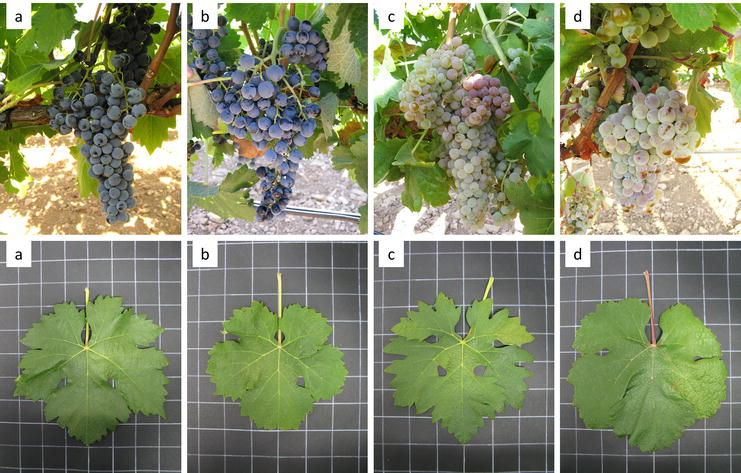
Figure 1.
Leaf and bunch morphology in the
The aim of the chapter is to synthesize the main results that our research group has obtained on the impact of a commercial
2. Materials and methods
2.1 Chemicals
All solvents and reagents were of analytical grade (>99%) and a Milli-Q purification system (Merck-Millipore, Darmstadt, Germany) was used to obtain the pure water. Several commercial standards were employed for volatile compound identification and quantification purposes, which were purchased from Extrasynthese (Genay, France), Fluka (Buchs, Germany), Merck (Darmstard, Germany), and Sigma-Aldrich Chemie (Steinheim, Germany). 4-nonanol and 4-methyl-2-pentanol were used as internal standards and were supplied by Sigma-Aldrich Chemie (Steinheim, Germany).
2.2 Grape samples
Grape samples of four minority varieties (
2.3 Winemaking process
Winemaking process was carried out in the winery of IRIAF and all produced wines were dry, i.e. containing less than 5 g/L of residual sugar. White wines were elaborated from 75 kg of grapes. The grapes were destemmed and crushed, with the addition of 80 mg/L of SO2. Cold pre-fermentation maceration was developed at 5°C for 24 h. Subsequently, the resultant grape must was separated from the solid phase by pressing, and total acidity was adjusted to 4.5 g/L in the case of grape must with lower values. In addition, ascorbic acid (100 mg/L) and lysozyme (100 mg/L) were added to avoid oxidation and malolactic fermentation respectively. Fermentation was carried out at 17°C in 100 L fermentation vessels using the commercial yeast Uvaferm VN® (Lallemand Inc., Zug, Switzerland) at 20 g/hL. Alcoholic fermentation was controlled by measures of the density. When a relative density of 1.010 was reached, the alcoholic fermentation continued at 20°C. When the glucose + fructose concentration was below 5 g/L, the alcoholic fermentation was terminated. Then, the wines were racked and sulphited (25 mg/L of free SO2). For 1 month, the fermentation lees were stirred regularly (
Red wines were elaborated from 75 kg of grapes in vats of 100 L, with skin maceration until the alcoholic fermentation ended. After stemming and crushing, a concentration of 50 mg/L of SO2 was added before the inoculation with
2.4 Volatile compound extraction
For each variety, an amount of 500 g of grapes was selected and crushed for 3 min with an ULTRA-TURRAX digital T50 crusher and subsequently centrifuged at 3500 rpm for 15 min. The obtained supernatant was then filtered, disregarding the first wort fraction. A volume of 25 mL was collected and 500 μL of 4-nonanol 0.1 g/L (internal standard) was added. The extraction of the free fraction of volatile compounds was performed following the procedure described in the literature [12], using SPE cartridges (LiChrolut EN, Merck, 0.2 g phase for wine, 0.3 g phase for grape must). A volume of 25 mL of grape must or wine was selected and passed through the SPE resin with the internal standard. Subsequently, 25 mL of Milli-Q water was added to remove polar compounds and sugars. The free fraction of volatile compounds was recovered by passing 15 mL of a pentane-dichloromethane solution (2:1, v/v). The eluate obtained was concentrated by distillation on a Vigreux column and then under a nitrogen stream to 150 μL, keeping at −20°C until analysis. The glycosidically bound volatile fraction of grapes was obtained by passing 25 mL of ethyl acetate-methanol (9:1, v/v) after recovering the aroma-free portion from the SPE resin. Subsequently, the obtained fraction was evaporated to dryness in a rotary evaporator and reconstituted with 5 mL of 0.2 M citrate buffer (pH = 5.00). Glycosidic aromatic precursors were released by enzymatic hydrolysis. The reconstituted sample was thawed and 250 mg of enzyme Lallzyme® BETA (Lallemand Inc., Zug, Switzerland) was added, remaining tightly covered in an oven at 40°C for 18 hours. Then it was left to cool and 500 μL of 4-nonanol 0.1 g/L was added. The process of volatile compound extraction described above was repeated once again, using SPE cartridges of 0.2 g of phase and 5 mL capacity.
2.5 GC-MS analysis of volatile compounds
Volatile compound analysis was performed with a Focus GC gas chromatograph system coupled to a mass spectrometer (ISQ , ThermoQuest, Waltham, MA, USA). A BP21, polyethylene glycol treated with nitroterephthalic acid, capillary column (50 m × 0.32 mm i.d.; 0.25 μm thick of Free Fatty Acid Phase (FFAP)) was used. For major volatile compounds, 0.8 μL of a mix solution (100 μL of wine sample, 100 μL of 4-methyl-2-pentanol (50 mg/L) as internal standard, and 1 mL of Milli-Q water) were injected in split mode. The gas chromatographic conditions were as follows: helium was used as carrier gas with a constant flow of 1.2 mL/min, the injector temperature was 195°C and oven temperature program was: 32°C (2 min), 5°C/min to 120°C, 75°C/min to 190°C, and 18 min at 190°C. Minor volatile compounds were analyzed by injecting 1 μL of sample in splitless mode after solid phase extraction (SPE). Operating conditions were as follows: carrier gas was helium (1 mL/min); injector temperature, 220°C; and oven temperature program was: 40°C for 15 min, 2°C/min to 100°C, 1°C/min to 150°C, 4°C/min to 210°C and 55 min at 210°C. The mass spectrometer operated in the electron impact mode with an electron energy of 70 eV, ion source temperature 250°C, and the global run time was recoded in full scan mode (mass scanning range, 40–250 amu). The identification of volatile compounds was performed by chromatographic retention times and mass spectra using commercial standards. These compounds were quantified by analyzing the characteristic m/z fragment for each compound following the internal standard method. When the commercial standards were not available, the volatile compound concentration was expressed as internal standard equivalents obtained by normalizing the compound peak area to that of the internal standard, multiplying by the internal standard concentration.
2.6 Odor activity values
The Odor Activity Values (OAVs) were determined to evaluate the contribution of a chemical compound to the wine aroma, providing the importance of a specific component to the sample odor. OAVs were calculated as the ratio between the individual compound concentration and the perception threshold found in literature [13, 14]. A possible contribution to the aroma of wine was considered when OAV was higher than 0.1.
2.7 Wine sensory analysis
Wines were sensorial evaluated by a trained panel of 11 experienced tasters from the IRIAF. The assessment was carried out under ISO standards related to taster selection and training [15], methodology and vocabulary [16], and tasting room [17], following the Napping technique [18]. Wine-taster members were asked to smell and taste the samples and to place them on white sheet of 40 × 60 cm, according to their similarities and dissimilarities. Samples close together on the sheet had similar sensory properties but if tasters perceived samples very differently, they had to place them far from each other. A second session was carried out providing a list of attributes imposed by the judge-in-chief and previously chosen by an expert panel. Napping sensory maps were obtained by Multiple Factorial Analysis (MFA) which provides useful information on the general perception of evaluated wines.
2.8 Statistical analysis
The statistical treatments were performed using the SPSS software version 23.0 (SPSS Inc., Chicago, Illinois, USA) and XLSTAT 2017 statistical software (Addinsoft, Paris, France). To determine statistically significant differences in the concentration of volatile compounds of wine aroma, a one-way analysis of variance (ANOVA, Student–Newman–Keuls/Tukey test, p < 0.05) was made. Sensory data were subjected to MFA to obtain the Napping sensory maps of wines.
3. Results and discussion
3.1 Aroma composition of grapes
The study of the aroma composition of these grapes allowed us to identify and quantify the free and bound volatile fractions usually located in berry skins by GC-MS (Tables 1 and 2).
Compounds | Tempranillo | Moribel | Tinto Fragoso | |||
---|---|---|---|---|---|---|
Free | Bound | Free | Bound | Free | Bound | |
1-Hexanol1 | 0.31 ± 0.02 b | 0.15 ± 0.03 a | 0.34 ± 0.03 b | 0.08 ± 0.01 a | 0.61 ± 0.09 c | 0.14 ± 0.02 a |
5.72 ± 0.27 a | ND | 5.95 ± 0.45 a | ND | 11.51 ± 2.70 b | ND | |
0.21 ± 0.02 d | 0.08 ± 0.00 b | 0.36 ± 0.03 e | 0.05 ± 0.01 a | 0.14 ± 0.02 c | 0.03 ± 0.00 a | |
0.29 ± 0.03 d | 0.03 ± 0.00 a | 0.24 ± 0.02 c | 0.01 ± 0.00 a | 0.52 ± 0.03 e | 0.07 ± 0.01 b | |
7.45 ± 0.50 d | 2.55 ± 0.19 b | 4.67 ± 0.38 c | 1.45 ± 0.45 a | 8.48 ± 0.84 e | 2.26 ± 0.37 ab | |
Hexanal1 | 1.50 ± 0.07 d | 0.12 ± 0.01 a | 1.34 ± 0.11 c | 0.08 ± 0.00 a | 0.96 ± 0.11 b | 0.06 ± 0.01 a |
9.96 ± 1.00 a | ND | 8.88 ± 0.96 a | ND | 10.28 ± 1.26 a | ND | |
0.12 ± 0.01 ab | 0.16 ± 0.02 c | 0.17 ± 0.01 c | 0.09 ± 0.01 a | 0.13 ± 0.01 b | 0.11 ± 0.02 ab | |
82.61 ± 4.95 d | 0.95 ± 0.21 a | 66.07 ± 12.07 c | 0.68 ± 0.07 a | 34.77 ± 7.63 b | 0.68 ± 0.13 a | |
2.54 ± 0.15 b | 0.54 ± 0.07 a | 2.52 ± 0.13 b | 0.30 ± 0.01 a | 2.42 ± 0.27 b | 0.41 ± 0.06 a | |
Linalool2 | 0.60 ± 0.15 ab | 0.81 ± 0.24 b | 0.25 ± 0.05 a | 0.67 ± 0.35 ab | 0.57 ± 0.01 ab | 0.49 ± 0.14 ab |
Nerol2 | 0.43 ± 0.07 a | 6.88 ± 0.56 c | 0.33 ± 0.05 a | 3.02 ± 0.36 b | 0.81 ± 0.10 a | 7.40 ± 0.96 c |
Geraniol2 | 1.35 ± 0.28 a | 28.23 ± 2.48 b | 1.57 ± 0.31 a | 12.66 ± 1.89 a | 6.85 ± 0.61 a | 51.20 ± 11.15 c |
2.37 ± 0.34 a | 35.91 ± 3.26 c | 2.15 ± 0.37 a | 16.35 ± 2.20 b | 8.22 ± 0.71 ab | 59.09 ± 11.87 d | |
3-Oxo-α-ionol1 | ND | 0.15 ± 0.02 b | ND | 0.08 ± 0.01 a | ND | 0.08 ± 0.01 a |
β-Ionol2 | ND | 16.51 ± 1.29 c | ND | 2.96 ± 0.20 a | ND | 6.80 ± 0.83 b |
ND | 0.17 ± 0.02 b | ND | 0.09 ± 0.01 a | ND | 0.08 ± 0.02 a | |
Benzaldehyde2 | 5.41 ± 0.77 a | 31.71 ± 6.41 bc | 6.77 ± 0.39 a | 36.18 ± 3.46 c | 5.51 ± 1.06 a | 27.87 ± 2.21 b |
Benzyl alcohol1 | 0.01 ± 0.00 a | 1.12 ± 0.22 c | 0.08 ± 0.00 a | 1.50 ± 0.20 d | 0.02 ± 0.00 a | 0.72 ± 0.04 b |
Phenylacetaldehyde2 | 3.63 ± 1.08 ab | 4.32 ± 0.51 ab | 6.99 ± 0.53 c | 3.17 ± 0.54 a | 5.72 ± 1.96 bc | 2.74 ± 0.31 a |
2-Phenylethanol1 | 0.02 ± 0.00 a | 0.57 ± 0.07 c | 0.19 ± 0.03 b | 0.58 ± 0.03 c | 0.01 ± 0.00 a | 0.19 ± 0.01 b |
Eugenol2 | ND | 14.80 ± 1.88 b | ND | 2.84 ± 0.62 a | ND | 26.30 ± 1.83 c |
Vanillin1 | 0.01 ± 0.00 a | 0.05 ± 0.00 b | 0.11 ± 0.04 c | 0.04 ± 0.00 ab | 0.01 ± 0.00 a | 0.02 ± 0.00 ab |
Acetovanillone1 | 0.01 ± 0.00 a | 0.15 ± 0.03 c | 0.01 ± 0.00 a | 0.08 ± 0.00 b | 0.00 ± 0.00 a | 0.15 ± 0.01 c |
0.04 ± 0.00 a | 1.94 ± 0.31 d | 0.40 ± 0.06 b | 2.24 ± 0.24 e | 0.05 ± 0.01 a | 1.13 ± 0.04 c | |
1-Butanol2 | 12.23 ± 1.25 a | 98.88 ± 4.09 e | 20.17 ± 1.73 b | 32.81 ± 1.74 c | 30.76 ± 2.62 c | 38.28 ± 4.27 d |
3-Methyl-2-butanol1 | ND | 0.08 ± 0.01 b | ND | 0.03 ± 0.00 a | ND | 0.03 ± 0.00 a |
3-Methyl-3-butanol1 | 1.79 ± 0.12 b | 0.25 ± 0.02 a | 4.53 ± 0.39 c | 0.11 ± 0.01 a | 2.48 ± 0.73 b | 0.05 ± 0.01 a |
1-Pentanol2 | 6.63 ± 0.86 a | 40.70 ± 1.82 c | 9.78 ± 0.93 a | 25.48 ± 0.84 b | 7.98 ± 0.54 a | 26.45 ± 3.73 b |
2-Ethyl-1-hexanol1 | 0.01 ± 0.00 bc | 0.04 ± 0.00 e | 0.02 ± 0.00 cd | 0.02 ± 0.00 d | 0.01 ± 0.00 e | 0.01 ± 0.00 a |
1-Octanol2 | 1.07 ± 0.11 a | 11.97 ± 1.31 d | 1.40 ± 0.26 a | 5.68 ± 0.42 c | 1.32 ± 0.19 a | 3.37 ± 0.44 b |
3-Octanol2 | 2.58 ± 0.09 b | 2.51 ± 0.11 b | 2.66 ± 0.05 b | 2.49 ± 0.16 b | 2.59 ± 0.02 b | 2.12 ± 0.17 a |
0.04 ± 0.00 a | 0.52 ± 0.03 d | 0.05 ± 0.00 a | 0.23 ± 0.01 c | 0.06 ± 0.01 a | 0.15 ± 0.02 b | |
2.62 ± 0.16 bc | 3.20 ± 0.43 c | 2.98 ± 0.08 bc | 2.88 ± 0.26 bc | 2.53 ± 0.27 b | 1.83 ± 0.11 a |
Table 1.
mg/L.
μg/L.
Abbreviation: ND = not detected.
Different letters in the same row indicate significant differences (ANOVA, Student–Newman–Keuls test,
Mean value ± standard deviation, n = 3.
Adapted from Pérez-Navarro et al. [19].
Compounds | Airén | Albillo Dorado | Montonera del Casar | |||
---|---|---|---|---|---|---|
Free | Bound | Free | Bound | Free | Bound | |
1-Hexanol1 | 0.17 ± 0.01 c | 0.03 ± 0.00 a | 0.27 ± 0.06 d | 0.10 ± 0.02 b | 0.46 ± 0.04 e | 0.04 ± 0.00 a |
5.28 ± 0.26 a | ND | 4.49 ± 0.84 a | ND | 31.17 ± 2.79 b | ND | |
0.45 ± 0.02 e | 0.03 ± 0.00 a | 0.37 ± 0.03 d | 0.08 ± 0.01 b | 0.16 ± 0.02 c | 0.01 ± 0.00 a | |
0.25 ± 0.03 b | 0.01 ± 0.00 a | 0.27 ± 0.01 b | 0.02 ± 0.00 a | 0.60 ± 0.04 c | 0.02 ± 0.00 a | |
3.80 ± 0.22 b | 0.93 ± 0.35 a | 6.17 ± 1.56 c | 1.86 ± 0.93 a | 12.08 ± 1.15 d | 0.94 ± 0.32 a | |
Hexanal1 | 0.84 ± 0.04 b | 0.06 ± 0.00 a | 1.10 ± 0.14 c | 0.06 ± 0.00 a | 1.24 ± 0.12 d | 0.06 ± 0.00 a |
7.29 ± 0.15 a | ND | 10.89 ± 0.27 c | ND | 10.26 ± 0.18 b | ND | |
0.19 ± 0.01 b | 0.08 ± 0.01 a | 0.15 ± 0.00 c | 0.19 ± 0.02 d | 0.11 ± 0.02 b | 0.17 ± 0.02 c | |
56.35 ± 1.83 b | 0.51 ± 0.11 a | 79.79 ± 8.79 c | 0.44 ± 0.12 a | 84.79 ± 8.34 c | 0.46 ± 0.06 a | |
1.90 ± 0.11 c | 0.21 ± 0.02 a | 2.27 ± 0.13 d | 0.45 ± 0.03 b | 2.72 ± 0.18 e | 0.30 ± 0.03 ab | |
Linalool2 | 0.26 ± 0.02 a | 0.62 ± 0.11 a | 0.24 ± 0.03 a | 0.55 ± 0.35 a | 0.25 ± 0.02 a | 0.62 ± 0.18 a |
Nerol2 | 0.39 ± 0.04 a | 6.27 ± 0.55 d | 0.36 ± 0.06 a | 2.81 ± 0.23 b | 0.65 ± 0.08 a | 4.51 ± 0.44 c |
Geraniol2 | 1.39 ± 0.26 a | 19.14 ± 2.16 b | 3.16 ± 0.38 a | 21.27 ± 7.77 b | 2.09 ± 0.63 a | 38.65 ± 4.35 c |
2.04 ± 0.26 a | 26.02 ± 1.91 b | 3.76 ± 0.41 a | 24.63 ± 7.93 b | 3.83 ± 0.98 a | 43.79 ± 4.83 c | |
3-Oxo-α-ionol1 | ND | 0.04 ± 0.01 a | ND | 0.13 ± 0.01 c | ND | 0.07 ± 0.01 b |
β-Ionol2 | ND | 5.20 ± 0.78 b | ND | 14.91 ± 0.87 c | ND | 1.71 ± 0.20 a |
ND | 0.05 ± 0.02 a | ND | 0.14 ± 0.01 c | ND | 0.07 ± 0.01 b | |
Benzaldehyde2 | 4.40 ± 0.50 a | 16.39 ± 1.78 a | 7.76 ± 0.69 a | 25.49 ± 1.32 a | 17.76 ± 0.87 a | 498.96 ± 32.79 b |
Benzyl alcohol1 | 0.01 ± 0.00 a | 0.49 ± 0.03 b | 0.01 ± 0.00 a | 0.61 ± 0.03 c | 0.02 ± 0.00 a | 0.84 ± 0.14 d |
Phenylacetaldehyde2 | 4.76 ± 0.34 b | 2.65 ± 0.40 a | 5.11 ± 0.08 b | 2.81 ± 0.26 a | 12.27 ± 1.99 c | 3.79 ± 0.27 ab |
2-Phenylethanol1 | 0.01 ± 0.00 a | 0.25 ± 0.03 b | 0.02 ± 0.00 a | 0.46 ± 0.04 d | 0.03 ± 0.00 a | 0.34 ± 0.03 c |
Eugenol2 | ND | 5.50 ± 1.56 b | ND | 2.09 ± 0.36 a | ND | 1.00 ± 0.22 a |
Vanillin2 | 6.75 ± 2.39 a | 24.71 ± 6.55 b | 27.68 ± 8.64 b | 9.93 ± 1.05 a | 66.42 ± 11.29 c | 9.37 ± 0.65 a |
Acetovanillone2 | 0.89 ± 0.58 a | 79.25 ± 10.71 c | 4.16 ± 0.69 a | 62.71 ± 3.57 b | 5.27 ± 1.45 a | 55.52 ± 5.86 b |
0.03 ± 0.00 a | 0.87 ± 0.06 b | 0.08 ± 0.01 a | 1.17 ± 0.07 c | 0.15 ± 0.01 a | 1.74 ± 0.17 d | |
1-Butanol2 | 2.72 ± 0.27 a | 6.92 ± 1.08 b | 23.10 ± 2.22 d | 45.67 ± 1.36 e | 6.35 ± 0.29 b | 10.65 ± 1.23 c |
3-Methyl-2-butanol2 | ND | 48.19 ± 3.81 a | ND | 44.88 ± 4.71 a | ND | 47.28 ± 1.87 a |
3-Methyl-3-butanol2 | 1.74 ± 0.24 a | 94.26 ± 9.15 b | 2.05 ± 0.12 a | 104.86 ± 14.51 b | 2.41 ± 0.17 a | 92.79 ± 10.81 b |
1-Pentanol2 | 5.96 ± 0.88 a | 15.88 ± 2.65 c | 9.80 ± 1.12 b | 28.06 ± 1.94 e | 9.12 ± 0.25 b | 19.28 ± 2.00d |
2-Ethyl-1-hexanol2 | 9.94 ± 0.39 a | 22.60 ± 1.69 c | 11.58 ± 0.73 a | 27.08 ± 3.29 d | 14.82 ± 0.63 b | 22.60 ± 0.59 c |
1-Octanol2 | 1.15 ± 0.24 a | 3.42 ± 0.77 b | 1.37 ± 0.20 a | 3.43 ± 1.07 b | 1.15 ± 0.14 a | 2.52 ± 0.37 ab |
3-Octanol2 | 2.14 ± 0.05 a | 2.38 ± 0.10 b | 2.61 ± 0.12 b | 2.40 ± 0.16 b | 2.61 ± 0.07 b | 2.02 ± 0.13 a |
0.02 ± 0.00 a | 0.19 ± 0.01 c | 0.05 ± 0.00 b | 0.25 ± 0.02 d | 0.04 ± 0.00 ab | 0.20 ± 0.02 c | |
1.96 ± 0.11 b | 1.34 ± 0.07 a | 2.40 ± 0.91 c | 2.03 ± 0.09 b | 2.91 ± 0.17 d | 2.35 ± 0.21 c |
Table 2.
Volatile composition of Airén, Albillo Dorando, and Montonera del Casar grape musts.
mg/L.
μg/L.
Abbreviation: ND = not detected.
Different letters in the same row indicate significant differences (ANOVA, Student–Newman–Keuls test,
Mean value ± standard deviation, n = 3.
Adapted from Pérez-Navarro et al. [19].
Different families of volatiles described the varietal composition of these minority cultivars, that is, C6 compounds, terpenes, norisoprenoids, benzenic compounds, and alcohols. C6 compounds are derived from linoleic and lignoceric acids via enzymatic reactions and are responsible for herbaceous aroma in grapes and wines [20]. Among the free C6 compounds, 1-hexanol,
The bound fraction of C6 compounds was dominated by 1-hexanol, hexanal, and
Benzaldehyde, benzyl alcohol, 2-phenylethanol, phenylacetaldehyde, vanillin, and acetovanillone were the benzenic compounds found in the six grape varieties. Eugenol was only present in bound form. The concentration of each bound benzenic compound was, in general, higher than those observed in the free one, mainly benzyl alcohol and 2-phenylethanol which have been described as a floral aroma component [25]. Eugenol contributes to the spicy and smoky notes and has a low perception threshold (6 μg/L) [26], thus may have a significant impact on wine aroma. In all grapes evaluated, the concentrations of this compound exceeded its odor threshold with the highest concentration in Tinto Fragoso grapes (26.30 μg/L). In this study, red grape cultivars were characterized by a higher eugenol concentration than white ones. Several alcohols in both free and bound forms were identified in the studied grapes. However, 3-methyl-2-butanol was only detected in the free form. The six grape cultivars had greater levels of bound alcohols than the free fraction. The higher amounts of alcohol in the glycosidic form were 5 to 13 times higher than those in the free forms. These compounds derived from grapes do have not a significant impact on wine aroma due to the number of alcohols formed during alcoholic fermentation as secondary yeast metabolites [6]. The total concentration of free volatiles varied according to grape cultivar, with values between 1.96 and 2.98 mg/L. In general, total concentration of the bound fraction of volatile compounds was lower than those observed in the free forms. However, the proportion of glycosylated fraction present in all grapes may have a relevant role in the aromatic potential of these grapes, with a concentration ranging from 1.34 to 3.20 mg/L. The bound volatile compounds can be converted into free form by hydrolysis, modifying the aromatic profile of wines and enhancing the varietal character [27].
3.2 Aroma composition of wines
Wines fermented by the commercial
Compounds | Tempranillo | Moribel | Tinto Fragoso | Airén | Albillo Dorado | Montonera del Casar |
---|---|---|---|---|---|---|
1-Hexanol1 | 2.50 ± 0.02 d | 1.82 ± 0.04 c | 3.16 ± 0.09 e | 0.80 ± 0.18 b | 0.66 ± 0.03 b | 0.39 ± 0.01 a |
0.07 ± 0.01 a | 0.15 ± 0.01 b | 0.09 ± 0.01 a | 0.17 ± 0.04 b | 0.16 ± 0.00 b | 0.08 ± 0.00 a | |
0.99 ± 0.07 b | 1.25 ± 0.08 b | 1.11 ± 0.01 b | 1.86 ± 0.37 c | 1.01 ± 0.01 b | 0.05 ± 0.00 a | |
3.56 ± 0.05 c | 3.21 ± 0.13 c | 4.36 ± 0.11 d | 2.83 ± 0.59 c | 1.82 ± 0.09 b | 0.52 ± 0.01 a | |
Linalool2 | 2.16 ± 0.04 ab | 1.40 ± 0.17 a | 4.76 ± 0.54 c | 2.98 ± 0.58 b | 2.78 ± 0.20 b | 3.17 ± 0.19 b |
Citronellol2 | 2.88 ± 0.38 b | 1.60 ± 0.09 a | 4.25 ± 0.08 c | 2.11 ± 0.29 ab | 2.21 ± 0.35 ab | 2.49 ± 0.04 b |
Geraniol2 | 12.18 ± 0.84 c | 14.64 ± 0.77 d | 18.12 ± 0.43 e | 4.94 ± 0.91 a | 4.12 ± 0.60 a | 6.75 ± 0.43 b |
17.22 ± 0.42 c | 17.64 ± 0.69 c | 27.13 ± 0.89 d | 10.02 ± 1.78 ab | 9.11 ± 1.14 a | 12.41 ± 0.66 b | |
β-Damascenone2 | 4.73 ± 0.90 ab | 2.30 ± 0.65 a | 6.41 ± 0.22 ab | 3.50 ± 0.52 a | 10.57 ± 2.98 b | 7.76 ± 2.67 ab |
β-Ionone2 | 0.52 ± 0.01 b | 0.47 ± 0.05 b | 0.58 ± 0.06 b | 0.10 ± 0.02 a | 0.08 ± 0.01 a | 0.02 ± 0.00 a |
5.24 ± 0.91 ab | 2.78 ± 0.70 a | 6.99 ± 0.16 ab | 3.60 ± 0.54 ab | 10.66 ± 3.00 b | 7.78 ± 2.67 ab | |
Benzaldehyde2 | ND | ND | ND | 2.32 ± 0.79 b | 0.98 ± 0.15 a | 44.13 ± 0.31 c |
Eugenol2 | 4.17 ± 0.45 a | 13.39 ± 1.41 b | 59.24 ± 5.82 c | 2.86 ± 0.15 a | 0.93 ± 0.17 a | 0.82 ± 0.18 a |
Guaiacol2 | 10.89 ± 0.28 b | 7.38 ± 0.13 ab | 9.93 ± 4.07 b | 2.14 ± 0.47 a | 2.30 ± 0.66 a | 11.82 ± 1.43 b |
2-Phenyletanol1 | 28.62 ± 3.09 b | 20.93 ± 1.97 a | 39.69 ± 3.15 c | 17.70 ± 5.85 a | 17.86 ± 1.19 a | 11.74 ± 0.29 a |
Vanillin2 | 22.82 ± 1.51 b | 28.61 ± 5.97 b | 31.97 ± 3.82 b | 7.33 ± 1.26 a | 9.44 ± 2.51 a | 5.75 ± 0.40 a |
Acetovainillone1 | 0.09 ± 0.00 a | 0.09 ± 0.00 a | 0.44 ± 0.03 b | ND | ND | ND |
28.74 ± 3.09 b | 21.08 ± 1.97 ab | 40.24 ± 3.19 c | 17.71 ± 5.85 a | 17.87 ± 1.19 a | 11.80 ± 0.29 a | |
Methanol1 | 80.97 ± 29.53 a | 84.51 ± 5.42 a | 118.16 ± 40.50 a | 48.95 ± 1.90 a | 40.53 ± 1.24 a | 120.37 ± 3.28 a |
1-Propanol1 | 32.35 ± 5.66 a | 38.36 ± 1.99 a | 46.68 ± 11.50 a | ND | ND | ND |
Isoamyl alcohol1 | 262.11 ± 24.31 c | 242.84 ± 8.16 bc | 305.28 ± 7.17 d | 200.17 ± 0.75 ab | 231.18 ± 21.49 bc | 173.87 ± 0.71 a |
3-Ethoxy-propanol2 | 27.36 ± 10.92 a | 35.23 ± 4.76 a | 115.55 ± 70.69 a | 3.55 ± 1.63 a | 2.22 ± 1.59 a | 14.58 ± 1.90 a |
3-Methylthio-propanol1 | 0.26 ± 0.06 a | 0.23 ± 0.05 a | 0.85 ± 0.06 a | 0.25 ± 0.08 a | 0.22 ± 0.10 a | 0.25 ± 0.04 a |
Isobutanol1 | 41.13 ± 1.25 a | 36.92 ± 3.12 a | 48.34 ± 4.24 a | 34.88 ± 3.54 a | 52.19 ± 11.76 a | 50.95 ± 1.34 a |
416.84 ± 49.48 a | 402.90 ± 2.43 a | 519.41 ± 55.06 b | 284.26 ± 2.73 a | 324.12 ± 34.58 a | 345.45 ± 1.27 a | |
Isobutyric acid1 | 0.23 ± 0.01 a | 0.23 ± 0.05 a | 0.29 ± 0.16 b | ND | ND | ND |
Butyric acid1 | 0.48 ± 0.07 a | 0.50 ± 0.07 a | 0.28 ± 0.17 a | 0.49 ± 0.07 a | 0.32 ± 0.09 a | 0.58 ± 0.04 a |
Isovaleric acid1 | 1.06 ± 0.06 a | 1.20 ± 0.23 a | 1.27 ± 0.83 a | 1.08 ± 0.30 a | 0.81 ± 0.22 a | 0.91 ± 0.11 a |
Hexanoic acid1 | 12.15 ± 1.37 b | 14.01 ± 0.47 b | 5.75 ± 3.42 a | 12.29 ± 1.83 b | 10.87 ± 0.52 ab | 10.16 ± 0.39 ab |
Octanoic acid1 | 19.57 ± 2.67 ab | 26.39 ± 2.06 b | 10.42 ± 6.06 a | 48.48 ± 7.08 c | 38.65 ± 1.28 c | 43.93 ± 4.87 c |
Decanoic acid1 | 4.18 ± 0.98 a | 6.46 ± 1.15 a | 2.90 ± 1.68 a | 12.26 ± 0.35 b | 12.91 ± 0.38 b | 19.99 ± 3.71 c |
37.68 ± 5.16 ab | 48.79 ± 4.03 bc | 20.91 ± 12.32 a | 74.61 ± 8.93 c | 63.56 ± 1.47 c | 75.58 ± 9.13 c | |
Acetaldehyde1 | 10.69 ± 1.30 a | 8.72 ± 0.43 a | 11.16 ± 1.36 a | 76.06 ± 6.43 d | 41.43 ± 3.46 c | 32.17 ± 3.58 b |
10.69 ± 1.30 a | 8.72 ± 0.43 a | 11.16 ± 1.36 a | 76.06 ± 6.43 d | 41.43 ± 3.46 c | 32.17 ± 3.58 b | |
Ethyl acetate1 | 63.42 ± 12.04 a | 85.49 ± 1.91 b | 52.09 ± 3.42 a | 43.35 ± 5.13 a | 50.21 ± 5.49 a | 60.28 ± 2.18 a |
Isoamyl acetate1 | 7.17 ± 2.38 b | 10.34 ± 1.34 b | 2.13 ± 0.27 a | 7.08 ± 1.13 b | 6.97 ± 0.60 b | 6.32 ± 0.41 b |
2-Phenylethyl acetate1 | 0.28 ± 0.04 b | 0.22 ± 0.06 b | 0.13 ± 0.01 a | 0.26 ± 0.03 b | 0.26 ± 0.02 b | 0.26 ± 0.02 b |
Ethyl lactate1 | 36.35 ± 0.43 c | 24.34 ± 1.08 b | 38.87 ± 4.22 c | 5.06 ± 0.52 a | 7.41 ± 0.72 a | 6.47 ± 0.44 a |
Ethyl butyrate1 | 0.33 ± 0.03 a | 0.47 ± 0.03 b | 0.22 ± 0.01 a | 0.25 ± 0.06 a | 0.30 ± 0.04 a | 0.26 ± 0.01 a |
Ethyl hexanoate1 | 0.57 ± 0.15 a | 0.61 ± 0.01 a | 0.59 ± 0.04 a | 1.03 ± 0.12 b | 0.89 ± 0.06 ab | 0.85 ± 0.00 ab |
Ethyl octanoate1 | 0.68 ± 0.10 a | 0.66 ± 0.01 a | 0.55 ± 0.06 a | 1.47 ± 0.19 b | 1.34 ± 0.17 b | 1.39 ± 0.04 b |
Ethyl decanoate1 | 0.12 ± 0.02 a | 0.12 ± 0.00 a | 0.11 ± 0.02 a | 0.27 ± 0.01 b | 0.36 ± 0.07 c | 0.41 ± 0.01 c |
108.94 ± 15.17 bc | 122.24 ± 1.53 c | 94.68 ± 5.04 b | 58.75 ± 5.45 a | 67.73 ± 5.70 a | 76.24 ± 3.01 a | |
Furaneol2 | 12.30 ± 0.78 a | 23.54 ± 0.05 a | 14.18 ± 4.07 a | 27.34 ± 4.71 a | 15.24 ± 8.36 a | 17.40 ± 3.93 a |
12.30 ± 0.78 a | 23.54 ± 0.05 a | 14.18 ± 4.07 a | 27.34 ± 4.71 a | 15.24 ± 8.36 a | 17.40 ± 3.93 a | |
γ-Butyrolactone1 | 0.07 ± 0.01 a | 0.05 ± 0.00 a | 0.16 ± 0.04 b | 0.04 ± 0.01 a | 0.07 ± 0.02 a | 0.11 ± 0.00 a |
γ-Nonalactone2 | 10.13 ± 0.02 c | 12.85 ± 1.16 d | 23.38 ± 2.09 e | 3.29 ± 0.68 a | 7.19 ± 0.50 b | 6.29 ± 0.13 b |
δ-Dodecalactone2 | 46.11 ± 5.28 a | 45.73 ± 3.79 a | 51.46 ± 0.70 a | 108.12 ± 28.01 b | 70.54 ± 17.90 a | 63.00 ± 0.07 a |
0.13 ± 0.00 a | 0.12 ± 0.01 a | 0.24 ± 0.04 b | 149.15 ± 37.65 ab | 144.67 ± 39.34 ab | 175.37 ± 3.37 ab |
Volatile compounds affecting the secondary aroma of wine are produced
In wine, variations in the alcohol concentration can occur using different yeast strains during alcoholic fermentation [32]. Total alcohol concentrations ranged from 284 to 519 mg/L in the evaluated samples, showing greater values in red wines. Isoamyl alcohol is synthesized in the yeast cell through the Ehrlich pathway [6]. In all wines, this compound was the most abundant alcohol produced (173–305 mg/L). Most of ethyl esters of carboxylic acids are biosynthesized by the yeast metabolism during fermentation. All samples contained ethyl lactate which was produced at high levels in red wines due to the malolactic fermentation. Ethyl acetate is a compound related to fruity aromas and was the most abundant ester determined in wines, with concentration values between 50 and 85 mg/L. Other significant esters are isoamyl acetate (banana odor) and 2-phenyltehyl acetate (honey, fruity and flowery aromas).
Acetaldehyde is also produced by the metabolism of yeasts and its production is affected by SO2 content of the media [33]. Significant differences in acetaldehyde content were observed among wines, showing lower levels in red wines due to the least amount of SO2 used in red winemaking. An important group of volatile compounds in wines are carboxylic acids and their lactones which are products of the lipid metabolism of yeast and provide fatty and wax-like smell [34]. The concentrations of these compounds were under the odor threshold and did not influence final aroma of wines.
Green and grassy odors are important for the final aroma of wines and are represented by C6 compounds. In all wine samples, 1-hexanol and
The production of monoterpenes from different precursors depends on
The concentrations of 𝛽-damascenone were higher than those of 𝛽-ionone in all wines. The odor threshold of 𝛽-damascenone was exceeded, contributing to wine aroma profile [40]. Among benzenic compounds, benzaldehyde was only determined in white wines with a concentration that did not exceed the odor threshold (2000 μg/L) [38] although it could have a synergic effect on wine aroma, providing fruity notes. Tinto Fragoso wine was characterized by a volatile composition rich in eugenol. This was also observed in grapes so this compound may be considered as a varietal market for this grape cultivar. The 2-phenylethanol was determined at significantly higher concentration than the odor threshold in red wines, supporting rose odors of terpenes like geraniol. Red wines showed 2-phenylethanol concentrations similar to those reported for wines made from Bobal grapes [41].
A large number of volatile compounds were determined in the evaluated wines. However, the impact of these compounds on the overall aroma character is different. To evaluate the influence of each volatile compound on wine aroma, Odor Activity Values (OAVs) were calculated as the ratio between the concentration of the aroma compound and its odor threshold concentration (Table 4).
Compounds | Odor descriptors | Odor threshold (μg/L) | Aromatic series |
---|---|---|---|
1-Hexanol | Flower, green, cut grass | 8000 [35] | II, III |
Green | 400 [38] | III | |
Green, cut grass | 400 [35] | III | |
Linalool | Floral | 15 [35] | II |
Citronellol | Floral | 100 [35] | II |
Geraniol | Roses, geranium | 30 [35] | II |
β-Damascenone | Sweet, fruit | 0.05 [35] | I, IV |
β-Ionone | Floral, violet | 0.09 [26] | II |
Benzaldehyde | Sweet, cherry, almond | 2000 [38] | I, IV |
Eugenol | Spices, clove, honey | 6 [26] | IV, V, VII |
Guaiacol | Medicine, caramel, smoke | 10 [26] | IV, VI |
2-Phenyletanol | Floral, roses | 10,000 [35] | II |
Vanillin | Vanilla | 60 [42] | V, VII |
Acetovainillone | Vanilla, clove | 1000 [42] | VI |
Methanol | Chemical, medicinal | 668,000 [38] | VI |
1-Propanol | Ripe fruit, alcohol | 830,000 [38] | I, VI |
Isoamyl alcohol | Solvent, fusel | 30,000 [35] | VII |
3-Ethoxy-propanol | Fruity | 100 [43] | I |
3-Methylthio-propanol | Cooked, vegetable | 500 [35] | VI |
Isobutanol | Bitter, green | 40,000 [35] | III, VI |
Isobutyric acid | Rancid, butter, cheese | 2300 [26] | VI |
Butyric acid | Rancid, cheese, sweat | 173 [26] | VI |
Isovaleric acid | Acid, rancid | 33 [26] | IV, VI |
Hexanoic acid | Sweat | 420 [26] | VI |
Octanoic acid | Sweat, cheese | 500 [26] | VI |
Decanoic acid | Rancid fat | 1000 [26] | VI |
Acetaldehyde | Pungent, ripe apple | 500 [35] | I, VI |
Ethyl acetate | Fruity, solvent | 7500 [35] | I, VI |
Isoamyl acetate | Banana | 30 [26] | I |
2-Phenylethyl acetate | Floral, roses | 250 [35] | II |
Ethyl lactate | Acid, medicine | 154,636 [42] | VI |
Ethyl butyrate | Fruity | 20 [35] | I |
Ethyl hexanoate | Green apple | 14 [26] | I |
Ethyl octanoate | Sweet, fruity | 5 [26] | I, II, IV |
Ethyl decanoate | Sweet, fruity | 200 [26] | I, IV |
Furaneol | Burnt sugar, caramel, maple | 5 [44] | IV |
γ-Butyrolactone | Sweet, toast, caramel | 35,000 [40] | IV |
γ-Nonalactone | Coconut | 30 [26] | IV |
δ-Dodecalactone | Coconut | 88 [38] | IV |
Table 4.
Aromatic series, odor descriptors, and thresholds of some volatile compounds in wines.
Aromatic series: I (fruity), II (floral), III (green, fresh), IV (sweet), V (spicy), VI (fatty), and VII (other odors).
The OAVs higher than 0.1 were grouped into several aromatic series to estimate the overall wine aroma and each compound was assigned to one or several aromatic series (fruity, floral, green/fresh, sweet, spicy, fatty, and other odors) based on similar odor descriptors.
A plot of the values obtained for the aromatic series in the wines made from the six grape cultivars is shown in Figure 2, indicating the mean values of each series. The main aroma categories were series I (fruity), III (green, fresh), and VII (other odors) which contributed most to the aroma profile of wines. A great impact of odor compounds on the aromatic profile was observed in white wines, mainly in series I and VII. Tinto Fragoso was characterized by higher OAVs in the spicy aromatic series, which may be explained by its eugenol concentration.
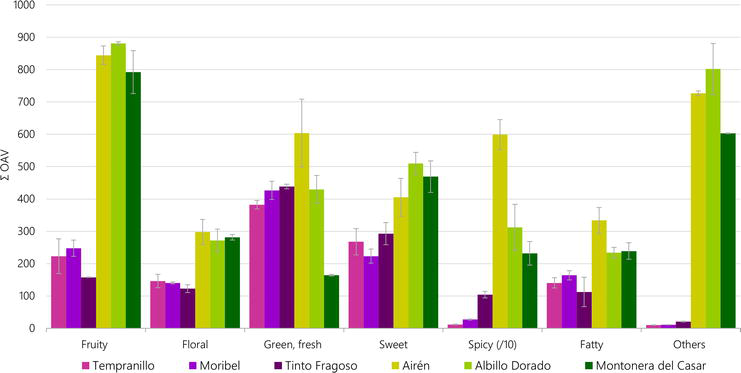
Figure 2.
Aromatic series in red and white wines (mean value and standard deviation, n = 2). Adapted from Pérez-Navarro et al. [
3.3 Sensory profiles
The Napping technique was employed to evaluate the sensory attributes identified in the produced wines from the grape cultivars studied. Figure 3A shows Napping data obtained from the sensory analysis of red wines.
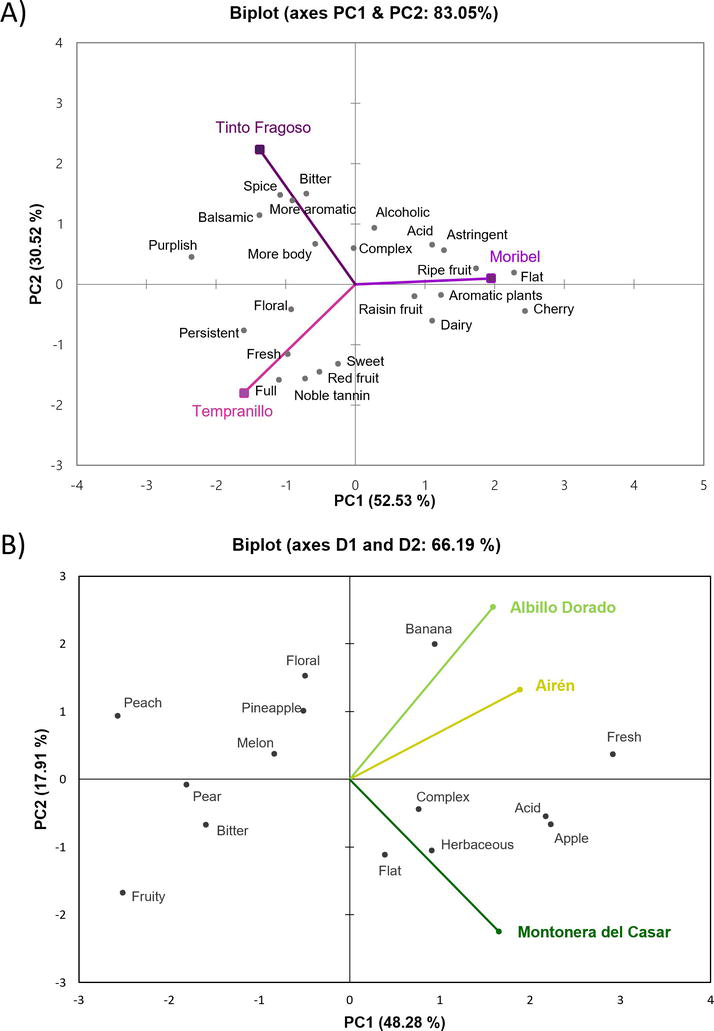
Figure 3.
Sensory characterization of red (A) and white (B) wines. Adapted from Pérez-Navarro et al. [
A clear separation between red wines was observed in the plot, which indicates that wines made from Tempranillo, Moribel, and Tinto Fragoso showed a distinctive sensory profile. Tinto Fragoso wine was characterized by its color with more intensity and purplish nuances. This wine had the most aromatic profile of evaluated red wines, providing spicy notes and persistence in mouth. Wines made from Tempranillo and Moribel grapes showed different odor descriptors. Tempranillo was characterized by red fruity odors, while forest berry and ripe fruit notes defined the aromatic profile of Moribel wine.
The sensory evaluation of white wines by a panel of experienced wine tasters is shown in Figure 3B. Airén wine was characterized by green apple odors that are well-known for this grape cultivar [39], and it was located close to Albillo Dorado wine in the plot, which had floral and fruity aromas. More herbaceous odors were determined in Montonera del Casar wine.
4. Conclusions
This chapter gathers the first comprehensive characterization of the chemical aroma composition and sensory properties of wines made from several minority grape cultivars from Castilla-La Mancha (Spain), showing the importance of a proper yeast stain for the development of the desired aroma components in wine. The strong impact exerted by the strain of
Therefore, the yeast strain selection is pivotal in grape must fermentation due to its significant impact on wine sensory properties, affecting consumer preferences. In addition, this work displayed that these minority grape varieties present an aroma profile characteristic and may be considered a viable alternative to well-known grape varieties used in wine production in this region.
Acknowledgments
This research was funded by the Castilla-La Mancha Regional Government (project POII-2014-008-P). J. P-N also thanks the European Union for financial support through the European Social Fund Plus (ESF+).
References
- 1.
Karabegović I, Malićanin M, Danilović B, Stanojević J, Stojanović SS, Nikolić N, et al. Potential of non-Saccharomyces yeast for improving the aroma and sensory profile of Prokupac red wine. Oeno One. 2021; 55 (2):181-195 - 2.
Gambetta JM, Bastian SE, Cozzolino D, Jeffery DW. Factors influencing the aroma composition of chardonnay wines. Journal of Agricultural and Food Chemistry. 2014; 62 (28):6512-6534 - 3.
Hashizume K, Samuta T. Grape maturity and light exposure affect berry methoxypyrazine concentration. American Journal of Enology and Viticulture. 1999; 50 (2):194-198 - 4.
Canosa P, Oliveira JM, Masa A, Vilanova M. Study of the volatile and glycosidically bound compounds of minority Vitis vinifera red cultivars from NW Spain. Journal of the Institute of Brewing. 2011; 117 (3):462-471 - 5.
Ferreira V, De-la-Fuente-Blanco A, Sáenz-Navajas M. Wine aroma vectors and sensory attributed. In: Reynolds AG, editor. Managing Wine Quality. 2nd ed. Cambridge, UK: Woodhead Publishing Limited; 2022. pp. 3-39 - 6.
Swiegers J, Bartowsky E, Henschke P, Pretorius I. Yeast and bacterial modulation of wine aroma and flavour. Australian Journal of Grape and Wine Research. 2005; 11 (2):139-173 - 7.
Denat M, Ferreira V, Pérez D, Sáenz-Navajas MP, Heras J. Impact of two yeast strains on Tempranillo red wine aroma profiles throughout accelerated ageing. OENO One. 2021; 55 (4):181-195 - 8.
Roca P. State of the World Vine and Wine Sector. OIV Press Conference; 2022. DOI: 10.1787/9789264244047-44-en - 9.
Wilmink J, Breuer M, Forneck A. Effects of grape phylloxera leaf infestation on grapevine growth and yield parameters in commercial vineyards: A pilot study. OENO One. 2022; 56 (1):197-208 - 10.
Robinson J, Harding J, Vouillamoz J. Wine Grapes: A Complete Guide to 1,368 Vine Varieties, Including their Origins and Flavours. Harmondsworth, UK: Allen Lane/Penguin; 2012 - 11.
Mena A, Martínez J, Fernández-González M. Recovery, identification and relationships by microsatellite analysis of ancient grapevine cultivars from Castilla-La Mancha: The largest wine growing region in the world. Genetic Resources and Crop Evolution. 2014; 61 :625-637 - 12.
Ibarz MJ, Ferreira V, Hernández-Orte P, Loscos N, Cacho J. Optimization and evaluation of a procedure for the gas chromatographic–mass spectrometric analysis of the aromas generated by fast acid hydrolysis of flavor precursors extracted from grapes. Journal of Chromatography A. 2006; 1116 (1-2):217-229 - 13.
Francis I, Newton J. Determining wine aroma from compositional data. Australian Journal of Grape and Wine Research. 2005; 11 (2):114-126 - 14.
Vilanova M, Masa A, Tardaguila J. Evaluation of the aroma descriptors variability in Spanish grape cultivars by a quantitative descriptive analysis. Euphytica. 2009; 165 :383-389 - 15.
International Organization for Standardization. ISO 8586-1:1993 - Sensory Analysis. General Guidance for the Selection, Training and Monitoring of Assessors. Part 1: Selected Assessors; 1993 - 16.
International Organization for Standardization. ISO 11035:1994 - Sensory Analysis. Identification and Selection of Descriptors for Establishing a Sensory Profile by a Multidimensional Approach; 1994 - 17.
International Organization for Standardization. ISO 8589:2007 - Sensory Analysis. General Guidance for the Design of Test Rooms; 2007 - 18.
Pagès J. Recueil direct de distances sensorielles: Application à l’évaluation de dix vins blancs du Val-de-Loire. Sciences Des Aliments. 2003; 23 (5-6):679-688 - 19.
Pérez-Navarro J. Calidad enológica de nuevos genotipos de uva identificados en el Banco de Germoplasma de Vid de Castilla-La Mancha [thesis]. Ciudad Real: University of Castilla-La Mancha; 2019 - 20.
Kalua CM, Boss PK. Evolution of volatile compounds during the development of Cabernet Sauvignon grapes ( Vitis vinifera L.). Journal of Agricultural and Food Chemistry. 2009;57 (9):3818-3830 - 21.
Qian X, Sun L, Xu X-Q , Zhu B-Q , Xu H-Y. Differential expression of VvLOXA diversifies C6 volatile profiles in some Vitis vinifera table grape cultivars. International Journal of Molecular Sciences. 2017; 18 (12):2705 - 22.
Mateo J, Jiménez M. Monoterpenes in grape juice and wines. Journal of Chromatography A. 2000; 881 (1-2):557-567 - 23.
Vilanova M, Genisheva Z, Bescansa L, Masa A, Oliveira JM. Changes in free and bound fractions of aroma compounds of four Vitis vinifera cultivars at the last ripening stages. Phytochemistry. 2012;74 :196-205 - 24.
Vilanova M, Martínez C. First study of determination of aromatic compounds of red wine from Vitis vinifera cv. Castañal grown in Galicia (NW Spain). European Food Research and Technology. 2007; 224 :431-436 - 25.
Lamikanra O, Grimm CC, Inyang ID. Formation and occurrence of flavor components in Noble muscadine wine. Food Chemistry. 1996; 56 (4):373-376 - 26.
Ferreira V, López R, Cacho JF. Quantitative determination of the odorants of young red wines from different grape varieties. Journal of the Science of Food and Agriculture. 2000; 80 (11):1659-1667 - 27.
Mendes Ferreira A, Clímaco M, Mendes FA. The role of non-Saccharomyces species in releasing glycosidic bound fraction of grape aroma components—A preliminary study. Journal of Applied Microbiology. 2001; 91 (1):67-71 - 28.
Pérez-Navarro J, Izquierdo-Canas PM, Mena-Morales A, Martinez-Gascuena J, Chacon-Vozmediano JL, Garcia-Romero E, et al. First chemical and sensory characterization of Moribel and Tinto Fragoso wines using HPLC-DAD-ESI-MS/MS, GC-MS, and napping techniques: Comparison with Tempranillo. Journal of the Science of Food and Agriculture. 2019; 99 (5):2108-2123 - 29.
Pérez-Navarro J, Izquierdo-Cañas PM, Mena-Morales A, Chacón-Vozmediano JL, Martínez-Gascueña J, García-Romero E, et al. Comprehensive chemical and sensory assessment of wines made from white grapes of Vitis vinifera cultivars Albillo Dorado and Montonera del Casar: A comparative study with Airén. Food. 2020;9 (9):1282 - 30.
Ferreira V, Fernández P, Peña C, Escudero A, Cacho JF. Investigation on the role played by fermentation esters in the aroma of young Spanish wines by multivariate analysis. Journal of the Science of Food and Agriculture. 1995; 67 (3):381-392 - 31.
Swiegers JH, Pretorius IS. Yeast modulation of wine flavor. Advances in Applied Microbiology. 2005; 57 :131-175 - 32.
Giudici P, Romano P, Zambonelli C. A biometric study of higher alcohol production in Saccharomyces cerevisiae. Canadian Journal of Microbiology. 1990; 36 (1):61-64 - 33.
Herraiz T, Martin-Alvarez PJ, Reglero G, Herraiz M, Cabezudo MD. Differences between wines fermented with and without Sulphur dioxide using various selected yeasts. Journal of the Science of Food and Agriculture. 1989; 49 (2):249-258 - 34.
Vilanova M, Oliveira JM. Application of gas chromatography on the evaluation of grape and wine aroma in Atlantic viticulture (NW Iberian Peninsula). In: Salih B, Celikbicak O, editors. Gas Chromatography in Plant Science, Wine Technology, Toxicology and Some Specific Applications. London, UK: IntechOpen; 2012. pp. 109-146 - 35.
Guth H. Identification of character impact odorants of different white wine varieties. Journal of Agricultural and Food Chemistry. 1997; 45 (8):3022-3026 - 36.
Boido E. Modificaciones producidas por la fermentación maloláctica en la fracción aromática de los vinos Tannat. Sección Enología. 2002 - 37.
Ugliano M, Bartowsky EJ, McCarthy J, Moio L, Henschke PA. Hydrolysis and transformation of grape glycosidically bound volatile compounds during fermentation with three Saccharomyces yeast strains. Journal of Agricultural and Food Chemistry. 2006; 54 (17):6322-6331 - 38.
Etievant P. Volatile Compounds in Food and Beverages: Wine. New York: Marcel Dekker; 1991 - 39.
Palomo ES, Hidalgo MD, Gonzalez-Vinas M, Perez-Coello M. Aroma enhancement in wines from different grape varieties using exogenous glycosidases. Food Chemistry. 2005; 92 (4):627-635 - 40.
Escudero A, Campo E, Fariña L, Cacho J, Ferreira V. Analytical characterization of the aroma of five premium red wines. Insights into the role of odor families and the concept of fruitiness of wines. Journal of Agricultural and Food Chemistry. 2007; 55 (11):4501-4510 - 41.
García-Carpintero EG, Sánchez-Palomo E, González-Viñas M. Aroma characterization of red wines from cv. Bobal grape variety grown in La Mancha region. Food Research International. 2011; 44 (1):61-70 - 42.
Gómez-Míguez MJ, Cacho JF, Ferreira V, Vicario IM, Heredia FJ. Volatile components of Zalema white wines. Food Chemistry. 2007; 100 (4):1464-1473 - 43.
Peinado R, Moreno J, Medina M, Mauricio J. Changes in volatile compounds and aromatic series in sherry wine with high gluconic acid levels subjected to aging by submerged flor yeast cultures. Biotechnology Letters. 2004; 26 :757-762 - 44.
Escudero A, Gogorza B, Melus M, Ortin N, Cacho J, Ferreira V. Characterization of the aroma of a wine from Maccabeo. Key role played by compounds with low odor activity values. Journal of Agricultural and Food Chemistry. 2004; 52 (11):3516-3524