Effect of UHPH on the control of wild yeast and bacteria.
Abstract
Ultra-High Pressure Homogenization (UHPH) is an emerging non-thermal technology that uses continuous pumping at 200–600 MPa (generally 300), and then the fluid is depressurized against a special valve made with highly resistant alleys and coated with diamond powder. The UHPH process is able to sterilize grape juice or whatever food fluid and at the same time is extremely efficient in inactivating oxidative enzymes. The total elimination of wild microorganisms in grape juice allows the perfect implantation of the selected Saccharomyces cerevisiae starter facilitating the expression of particular metabolic profiles or the expression of specific extracellular activities. Furthermore, the use of complex emerging biotechnologies such as the use of co-inoculations with S. cerevisiae and lactic acid bacteria to perform simultaneously alcoholic and malolactic fermentations works much better if the initial juice has a very low load of wild microorganisms. Additionally, the development of spoilage processes by wild microorganisms that can remain in the wine after fermentation is practically impossible when the initial juice is treated by UHPH. Thus, UHPH helps to obtain wines more stable from a microbiological perspective and therefore safer and more hygienic.
Keywords
- UHPH
- wine
- Saccharomyces cerevisiae
- non-Saccharomyces
- Lachancea thermotolerans
- Metschnikowia pulcherrima
- Hanseniaspora spp.
- SO2
- clean labels
- emerging biotechnologies
1. Introduction
Ultra-High Pressure Homogenization (UHPH) is an emerging non-thermal technology with many interesting advantages in food processing, including: powerful antimicrobial effect, high inactivation of oxidative enzymes, nanofragmentation, formation of stable colloidal dispersions, nanocoating, and nanoencapsulation [1, 2, 3, 4]. The liquid must be pumped at ultra-high pressure (200–600 MPa, normally 300 MPa), reaching a speed of Mach 3 in the capillary pipeline upstream of the valve and a very high intense kinetic energy that is released against the valve with intense impact forces and shear stresses. The temperature at the valve can reach 70–80°C (Figure 1) when the fluid is at room temperature or refrigerated (4–20°C), or even much higher if the fluid is preheated. Downstream of the valve, subsequent depressurization leads to cooling of the liquid that can be also enhanced by the use of heat exchangers (Figure 1).
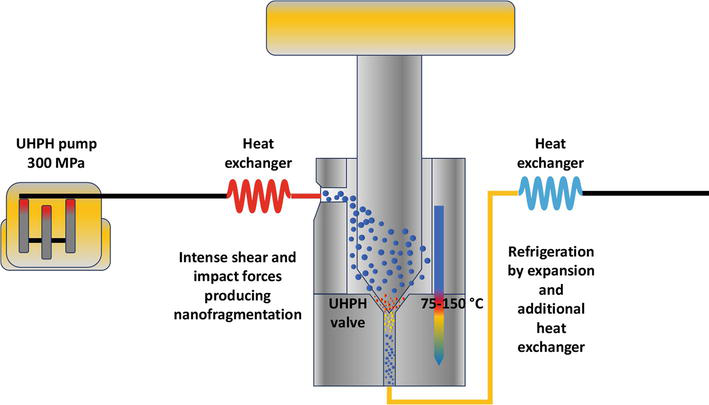
Figure 1.
Main components of the UHPH system (not at scale), including the design of the UHPH valve, the nanofragmentation process, and the temperature change of the colloidal particles.
After passing through the valve, the colloidal particles in the fluid and the microorganisms are nanofragmented, resulting in inactivation of the microorganisms. It can be observed how the initial juice contains a lot of colloidal particles from the cell walls of the pulp but also tartrate crystals and wild microbial cells (Figure 2a). From optical microscopic observations, it can be seen that after UHPH treatment, the colloidal structure is thinner in the juice, which makes it highly stable and difficult to settle, giving the appearance of a haze in the beaker and of a small granulation under optical microscopy (Figure 2b). The colloidal particle size of a pressed juice is usually in the range of a few micrometers and after UHPH processing, is reduced to nanometric scale. We have measured by AFM and laser light scattering a particle size range of 0.8–3.2 μm which is decreased by nanofragmentation after UHPH to 235–744 nm [5, 6].
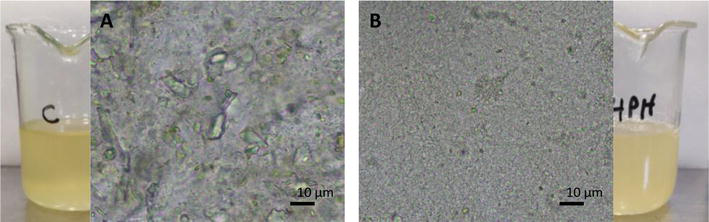
Figure 2.
Macroscopical appearance and optical microscopy of the colloids in a white grape juice
Yeast counts of 6-log cfu/mL can be easily reached within a few hours in fresh grape must after grape pressing due to the growth and development of wild microorganisms and that population can be completely eliminated with a single pass through UHPH at 300 MPa [7]. Under the same conditions, 4-log bacteria and even spores can also be inactivated depending on the in-valve temperature [5, 7].
At the same time, the UHPH process is very gentle and protective of sensitive molecules with high impact on sensory quality, such as anthocyanins [6], terpenes [5], or thiols [8]. Even when high temperatures (75–150°C) can be reached in the valve, the amount of thermal markers as HMF [5] or furosine [9] is very low or not detectable. Furthermore, the formation of the carcinogenic molecule ethyl carbamate in sake after pasteurization and its absence after UHPH processing has recently been observed [10].
2. Antimicrobial effect and implantation of Saccharomyces
UHPH is a highly effective technology to eliminate vegetative cells of yeasts and bacteria working at 300 MPa. Yeasts are particularly sensitive to the effect of UHPH and can be easily controlled (Table 1), even considering very high populations that are abnormal in healthy grapes. The same degree of inactivation can be reached with vegetative cells of aerobic bacteria.
Grape variety | UHPH conditions | Microorganisms | Initial count (CFU/mL) | Count after UHPH (CFU/mL) | Reference |
---|---|---|---|---|---|
300 ± 3 MPa, inlet temperature 20°C, in-valve 98°C (0.02s), outlet 25°C | Total yeasts | >5-log | nd | [7] | |
Non- | >5-log | nd | |||
Total aerobic bacteria | ≈4-log | nd | |||
Lactic acid bacteria | ≈4-log | nd | |||
307 ± 3 MPa, inlet temperature 23–25°C, in-valve 78–65°C (0.02 s), outlet 13–15°C | Total yeasts | 3.62-log | nd | [5] | |
Non- | 3.52-log | nd | |||
Total aerobic bacteria | 3.88-log | nd | |||
Lactic acid bacteria | 3.26-log | nd | |||
300 ± 3 MPa, inlet temperature 4°C, in-valve 78 ± 2 °C (<0.2 s), outlet 15°C | Total yeasts | ≈4-log | nd | [6] | |
Non- | 4.08-log | nd | |||
Total aerobic bacteria | 3.23-log | nd |
Table 1.
nd: not detected.
The degree of inactivation can be comparable to HTST (High Temperature Short Time) pasteurization when processed without thermal assistance at the valve, and similar to UHT (Ultra-High Temperature) sterilization, but with less thermal damage, when the temperature at the valve reaches 140–160
The initial yeast population in wine grapes normally ranges between 3 and 4-log CFU/mL depending on maturity and environmental conditions. It consists mainly of low fermentative apiculate yeasts, such as
No clear resistance to UHPH processing has been observed among the different non-
The selection of
3. Emerging fermentation biotechnologies and UHPH
Currently, several non-
Most of these non-
Additionally, most of these non-
Currently, it is also frequent to develop alcoholic (AF) and malolactic (MLF) fermentations, at the same time to reduce the total fermentation time with safer fermentations [31, 32, 33, 34, 35] and often with higher contents of ethyl and acetate esters [31]. For this particular biotechnology, it is important to avoid unsuitable wild yeast and bacteria species and to achieve a good implantation of the inoculated bacteria-yeast starter. It is usually recommended to make yeast-bacteria co-inoculations on healthy grapes with pH equal or lower than 3.5 and with a low wild population, to avoid undesired developments of wild bacteria and the increase of volatile acidity and other metabolites that can behave as off-flavors [32].
Therefore, UHPH is a powerful tool to facilitate the use of emerging biotechnologies in wine fermentation. Nowadays, the use of more complex mixtures of microorganisms with special growth conditions and delicate fermentative metabolisms makes it more essential to eliminate competitive wild species to better implant these inoculants and favor the expression of their metabolisms to improve the sensory quality of the wine [26, 36, 37, 38, 39, 40, 41, 42]. In this framework, UHPH produces sterilized or pasteurized juices in which all the competitiveness can be avoided while preserving the sensory quality of the juice [5, 6, 7, 8].
4. Control of polyphenol oxidase enzymes, antioxidant capacity, and SO2 reduction
Oxidative enzymes are very detrimental to wine quality and rapidly affect color, reducing paleness in white wines and increasing browning in whites (Figure 3), rosés, and reds. The main oxidative enzymes in white wines are polyphenol oxidase enzymes (PPOs), among which the most important are tyrosinase in healthy grapes, and laccase in grapes affected by
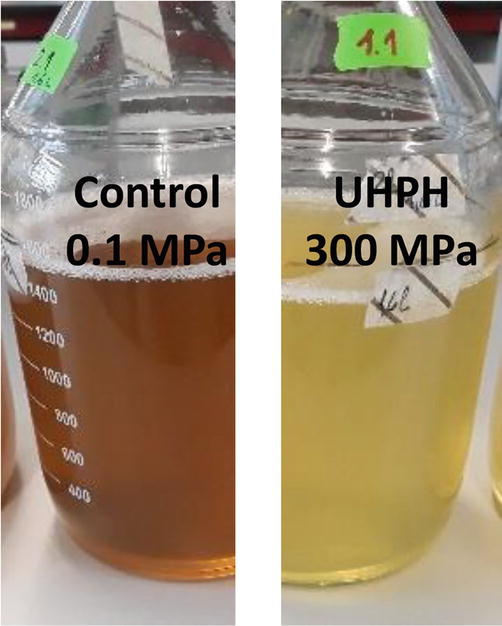
Figure 3.
Effect on color of PPOs after a few hours of air exposition in the absence of antioxidants in a control (left) and in a juice processed by UHPH (right) at 300 MPa.
The protection of juice and wine from oxidation by PPOs is done by adding antioxidants such as SO2, or ascorbic acid. SO2 is highly effective, but currently under discussion due to its toxicity for the general public, but especially for sensitive people. SO2 has a double role: anti-oxidase by inhibiting the effect of PPOs and antioxidant by protecting
UHPH causes the fragmentation or unfold and denaturation of several enzymes by impact and shear efforts, including oxidative PPOs. In white varieties fermented in the absence of SO2, it can be observed how musts processed by UHPH remain pale and without browning even under high aeration conditions and with sensitive varieties, such as Muscat (Figure 3). We have also observed that oxidation continues after fermentation and if we clarify the wines with fining agents, the wines obtained from the non-processed juices continue to form brown compounds, but this does not occur with wines obtained from musts treated by UHPH. The effects on PPO inactivation, antioxidant activity, and anthocyanin contents are summarized (Table 2) for 3 grape varieties (2 whites and 1 red). UHPH control of PPO is very useful when it is desired to apply emerging biotechnologies, such as the use of non-
Grape variety | UHPH conditions | Inactivation of PPOs and browning | Antioxidant activity | Anthocyanins | Reference |
---|---|---|---|---|---|
300 ± 3 MPa, inlet temperature 20°C, in-valve 98°C (0.02 s), outlet 25°C | Strong inactivation (>90%) Paler juices (10%) | +45% | White variety | [7] | |
307 ± 3 MPa, inlet temperature 23–25°C, in-valve 78–65°C (0.02 s), outlet 13–15°C | Strong inactivation (>90%) Paler juices that remain stable more than 3 days in the absence of SO2 | +56% | White variety | [5] | |
300 ± 3 MPa, inlet temperature 4°C, in-valve 78 ± 2°C (<0.2 s), outlet 15°C | Strong inactivation Lower browning of anthocyanins | +6% | Protection of anthocyanins especially acylated forms (+9%) | [6] |
Table 2.
Effect of UHPH on the control of PPOs and oxidation.
In addition to the improved sensory quality and stability of the juices processed by UHPH, higher antioxidant activity was observed, probably due to the inactivation of oxidative enzymes (Table 2). These effects made the UHPH juices healthier and with better nutraceutical properties. These characteristics are maintained in the subsequent wines after fermentation. The inactivation of enzymes by UHPH probably by unfold and denaturation could also have a positive effect on protein haze in white wines [5]. Therefore, UHPH musts can have fewer proteins able to produce turbidity or even the unfolding produced by the UHPH can facilitate the removal of unstable proteins by the use of proteases without the need for thermal pre-treatments.
5. Conclusion
UHPH is a powerful emerging non-thermal technology able to eliminate wild microorganisms facilitating the use of new biotechnologies with non-competitive non-
Acknowledgments
This research was funded by MINISTERIO DE CIENCIA E INNOVACIÓN, project ENOINNOVAPRESS – PID2021-124250OB-I00 Ministerio de Ciencia e Innovación. This work was partially supported by the State Program to Promote Scientific-Technical Research and its Transfer of the Spanish Ministry of Science and Innovation through the MALTA CONSOLIDER TEAM Research Network (RED2022-134388-T).
Appendices and nomenclature
Atomic Force Microscopy | |
Colony Forming Units | |
glutathione | |
hydroxy methyl furfural | |
malolactic fermentation | |
mega pascal | |
poly phenol oxidases | |
Ultra-High Pressure Homogenization |
References
- 1.
Zamora A, Guamis B. Opportunities for ultra-high-pressure homogenization (UHPH) for the food industry. Food Engineering Reviews. 2015; 7 :130-142. DOI: 10.1007/s12393-014-9097-4 - 2.
Patrignani F, Lanciotti R. Applications of high and ultra high pressure homogenization for food safety. Frontiers in Microbiology. 2016; 7 :1132. DOI: 10.3389/fmicb.2016.01132 - 3.
Morata A, Guamis B. Use of UHPH to obtain juices with better nutritional quality and healthier wines with low levels of SO2. Frontiers in Nutrition. 2020; 7 :598286. DOI: 10.3389/fnut.2020.598286 - 4.
Morata A, Loira I, Guamis B, Raso J, et al. Emerging technologies to increase extraction, control microorganisms, and reduce SO2. In: Cosme F, Nunes FM, Filipe-Ribeiro L, editors. Chemistry and Biochemistry of Winemaking, Wine Stabilization and Aging. London, UK: IntechOpen; 2021. DOI: 10.5772/intechopen.92035 - 5.
Bañuelos MA, Loira I, Guamis B, Escott C, Del Fresno JM, Codina-Torrella I, et al. White wine processing by UHPH without SO2. Elimination of microbial populations and effect in oxidative enzymes, colloidal stability and sensory quality. Food Chemistry. 2020; 332 :127417. DOI: 10.1016/j.foodchem.2020.127417 - 6.
Vaquero C, Escott C, Loira I, Guamis B del Fresno JM, et al. Cabernet sauvignon red must processing by UHPH to produce wine without SO2: The colloidal structure, microbial and oxidation control, colour protection and sensory quality of the wine. Food and Bioprocess Technology. 2022; 15 :620-634. DOI: 10.1007/s11947-022-02766-8 - 7.
Loira I, Morata A, Bañuelos MA, Puig-Pujol A, Guamis B, González C, et al. Use of ultra-high pressure homogenization processing in winemaking: Control of microbial populations in grape musts and effects in sensory quality. Innovative Food Science and Emerging Technologies. 2018; 50 :50-56. DOI: 10.1016/j.ifset.2018.10.005 - 8.
Morata A, Escott C, Vaquero C, del Fresno JM, Guamis B, Loira I, et al. Improving the implantation of non- Saccharomyces yeasts in winemaking by UHPH processing. Oral communication. In: Proceedings of the 44th World Congress of Vine and Wine; 5-9 June 2023; Cadiz-Jerez, Spain. BIO Web of Conferences. Vol. 68. Dijon, France: OIV; 2023. p. 02001 - 9.
Ferragut V, Hernández-Herrero M, Veciana-Nogués MT, Borras-Suarez M, González-Linares J, Vidal-Carou MC, et al. Ultra-high-pressure homogenization (UHPH) system for producing high-quality vegetable-based beverages: Physicochemical, microbiological, nutritional and toxicological characteristics. Journal of the Science of Food and Agriculture. 2015; 95 :953-961. DOI: 10.1002/jsfa.6769 - 10.
Arakawa G, Yokoi K. Application of multiple ultra-high-pressure homogenization to the pasteurization process of Japanese rice wine, sake. Journal of Bioscience and Bioengineering. 2023; 136 :117-122. DOI: 10.1016/j.jbiosc.2023.05.003 - 11.
Cocolin L, Bisson LF, Mills DA. Direct profiling of the yeast dynamics in wine fermentations. FEMS Microbiology Letters. 2000; 189 (1):81-87. DOI: 10.1111/j.1574-6968.2000.tb09210.x - 12.
Mills DA, Johannsen EA, Cocolin L. Yeast diversity and persistence in Botrytis-affected wine fermentations. Applied and Environmental Microbiology. 2002; 68 (10):4884-4893. DOI: 10.1128/AEM.68.10.4884-4893.2002 - 13.
Fleet GH. Wine yeasts for the future. FEMS Yeast Research. Nov 2008; 8 (7):979-995. DOI: 10.1111/j.1567-1364.2008.00427.x - 14.
Anagnostopoulos D, Kamilari E, Tsaltas D. Contribution of the microbiome as a tool for estimating Wine’s fermentation output and authentication. In: Morata A, Loira I, editors. Advances in Grape and Wine Biotechnology. London, UK: IntechOpen; 2019. DOI: 10.5772/intechopen.85692 - 15.
Loira I, Escott C, del Fresno JM, Bañuelos MA, González C, Guamis B, et al. Chapter 5 – White must preservation by ultra-high pressure homogenization without SO2. In: Morata A, editor. White Wine Technology. London, UK: Academic Press; 2022. pp. 49-59. DOI: 10.1016/B978-0-12-823497-6.00014-4 - 16.
Pretorius IS. Tailoring wine yeast for the new millennium: Novel approaches to the ancient art of winemaking. Yeast. 2000; 16 :675-729. DOI: 10.1002/1097-0061(20000615)16:8<675::AID-YEA585>3.0.CO;2-B - 17.
Suárez-Lepe JA, Morata A. New trends in yeast selection for winemaking. Trends in Food Science & Technology. 2012; 23 :39-50. DOI: 10.1016/j.tifs.2011.08.005 - 18.
Loira I, Morata A, Bañuelos MA, Suárez-Lepe JA. Chapter 15 – Isolation, selection, and identification techniques for non- Saccharomyces yeasts of oenological interest. In: Grumezescu AM, Holban AM, editors. Biotechnological Progress and Beverage Consumption. London, UK: Academic Press; 2020. pp. 467-508. DOI: 10.1016/B978-0-12-816678-9.00015-1 - 19.
Morata A, Loira I, Tesfaye W, Bañuelos MA, González C, Suárez Lepe JA. Lachancea thermotolerans applications in wine technology. Fermentation. 2018;4 :53. DOI: 10.3390/fermentation4030053 - 20.
Loira I, Morata A, Palomero F, González C, Suárez-Lepe JA. Schizosaccharomyces pombe : A promising biotechnology for modulating wine composition. Fermentation. 2018;4 :70. DOI: 10.3390/fermentation4030070 - 21.
Portaro L, Maioli F, Canuti V, Picchi M, Lencioni L, Mannazzu I, et al. Schizosaccharomyces japonicus /Saccharomyces cerevisiae mixed starter cultures: New perspectives for the improvement of Sangiovese aroma, taste, and color stability. LWT. 2022;156 :113009. DOI: 10.1016/j.lwt.2021.113009 - 22.
Ramírez M, Velázquez R. The yeast Torulaspora delbrueckii : An interesting but difficult-to-use tool for winemaking. Fermentation. 2018;4 :94. DOI: 10.3390/fermentation4040094 - 23.
Martin V, Valera MJ, Medina K, Boido E, Carrau F. Oenological impact of the Hanseniaspora /Kloeckera yeast genus on wines—A review. Fermentation. 2018;4 :76. DOI: 10.3390/fermentation4030076 - 24.
Morata A, Loira I, Escott C, del Fresno JM, Bañuelos MA, Suárez-Lepe JA. Applications of Metschnikowia pulcherrima in wine biotechnology. Fermentation. 2019;5 :63. DOI: 10.3390/fermentation5030063 - 25.
Morata A, Escott C, Bañuelos MA, Loira I, del Fresno JM, González C, et al. Contribution of non- Saccharomyces yeasts to wine freshness: A review. Biomolecules. 2020;10 :34. DOI: 10.3390/biom10010034 - 26.
Escott C, Vaquero C, Loira I, López C, González C, Morata A. Synergetic effect of Metschnikowia pulcherrima andLachancea thermotolerans in acidification and aroma compounds in airén wines. Food. 2022;11 :3734. DOI: 10.3390/foods11223734 - 27.
Jolly NP, Varela C, Pretorius IS. Not your ordinary yeast: Non- Saccharomyces yeasts in wine production uncovered. FEMS Yeast Research. 2014;14 :215-237. DOI: 10.1111/1567-1364.12111 - 28.
Gobbi M, Comitini F, Domizio P, Romani C, Lencioni L, Mannazzu I, et al. Lachancea thermotolerans andSaccharomyces cerevisiae in simultaneous and sequential co-fermentation: A strategy to enhance acidity and improve the overall quality of wine. Food Microbiology. 2013;33 :271-281. DOI: 10.1016/j.fm.2012.10.004 - 29.
Ciani M, Comitini F, Mannazzu I, Domizio P. Controlled mixed culture fermentation: A new perspective on the use of non- Saccharomyces yeasts in winemaking. FEMS Yeast Research. 2010;10 :123-133. DOI: 10.1111/j.1567-1364.2009.00579.x - 30.
Comitini F, Gobbi M, Domizio P, Romani C, Lencioni L, Mannazzu I, et al. Selected non- Saccharomyces wine yeasts in controlled multistarter fermentations withSaccharomyces cerevisiae . Food Microbiology. 2011;28 :873-882. DOI: 10.1016/j.fm.2010.12.001 - 31.
Knoll C, Fritsch S, Schnell S, Grossmann M, Krieger-Weber S, du Toit M, et al. Impact of different malolactic fermentation inoculation scenarios on Riesling wine aroma. World Journal of Microbiology and Biotechnology. 2012; 28 :1143-1153. DOI: 10.1007/s11274-011-0917-x - 32.
Pardo I, Ferrer S. Chapter 7 - Yeast-bacteria coinoculation. In: Morata A, editor. Red Wine Technology. London, UK: Academic Press; 2019. pp. 99-114. DOI: 10.1016/B978-0-12-814399-5.00007-4 - 33.
Krieger-Weber S. Application of yeast and bacteria as starter cultures. In: König H, Unden G, Fröhlich J, editors. Biology of Microorganisms on Grapes, in Must and in Wine. 2nd ed. Cham: Springer International Publishing; 2017. pp. 605-634. DOI: 10.1007/978-3-319-60021-5_25 - 34.
Bartowsky EJ, Costello PJ, Chambers PJ. Emerging trends in the application of malolactic fermentation. Australian Journal of Grape and Wine Research. 2015; 21 :663-669. DOI: 10.1111/ajgw.12185 - 35.
Krieger-Weber S, Heras JM, Suarez C. Lactobacillus plantarum , a new biological tool to control malolactic fermentation: A review and an outlook. Beverages. 2020;6 :23. DOI: 10.3390/beverages6020023 - 36.
Vaquero C, Loira I, Heras JM, Carrau F, González C, Morata A. Biocompatibility in ternary fermentations with Lachancea thermotolerans , other non-Saccharomyces andSaccharomyces cerevisiae to control pH and improve the sensory profile of wines from warm areas. Frontiers in Microbiology. 2021;12 :656262. DOI: 10.3389/fmicb.2021.656262 - 37.
Morata A, Arroyo T, Bañuelos MA, Blanco P, Briones A, Cantoral JM, et al. Wine yeast selection in the Iberian Peninsula: Saccharomyces and non-Saccharomyces as drivers of innovation in Spanish and Portuguese wine industries. Critical Reviews in Food Science and Nutrition. 2022;10 :1-29. DOI: 10.1080/10408398.2022.2083574 - 38.
Vaquero C, Escott C, Heras JM, Carrau F, Morata A. Co-inoculations of Lachancea thermotolerans with differentHanseniaspora spp.: Acidification, aroma, biocompatibility, and effects of nutrients in wine. Food Research International. 2022;161 :111891. DOI: 10.1016/j.foodres.2022.111891 - 39.
Del Fresno JM, Escott C, Loira I, Herbert-Pucheta JE, Schneider R, Carrau F, et al. Impact of Hanseniaspora vineae in alcoholic fermentation and ageing on lees of high-quality white wine. Fermentation. 2020;6 :66. DOI: 10.3390/fermentation6030066 - 40.
Vaquero C, Izquierdo-Cañas PM, Mena-Morales A, Marchante-Cuevas L, Heras JM, Morata A. Use of Lachancea thermotolerans for biological vs. chemical acidification at pilot-scale in white wines from warm areas. Fermentation. 2021;7 :193. DOI: 10.3390/fermentation7030193 - 41.
Del Fresno JM, Escott C, Loira I, Carrau F, Cuerda R, Schneider R, et al. The impact of Hanseniaspora vineae fermentation and ageing on lees on the terpenic aromatic profile of white wines of the Albillo variety. International Journal of Molecular Sciences. 2021;22 :2195. DOI: 10.3390/ijms22042195 - 42.
Vaquero C, Loira I, Bañuelos MA, Heras JM, Cuerda R, Morata A. Industrial performance of several Lachancea thermotolerans strains for pH control in white wines from warm areas. Microorganisms. 2020;8 :830. DOI: 10.3390/microorganisms8060830 - 43.
Martinez MV, Whitaker JR. The biochemistry and control of enzymatic browning. Trends in Food Science & Technology. 1995; 6 :195-200. DOI: 10.1016/S0924-2244(00)89054-8 - 44.
Giacosa S, Río Segade S, Cagnasso E, Caudana A, Rolle L, Gerbi V. Chapter 21 – SO2 in wines: Rational use and possible alternatives. In: Morata A, editor. Red Wine Technology. London, UK: Academic Press; 2019. pp. 309-321. DOI: 10.1016/B978-0-12-814399-5.00021-9 - 45.
Martínez J, González-Arenzana L. Chapter 3 – Use of glutathione in the winemaking of white grape varieties. In: Morata A, editor. White Wine Technology. London, UK: Academic Press; 2022. pp. 29-38. DOI: 10.1016/B978-0-12-823497-6.00021-1 - 46.
Kilmartin P. Chapter 23 - Impacts of phenolics and prefermentation antioxidant additions on wine aroma. In: Morata A, editor. White Wine Technology. London, UK: Academic Press; 2022. pp. 301-312. DOI: 10.1016/B978-0-12-823497-6.00029-6