Genetic modifications and adaptations of viral Gag-CA that render HIV-1 macaque-tropic.
Abstract
Human immunodeficiency virus type 1 (HIV-1) is tropic for humans and replicates in virtually none of the other animal species. While various animal models to mimic the conflict between HIV-1 and human hosts have been proposed, nonhuman primates (NHPs) are thought to be most suitable from a purely scientific point of view for the HIV-1/AIDS model studies. Because NHPs are resistant to HIV-1, remodeling the HIV-1 genome is required to validate the productive infection of NHPs. Two types have been reported as retrofitted viruses, that is, SHIVs and HIV-1 derivatives. SHIVs are SIVs (simian immunodeficiency viruses) that carry a small portion of the HIV-1 genome, whereas HIV-1 derivatives are HIV-1 with a minimal sequence/genome modification. SHIVs have been successfully used for studies specifically targeting HIV-1 Pol-RT (reverse transcriptase) and Env proteins. HIV-1 derivatives can induce AIDS in NHPs under certain conditions. More importantly, HIV-1 derivatives contribute to elucidating the HIV-1 adaptation and virus-host interaction through analyzing the process of acquiring replication capacity and pathogenicity in restrictive hosts distinct from natural hosts. In this chapter, we summarize NHP model studies on HIV-1/AIDS using SIV, SHIV, or HIV-1 derivatives and discuss the significance of HIV-1 derivatives toward understanding the HIV-1 biology.
Keywords
- HIV-1
- macaque-tropic HIV-1
- SIV
- SHIV
- animal model
- adaptation
- replication
- pathogenesis
1. Introduction
HIV-1 belongs to a subfamily
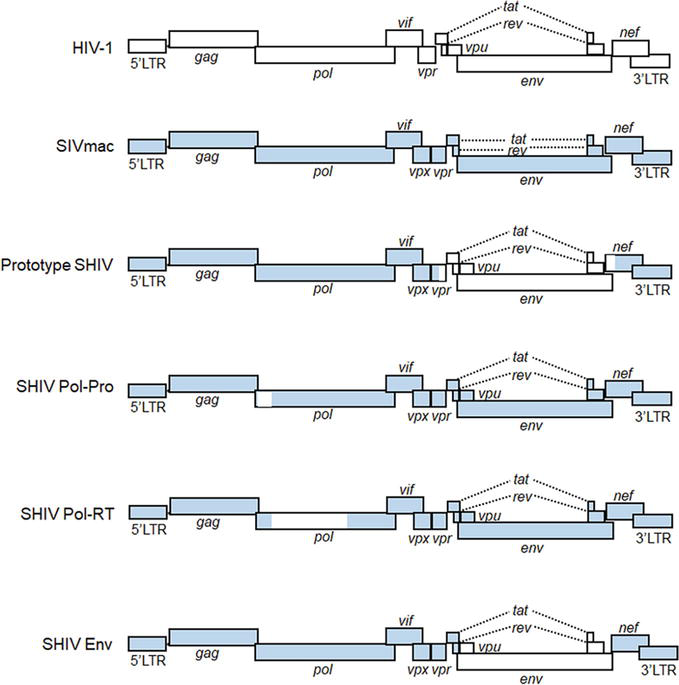
Figure 1.
Schematic genome representation of HIV-1, SIVmac, and various SHIVs. White and blue areas indicate sequences from HIV-1 and SIVmac, respectively. Pol-Pro, pol-protease; Pol-RT, pol-reverse transcriptase; LTR, long terminal repeat.
One of the important biological features of HIV-1 is to specifically adapt itself to replication in humans, exhibiting a narrow host range virtually only to humans. Thus, no appropriate experimental animals that are susceptible to authentic HIV-1 infection and pathogenesis are currently available. Unadulterated HIV-1 clones can establish spreading infection in humanized mice (mice reconstituted with human hematopoietic cells), although they are not pathogenic to humanized mice. HIV-1-infected humanized mice are useful for basic and clinical studies such as those on determination of the
2. The emergence of HIV-1 and its narrow host range
HIV-1 exhibits a narrow host range and cannot replicate in macaque cells as described above. The excellent study using SHIV clones first demonstrated that HIV-1 replication in macaque cells is blocked at the post-entry step [19]. Results obtained in the study indicated that Gag-Pol and Vif derived from SIVmac are most likely to be necessary for viral replication in macaque cells [19]. Several years later, intrinsic restriction factors that potently inhibit HIV-1 replication in macaque cells (APOBEC3 protein family, TRIM5 protein family, and tetherin) were identified one after another [20, 21, 22, 23]. APOBEC3 proteins have cytidine deaminase activity, and its major function is to introduce lethal mutations into the HIV-1 genome. TRIM5 proteins target incoming viral cores and perturb the reverse transcription step of HIV-1 replication. Tetherin suppresses virus budding by tethering progeny virions at the plasma membrane as its name suggests. HIV-1 Vif, Gag-CA, and Vpu can antagonize APOBEC3 proteins, TRIM5 proteins, and tetherin in human cells, respectively, but not at all in macaque cells. The actions of these intrinsic restriction factors explain really well why HIV-1 cannot replicate in macaque cells.
While HIV-1 replication is completely blocked in macaques, HIV-1 has been shown to have emerged through the repeated cross-species transmissions of various SIVs from their natural hosts to the new hosts and the viral recombination/adaptation in transmitted hosts (Figure 2) [20, 24, 25]. In brief, SIVmon/mus/gsn, which parasitize Mona monkey, Mustached monkey, and Greater spot-nosed monkey as natural hosts, respectively, and SIVrcm from Red-capped mangabey co-infected chimpanzees by cross-species transmissions. SIVcpz arose by their recombination in chimpanzees and resulted in the elimination of
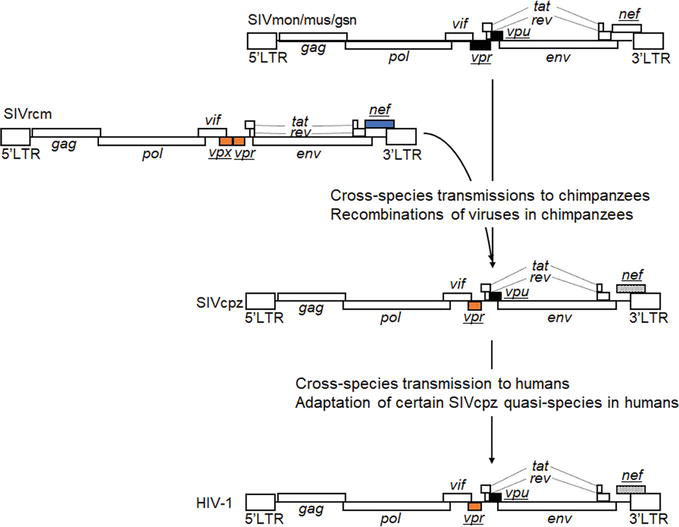
Figure 2.
The emergence of HIV-1 from ancestral SIVs. HIV-1 emergence has been suggested to have resulted from cross-species transmissions of SIVs and their recombinations in transmitted hosts [
3. SIV/SHIV clones and nonhuman primate models
Taking the strict human tropism of HIV-1 into consideration, researchers have made every effort to generate appropriate model study systems for the HIV-1/AIDS research by selecting viruses, selecting host animals, genetically altering viruses, and/or genetically altering host animals [10, 11, 12, 13, 14, 15, 16, 17, 18]. A wide variety of animal species from mice to primates and of lentivirus species have been proposed for the surrogate models and extensively tested for their usability and for their scientific value. Among these, NHP models using primate immunodeficiency viruses (SIVmac and SHIV) (Figure 1) are most suitable for the HIV-1/AIDS research from various points of view, such as pathogenic outcome and robust immune responses [13, 17, 28]. Due to the close similarity in genetic background and physiological status, researchers have plenty and successful experience in the NHP system to carry out various basic research on viruses and/or to do translational research to develop drugs, vaccines, and other interventions [10, 11, 12, 13, 14, 15, 16, 17, 18]. Of the three macaque species (rhesus, cynomolgus, and pigtailed macaques) frequently used for experimental virus infections, the pigtailed and rhesus macaques are known to be most susceptible and resistant to the SIV infection, respectively. Pigtailed macaques genetically lack the restriction of TRIM5alpha against viruses
To construct a novel class of infectious molecular clones carrying the HIV-1 genes that replicate in macaque cells, two different approaches have been taken. One is the SHIV (Figure 1), that is, a virus clone constructed in the background of SIVmac genome, and another is the HIV-1 derivative, that is, a variant HIV-1 clone with a minimal sequence modification in the context of HIV-1 genome [29, 30, 31, 32, 33, 34, 35, 36, 37, 38, 39, 40, 41, 42, 43, 44]. While the former virus clones commonly have been designated SHIV [31], the latter clones have been variously called as HIV-1 derivative [34], stHIV-1 [33], HIV-1mt [39], HSIV [40], or HIV-1rmt [43]. Research on the SHIV preceded study on the HIV-1 derivative. As for SHIVs, it is reasonable to construct clones carrying the HIV-1 genes/domain sequences that are not determinants for its species tropism. Even if the chimeric constructs mainly consist of SIVmac sequences/genes, the activity and/or function of the inserted HIV-1 genes in the replication cycle can be readily assessed individually or combinedly
4. HIV-1 derivative clones
It has been well-recognized and generalized that the restriction of viral replication occurs at the entry step on the cell surface and/or at some intracellular stage(s) [84]. As clarified by our early studies, the HIV-1 species tropism is determined at the early post-entry phase, within the viral DNA synthesis event [19, 29]. The viral determinants for the tropism were predicted to be Gag-CA and Vif as described above. To generate a novel class of HIV-1 that can replicate in macaque cells, it was necessary to genetically modify the two viral genomic regions. We first checked whether a small portion of
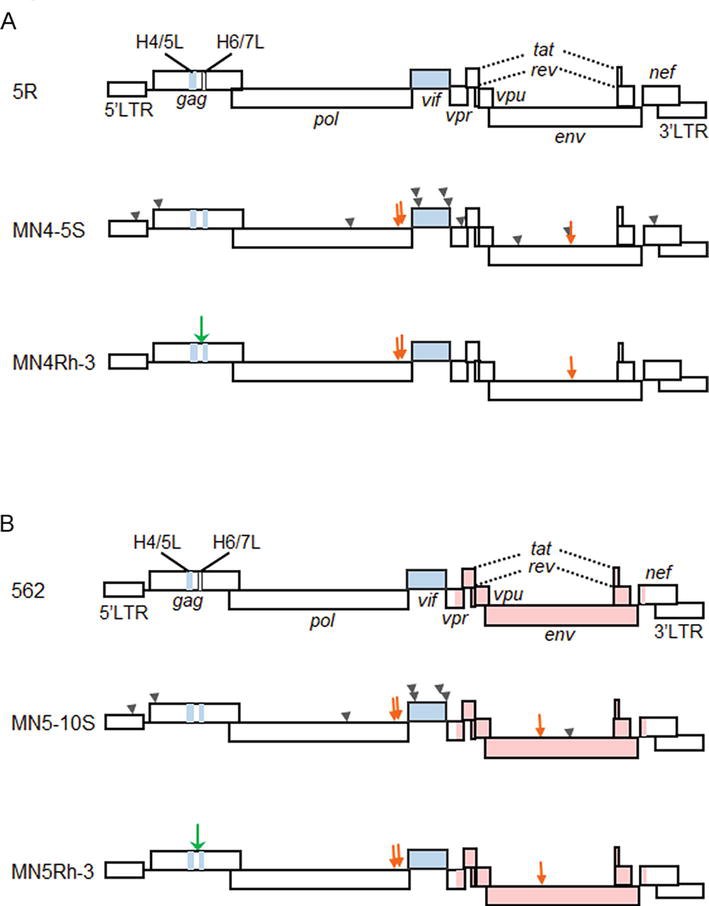
Figure 3.
Schematic representation of various HIV-1mt genomes. CXCR4-tropic and CCR5-tropic clones are shown in panels (A) and (B), respectively. Green and orange arrows indicate the structure guided and spontaneously occurred mutations with growth-enhancing effects, respectively [
In order to improve the replication ability in macaque cells of NL-DT5R, and also to construct CCR5-tropic clones, we altered virus genomes in a stepwise manner by the sequence/structure-guided mutagenesis and by virus adaptation in cells (Figure 3). NL-DT5R was found to be rather sensitive, if not completely, to cellular restriction factors TRIM5 and APOBEC3 proteins [91]. First, we replaced the loop domain of HIV-1 Gag-CA between helices 6 and 7 with the corresponding site of SIVmac (MN4-5S in Figure 3A) [39, 92] to increase resistance to TRIM5, and then randomly modify the viral genome by the adaptation in macaque cells and structure-guided mutagenesis (MN4-Rh-3 in Figure 3A) [42, 87]. CCR5-tropic MN5Rh-3 that carries the
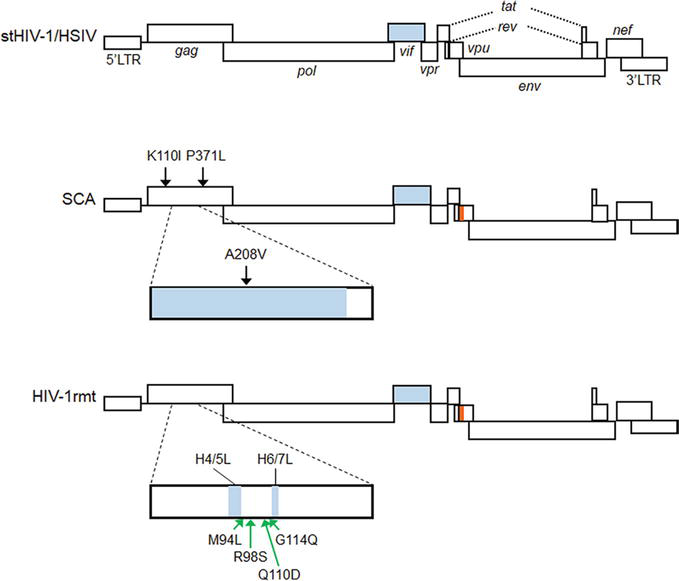
Figure 4.
Schematic representation of HIV-1rmt genomes. For comparison, the genomes of stHIV-1 (pig-tailed macaque version) [
Finally, the growth potential of CXCR4-tropic and CCR5-tropic HIV-1rmt clones was examined in rhesus macaques [44]. Both clones grew comparably well in rhesus PBMCs. When inoculated into rhesus macaques, the two virus clones certainly initiated productive infection (peak viremia ~105 copies/mL and ~ 104 copies/mL for CXCR4- and CCR5-tropic viruses, respectively). However, virus production gradually became undetectable for all the animals tested (5–6- and 3–4-weeks post-infection for CXCR4- and CCR5-tropic viruses, respectively), indicating that the virus replication level at the initial phase was insufficient for viral persistence [44]. The peak level of virus production was significantly low relative to that by SIVmac. On one hand, Hatziioannou et al. have reported that they successfully generated a pathogenic molecular clone (stHIV-A19) upon infection to CD8+ cell-depleted pigtailed macaques [93, 94]. The molecular clone stHIV-A19 was obtained from a pathogenic viral swarm by repeated animal-to-animal (CD8+ cell-depleted pigtailed macaque) virus passage [94]. The virus level in the animal is maintained to be high only with the depletion treatment, being consistent with the appearance of AIDS. Clearly, this is the first study to describe the HIV-1 derivative molecular clone that can cause AIDS in animals.
5. Adaptation of macaque-tropic HIV-1 derivatives to macaque cells/individuals
Macaque-tropic HIV-1 derivatives are useful not only for the establishment of HIV-1-macaque infection models but also serve as model systems to investigate the inherent HIV-1 property, the high mutation/adaptation abilities. It is because one can analyze how HIV-1 adapts to replication-restrictive environments imposed by macaque cells. Studies on the adaptation process, for example, what mutations emerged in the genome of macaque-tropic HIV-1 derivatives in macaques as a new host and how the mutations altered the activity/function of viral proteins, would provide pivotal insights into determinants related to HIV-1 replication and pathogenesis. As described earlier, evading the potent restriction from intrinsic factors such as APOBEC3 and TRIM5 proteins in macaque cells was essential for the generation of macaque-tropic HIV-1 clones. The restriction from APOBEC3 proteins can be overcome by the replacement of the entire
Clones | Gag-CA | Genetic modifications | Adaptive mutations | References |
---|---|---|---|---|
stHIV-1(SCA) (Figure 4) | SCA | Gag containing SIVmac239 Gag-CA (1 to 204 amino acids) | Gag (K110I, A208V, and P371L) | [33] |
HIV-1NL4–3/HIV-1HXB2/GFP (NHG) | LNEIEa | Gag-CA (M10L, I91N, A92E, M96I, and G116E) | [97] | |
HIV-1rmt (Figure 4) | LSDQ | Gag-CA containing a loop between helices 4 and 5/a loop between helices 6 and 7 loop from SIVmac239, and amino acid substitutions (M94L, R98S, and G114Q) | Structure-guided Gag-CA mutation (Q110D) based on an adaptive mutation (Gag-CA G114E) | [43, 87, 96] |
Adaptation experiments of our prototype macaque-tropic HIV-1 derivatives NL-DT5R and NL-DT562 in macaque cells led to the identification of a novel genomic region that can determine the Vif expression level. Initially, we found replication-enhancing adaptive mutations within integrase (Pol-IN) C-terminal domain (CTD) that frequently and reproducibly emerged during independent adaptation experiments (Table 2) [88, 104, 105]. Extensive virological and sequence analyses of these adaptive mutations showed: 1) viral growth potential can be altered by naturally occurring synonymous single-nucleotide mutations (nsSNMs) within the region surrounding identified adaptive mutations, 2) these identified nsSNMs result in variations in Vif expression levels, and thus, 3) variations in viral replication by the nsSNMs occur dependently on both Vif and APOBEC3G expression levels. Moreover, we found that changes in Vif expression levels were due to the effect of these nsSNMs on the splicing efficiency at the splicing acceptor 1 (SA1) within
Clones | Cells or animals used for adaptation | Adaptive mutations | Effects | References | |
---|---|---|---|---|---|
Regions | Mutations | ||||
NL-DT5R (Figure 3) | Cynomolgus HSC-F cells | Pol-IN | V234I | Optimization of vial mRNA production through modification of splicing efficiency at splicing acceptor 1 | [88, 104, 105] |
Env-gp120 (C4) | E427K | Enhancement of CD4 binding ability | [88, 106] | ||
Rhesus HSR5.4S1 cells | Pol-IN | F223Y | Same as the Pol-IN V234I mutation | [88, 104, 105] | |
NL-DT562 (Figure 3) | Cynomolgus HSC-F cells | Pol-IN | N222K | Same as the Pol-IN V234I mutation | [88, 104, 105] |
Env-gp120 (V3)a | S304G | Increase in the interaction with CCR5 | [88, 107] | ||
Rhesus HSR5.4S1 cells | Pol-IN | N222K | Same as the Pol-IN V234I mutation | [88, 104, 105] | |
Env-gp120 (V3)b | S304G | See the identical mutation above | [88, 107] | ||
G310R | Increase in the species-specific interaction with macaque CD4 and CCR5 | [88, 108] | |||
stHIV-1 carrying | Pig-tailed macaques | Env-gp120 (V3) | Deletion of four amino acids (313TTGD316) | Association with coreceptor switch | [93] |
Vpu | One amino acid (I) insertion at position 15 and one amino acid substitution (V21G) | Antagonization to pig-tailed macaque tetherin | [93] | ||
stHIV-1-A19 | Pig-tailed macaques | Gag-CA | H87P, T107I, and I91A | Resistance to interferon alpha-inducible restriction factor Mx2 | [94] |
Table 2.
As expected, we found numerous growth-enhancing mutations in the Env proteins of the viral clones that were obtained from adaptation experiments in macaque cells using NL-DT5R/NL-DT562 (Table 2). Our
The stHIV-1 clone, which is currently the only AIDS-inducible clone, also has been shown to adapt to CD8-depleted macaque individuals (Table 2) [93, 94]. After the animal-to-animal adaptation using stHIV-1 clones carrying four distinct
6. Conclusion
The infection system consisting of HIV-1 derivative viruses and macaque hosts may sophisticatedly reflect, as an experimental model, the interaction between HIV-1 and its host humans. In an effort to establish HIV-1-infected macaque models, a noteworthy result was obtained that stHIV-1/stHIV-1-A19 could induce AIDS in CD8-depleted pigtailed macaques after some animal-to-animal adaptations. However, this is the only case that showed the pathogenicity of the HIV-1 derivative virus in macaques as fully described above. Furthermore, the viruses were unable to cause the disease in naïve animals. And, it is not clarified yet whether certain viral gene(s) and/or viral genomic region(s) are linked to viral pathogenicity. Totally, based on the experimental results obtained so far, we may predict that the pathogenic viruses such as some SIVs and SHIVs grew quite well at the early infection phase in individuals so as to obtain variations sufficient to persist in host individuals in the presence of strong host antiviral immunity. If the viruses could persist and maintain the critical set-point level in hosts, they might finally cause AIDS and AIDS-related complex. Further experimental studies are required to confirm this perspective.
It should be mentioned here as a virologically critically important matter that through the generation of macaque-tropic HIV-1 derivatives and a series of their infection experiments in macaque cells and individuals, we and others could learn how HIV-1 mutate and adapt itself to restrictive environments. Numerous synonymous and non-synonymous mutational changes in the HIV-1 genome that significantly affect viral replication and pathogenicity have been successfully identified. The identified alterations are closely linked to our understanding as to how HIV-1 replicates in host cells through modulating functional domains/regions/activity of its genome and proteins such as viral RNA splicing and viral proteins’ binding to cellular receptors and to some other replication-relevant cellular factors. Findings obtained using macaque-tropic HIV-1 derivatives have already greatly contributed to and would also play a significant role in understanding the HIV-1 biology.
Acknowledgments
We thank Yayoi Shono (Tokushima University) for experimental assistance. We also thank Kazuko Yoshida (Tokushima University) and Kyoko Inui (Tokushima University) for editorial and administrative assistance.
AA and MN conceived the idea and wrote a draft/the final manuscript. TaK, ND, BQL, and ToK reviewed it and discussed its content. All authors approved submission.
This work was supported in part by JSPS KAKENHI Grant Numbers 21K07042 to MN, 22K07102 to TaK, 21K08491 to ND, and 20K18484 to ToK, and by grants from the Takeda Science Foundation and The Uehara Memorial Foundation to TaK.
Notes/thanks/other declarations
Our recent studies described in this chapter have been done in collaboration with the following researchers: Masaru Yokoyama, Osamu Kotani, Hironori Sato, Kei Miyakawa, and Akihide Ryo (National Institute of Infectious Diseases, Japan). We are indebted to these scientists for their critical contribution to our work. We also thank our all staffs in our department and the other institutions who have supported our work.
Many original articles reporting the scientifically new and important findings could not be cited due to the tremendous numbers of publications and the space limitations. We express our sincere regret over these omissions based on rather subjective considerations.
Abbreviations
human immunodeficiency virus type 1 | |
nonhuman primate | |
acquired immunodeficiency syndrome | |
simian immunodeficiency virus | |
chimeric viruses of SIV and HIV-1 | |
tripartite motif 5 | |
apolipoprotein B mRNA editing enzyme, catalytic polypeptide-like 3 | |
simian-tropic HIV-1 | |
macaque-tropic HIV-1 | |
pigtailed macaque-tropic HIV-1 | |
rhesus macaque-tropic HIV-1 |
References
- 1.
Goff SP. Retroviridae. In: Knipe DM, Howley PM, editors. Fields virology. 6th ed. Philadelphia PA: Lippincott Williams & Wilkins; 2013. pp. 1424-1473 - 2.
Freed EO, Martin MA. Human immunodeficiency viruses: Replication. In: Knipe DM, Howley PM, editors. Fields virology. 6th ed. Philadelphia PA: Lippincott Williams & Wilkins; 2013. pp. 1502-1560 - 3.
Kuritzkes DR, Koup RA. HIV-1: Pathogenesis, clinical manifestations, and treatment. In: Knipe DM, Howley PM, editors. Fields Virology. 6th ed. Philadelphia PA: Lippincott Williams & Wilkins; 2013. pp. 1561-1583 - 4.
Evans DT, Elder JH, Desrosiers RC. Nonhuman Lentiviruses. In: Knipe DM, Howley PM, editors. Fields Virology. 6th ed. Philadelphia PA: Lippincott Williams & Wilkins; 2013. pp. 1584-1612 - 5.
Victor GJ. Humanized mice for HIV and AIDS research. Current Opinion in Virology. 2016; 19 :56-64. DOI: 10.1016/j.coviro.2016.06.010 - 6.
Marsden MD, Zack JA. Humanized mouse models for human immunodeficiency virus infection. Annual Reviews of Virology. 2017; 4 :393-412. DOI: 10.1146/annurev-virology-101416-041703 - 7.
Gillgrass A, Wessels JM, Yang JX, Kaushic C. Advances in humanized mouse models to improve understanding of HIV-1 pathogenesis and immune responses. Frontiers in Immunology. 2021; 11 :617516. DOI: 10.3389/fimmu.2020.617516 - 8.
Terahara K, Iwabuchi R, Tsunetsugu-Yokota Y. Perspectives on non-BLT humanized mouse models for studying HIV pathogenesis and therapy. Viruses. 2021; 13 :776. DOI: 10.3390/v13050776 - 9.
Baroncini L, Bredl S, Nicole KP, Speck RF. The humanized mouse model: What added value does it offer for HIV research? Pathogens. 2023; 12 :608. DOI: 10.3390/pathogens12040608 - 10.
Ambrose Z, KewalRamani VN, Bieniasz PD, Hatziioannou T. HIV/AIDS: In search of an animal model. Trends in Biotechnology. 2007; 25 :333-337. DOI: 10.1016/j.tibtech.2007.05.004 - 11.
Nomaguchi M, Doi N, Kamada K, Adachi A. Species barrier of HIV-1 and its jumping by virus engineering. Reviews in Medical Virology. 2008; 18 :261-275. DOI: 10.1002/rmv.576 - 12.
Nomaguchi M, Doi N, Fujiwara S, Adachi A. Macaque-tropic HIV-1 derivatives: A novel experimental approach to understand viral replication and evolution in vivo . In: Chang TL, editor. HIV-Host Interactions. London, UK, Rijeka, Croatia: IntechOpen; 2011. pp. 325-348. DOI: 10.5772/23624 - 13.
Hatziioannou T, Evans DT. Animal models for HIV/AIDS research. Nature Reviews Microbiology. 2012; 10 :852-867. DOI: 10.1038/nrmicro2911 - 14.
Misra A, Thippeshappa R, Kimata JT. Macaques as model hosts for studies of HIV-1 infection. Frontiers in Microbiology. 2013; 4 :176. DOI: 10.3389/fmicb.2013.00176 - 15.
Saito A, Akari H. Macaque-tropic human immunodeficiency virus type 1: Breaking out of the host restriction factors. Frontiers in Microbiology. 2013; 4 :187. DOI: 10.3389/fmicb.2013.00187 - 16.
Garcia-Tellez T, Huot N, Ploquin MJ, Rascle P, Jacquelin B, Müller-Trutwin M. Non-human primates in HIV research: Achievements, limits and alternatives. Infection, Genetics and Evolution. 2016; 46 :324-332. DOI: 10.1016/j.meegid.2016.07.012 - 17.
Nishimura Y, Martin MA. Of mice, macaques, and men: Broadly neutralizing antibody immunotherapy for HIV-1. Cell Host & Microbe. 2017; 22 :207-216. DOI: 10.1016/j.chom.2017.07.010 - 18.
Thippeshappa R, Kimata JT, Kaushal D. Toward a macaque model of HIV-1 infection: Roadblocks, progress, and future strategies. Frontiers in Microbiology. 2020; 11 :882. DOI: 10.3389/fmicb.2020.00882 - 19.
Shibata R, Sakai H, Kawamura M, Tokunaga K, Adachi A. Early replication block of human immunodeficiency virus type 1 in monkey cells. Journal of General Virology. 1995; 76 :2723-2730. DOI: 10.1099/0022-1317-76-11-2723 - 20.
Kirchhoff F. Immune evasion and counteraction of restriction factors by HIV-1 and other primate lentiviruses. Cell Host & Microbe. 2010; 8 :55-67. DOI: 10.1016/j.chom.2010.06.004 - 21.
Blanco-Melo D, Venkatesh S, Bieniasz PD. Intrinsic cellular defenses against human immunodeficiency viruses. Immunity. 2012; 37 :399-411. DOI: 10.1016/j.immuni.2012.08.013 - 22.
Harris RS, Hultquist JF, Evans DT. The restriction factors of human immunodeficiency virus. Journal of Biological Chemistry. 2012; 287 :40875-40883. DOI: 10.1074/jbc.R112.416925 - 23.
Malim MH, Bieniasz PD. HIV restriction factors and mechanisms of evasion. Cold Spring Harbor Perspectives in Medicine. 2012; 2 :a006940. DOI: 10.1101/cshperspect.a006940 - 24.
Kirchhoff F. Is the high virulence of HIV-1 an unfortunate coincidence of primate lentiviral evolution? Nature Reviews Microbiology. 2009; 7 :467-476. DOI: 10.1038/nrmicro2111 - 25.
Sharp PM, Hahn BH. Origins of HIV and the AIDS pandemic. Cold Spring Harbor Perspectives in Medicine. 2011; 1 :a006841. DOI: 10.1101/cshperspect.a006841 - 26.
Sakai Y, Miyake A, Doi N, Sasada H, Miyazaki Y, Adachi A, et al. Expression of profiles of Vpx/Vpr proteins are co-related with the primate lentiviral lineage. Frontiers in Microbiology. 2016; 7 :1211. DOI: 10.3389/fmicb.2016.01211 - 27.
Sakai Y, Doi N, Miyazaki Y, Adachi A, Nomaguchi M. Phylogenetic insights into the functional relationship between primate lentiviral reverse transcriptase and accessory proteins Vpx/Vpr. Frontiers in Microbiology. 2016; 7 :1655. DOI: 10.3389/fmicb.2016.01655 - 28.
Kleinman AJ, Pandrea I, Apetrei C. So pathogenic or so what?-a brief overview of SIV pathogenesis with an emphasis on cure research. Viruses. 2022; 14 :135. DOI: 10.3390/v14010135 - 29.
Shibata R, Kawamura M, Sakai H, Hayami M, Ishimoto A, Adachi A. Generation of a chimeric human and simian immunodeficiency virus infectious to monkey peripheral blood mononuclear cells. Journal of Virology. 1991; 65 :3514-3520. DOI: 10.1128/JVI.65.7.3514-3520.1991 - 30.
Sakuragi S, Shibata R, Mukai R, Komatsu T, Fukasawa M, Sakai H, et al. Infection of macaque monkeys with a chimeric human and simian immunodeficiency virus. Journal of General Virology. 1992; 73 :2983-2987. DOI: 10.1099/0022-1317-73-11-2983 - 31.
Li J, Lord CI, Haseltine W, Letvin NL, Sodroski J. Infection of cynomolgus monkeys with a chimeric HIV-1/SIVmac virus that expresses the HIV-1 envelope glycoproteins. Journal of Acquired Immune Deficiency Syndromes. 1988; 1992 (5):639-646 - 32.
Uberla K, Stahl-Hennig C, Böttiger D, Mätz-Rensing K, Kaup FJ, Li J, et al. Animal model for the therapy of acquired immunodeficiency syndrome with reverse transcriptase inhibitors. Proceedings of the National Academy of Sciences of the United States of America. 1995; 92 :8210-8214. DOI: 10.1073/pnas.92.18.8210 - 33.
Hatziioannou T, Princiotta M, Piatak M Jr, Yuan F, Zhang F, Lifson JD, et al. Generation of simian-tropic HIV-1 by restriction factor evasion. Science. 2006; 314 :95. DOI: 10.1126/science.1130994 - 34.
Kamada K, Igarashi T, Martin MA, Khamsri B, Hatcho K, Yamashita T, et al. Generation of HIV-1 derivatives that productively infect macaque monkey lymphoid cells. Proceedings of the National Academy of Sciences of the United States of America. 2006; 103 :16959-16964. DOI: 10.1073/pnas.0608289103 - 35.
Igarashi T, Iyengar R, Byrum RA, Buckler-White A, Dewar RL, Buckler CE, et al. Human immunodeficiency virus type 1 derivative with 7% simian immunodeficiency virus genetic content is able to establish infections in pig-tailed macaques. Journal of Virology. 2007; 81 :11549-11552. DOI: 10.1128/JVI.00960-07 - 36.
Ishimatsu M, Suzuki H, Akiyama H, Miura T, Hayami M, Ido E. Construction of a novel SHIV having an HIV-1-derived protease gene and its infection to rhesus macaques: A useful tool for in vivo efficacy tests of protease inhibitors. Microbes and Infection. 2007; 9 :475-482. DOI: 10.1016/j.micinf.2007.01.005 - 37.
Hatziioannou T, Ambrose Z, Chung NP, Piatak M Jr, Yuan F, Trubey CM, et al. A macaque model of HIV-1 infection. Proceedings of the National Academy of Sciences of the United States of America. 2009; 106 :4425-4429. DOI: 10.1073/pnas.0812587106 - 38.
Nishimura Y, Shingai M, Willey R, Sadjadpour R, Lee WR, Brown CR, et al. Generation of the pathogenic R5-tropic simian/human immunodeficiency virus SHIVAD8 by serial passaging in rhesus macaques. Journal of Virology. 2010; 84 :4769-4781. DOI: 10.1128/JVI.02279-09 - 39.
Saito A, Nomaguchi M, Iijima S, Kuroishi A, Yoshida T, Lee YJ, et al. Improved capacity of a monkey-tropic HIV-1 derivative to replicate in cynomolgus monkeys with minimal modifications. Microbes and Infection. 2011; 13 :58-64. DOI: 10.1016/j.micinf.2010.10.001 - 40.
Thippeshappa R, Polacino P, Yu Kimata MT, Siwak EB, Anderson D, Wang W, et al. Vif substitution enables persistent infection of pig-tailed macaques by human immunodeficiency virus type 1. Journal of Virology. 2011; 85 :3767-3779. DOI: 10.1128/JVI.02438-10 - 41.
Shingai M, Donau OK, Schmidt SD, Gautam R, Plishka RJ, Buckler-White A, et al. Most rhesus macaques infected with the CCR5-tropic SHIV(AD8) generate cross-reactive antibodies that neutralize multiple HIV-1 strains. Proceedings of the National Academy of Sciences of the United States of America. 2012; 109 :19769-19774. DOI: 10.1073/pnas.1217443109 - 42.
Saito A, Nomaguchi M, Kono K, Iwatani Y, Yokoyama M, Yasutomi Y, et al. TRIM5 genotypes in cynomolgus monkeys primarily influence inter-individual diversity in susceptibility to monkey-tropic human immunodeficiency virus type 1. Journal of General Virology. 2013; 94 :1318-1324. DOI: 10.1099/vir.0.050252-0 - 43.
Nomaguchi M, Yokoyama M, Kono K, Nakayama EE, Shioda T, Doi N, et al. Generation of rhesus macaque-tropic HIV-1 clones that are resistant to major anti-HIV-1 restriction factors. Journal of Virology. 2013; 87 :11447-11461. DOI: 10.1128/JVI.01549-13 - 44.
Doi N, Miura T, Mori H, Sakawaki H, Koma T, Adachi A, et al. CXCR4- and CCR5-tropic HIV-1 clones are both tractable to grow in rhesus macaques. Frontiers in Microbiology. 2018; 9 :2510. DOI: 10.3389/fmicb.2018.02510 - 45.
Rosenwirth B, ten Haaft P, Bogers WM, Nieuwenhuis IG, Niphuis H, Kuhn EM, et al. Antiretroviral therapy during primary immunodeficiency virus infection can induce persistent suppression of virus load and protection from heterologous challenge in rhesus macaques. Journal of Virology. 2000; 74 :1704-1711. DOI: 10.1128/jvi.74.4.1704-1711.2000 - 46.
Hofman MJ, Higgins J, Matthews TB, Pedersen NC, Tan C, Schinazi RF, et al. Efavirenz therapy in rhesus macaques infected with a chimera of simian immunodeficiency virus containing reverse transcriptase from human immunodeficiency virus type 1. Antimicrobial Agents and Chemotherapy. 2004; 48 :3483-3490. DOI: 10.1128/AAC.48.9.3483-3490.2004 - 47.
Ambrose Z, Boltz V, Palmer S, Coffin JM, Hughes SH, Kewalramani VN. In vitro characterization of a simian immunodeficiency virus-human immunodeficiency virus (HIV) chimera expressing HIV type 1 reverse transcriptase to study antiviral resistance in pigtail macaques. Journal of Virology. 2004; 78 :13553-13561. DOI: 10.1128/JVI.78.24.13553-13561.2004 - 48.
North TW, Van Rompay KK, Higgins J, Matthews TB, Wadford DA, Pedersen NC, et al. Suppression of virus load by highly active antiretroviral therapy in rhesus macaques infected with a recombinant simian immunodeficiency virus containing reverse transcriptase from human immunodeficiency virus type 1. Journal of Virology. 2005; 79 :7349-7354. DOI: 10.1128/JVI.79.12.7349-7354.2005 - 49.
Van Rompay KK, Johnson JA, Blackwood EJ, Singh RP, Lipscomb J, Matthews TB, et al. Sequential emergence and clinical implications of viral mutants with K70E and K65R mutation in reverse transcriptase during prolonged tenofovir monotherapy in rhesus macaques with chronic RT-SHIV infection. Retrovirology. 2007; 4 :25. DOI: 10.1186/1742-4690-4-25 - 50.
Ambrose Z, Palmer S, Boltz VF, Kearney M, Larsen K, Polacino P, et al. Suppression of viremia and evolution of human immunodeficiency virus type 1 drug resistance in a macaque model for antiretroviral therapy. Journal of Virology. 2007; 81 :12145-12155. DOI: 10.1128/JVI.01301-07 - 51.
Shao W, Kearney M, Maldarelli F, Mellors JW, Stephens RM, Lifson JD, et al. RT-SHIV subpopulation dynamics in infected macaques during anti-HIV therapy. Retrovirology. 2009; 6 :101. DOI: 10.1186/1742-4690-6-101 - 52.
North TW, Higgins J, Deere JD, Hayes TL, Villalobos A, Adamson L, et al. Viral sanctuaries during highly active antiretroviral therapy in a nonhuman primate model for AIDS. Journal of Virology. 2010; 84 :2913-2922. DOI: 10.1128/JVI.02356-09 - 53.
Kearney M, Spindler J, Shao W, Maldarelli F, Palmer S, Hu SL, et al. Genetic diversity of simian immunodeficiency virus encoding HIV-1 reverse transcriptase persists in macaques despite antiretroviral therapy. Journal of Virology. 2011; 85 :1067-1076. DOI: 10.1128/JVI.01701-10 - 54.
Boltz VF, Ambrose Z, Kearney MF, Shao W, Kewalramani VN, Maldarelli F, et al. Ultrasensitive allele-specific PCR reveals rare preexisting drug-resistant variants and a large replicating virus population in macaques infected with a simian immunodeficiency virus containing human immunodeficiency virus reverse transcriptase. Journal of Virology. 2012; 86 :12525-12530. DOI: 10.1128/JVI.01963-12 - 55.
Gavegnano C, Detorio M, Montero C, Bosque A, Planelles V, Schinazi RF. Ruxolitinib and tofacitinib are potent and selective inhibitors of HIV-1 replication and virus reactivation in vitro. Antimicrobial Agents and Chemotherapy. 2014; 58 :1977-1986. DOI: 10.1128/AAC.02496-13 - 56.
North TW, Villalobos A, Hurwitz SJ, Deere JD, Higgins J, Chatterjee P, et al. Enhanced antiretroviral therapy in rhesus macaques improves RT-SHIV viral decay kinetics. Antimicrobial Agents and Chemotherapy. 2014; 58 :3927-3933. DOI: 10.1128/AAC.02522-14 - 57.
Kearney MF, Anderson EM, Coomer C, Smith L, Shao W, Johnson N, et al. Well-mixed plasma and tissue viral populations in RT-SHIV-infected macaques implies a lack of viral replication in the tissues during antiretroviral therapy. Retrovirology. 2015; 12 :93. DOI: 10.1186/s12977-015-0212-2 - 58.
Melody K, McBeth S, Kline C, Kashuba AD, Mellors JW, Ambrose Z. Low frequency of drug-resistant variants selected by long-acting rilpivirine in macaques infected with simian immunodeficiency virus containing HIV-1 reverse transcriptase. Antimicrobial Agents and Chemotherapy. 2015; 59 :7762-7770. DOI: 10.1128/AAC.01937-15 - 59.
Akiyama H, Ramirez NP, Gibson G, Kline C, Watkins S, Ambrose Z, et al. Interferon-inducible CD169/siglec1 attenuates anti-HIV-1 effects of alpha interferon. Journal of Virology. 2017; 91 :e00972-e00917. DOI: 10.1128/JVI.00972-17 - 60.
Luciw PA, Pratt-Lowe E, Shaw KE, Levy JA, Cheng-Mayer C. Persistent infection of rhesus macaques with T-cell-line-tropic and macrophage-tropic clones of simian/human immunodeficiency viruses (SHIV). Proceedings of the National Academy of Sciences of the United States of America. 1995; 92 :7490-7494. DOI: 10.1073/pnas.92.16.7490 - 61.
Letvin NL, Montefiori DC, Yasutomi Y, Perry HC, Davies ME, Lekutis C, et al. Potent, protective anti-HIV immune responses generated by bimodal HIV envelope DNA plus protein vaccination. Proceedings of the National Academy of Sciences of the United States of America. 1997; 94 :9378-9383. DOI: 10.1073/pnas.94.17.9378 - 62.
Shibata R, Igarashi T, Haigwood N, Buckler-White A, Ogert R, Ross W, et al. Neutralizing antibody directed against the HIV-1 envelope glycoprotein can completely block HIV-1/SIV chimeric virus infections of macaque monkeys. Nature Medicine. 1999; 5 :204-210. DOI: 10.1038/5568 - 63.
Baba TW, Liska V, Hofmann-Lehmann R, Vlasak J, Xu W, Ayehunie S, et al. Human neutralizing monoclonal antibodies of the IgG1 subtype protect against mucosal simian-human immunodeficiency virus infection. Nature Medicine. 2000; 6 :200-206. DOI: 10.1038/72309 - 64.
Barouch DH, Santra S, Schmitz JE, Kuroda MJ, Fu TM, Wagner W, et al. Control of viremia and prevention of clinical AIDS in rhesus monkeys by cytokine-augmented DNA vaccination. Science. 2000; 290 :486-492. DOI: 10.1126/science.290.5491.486 - 65.
Walker LM, Sok D, Nishimura Y, Donau O, Sadjadpour R, Gautam R, et al. Rapid development of glycan-specific, broad, and potent anti-HIV-1 gp120 neutralizing antibodies in an R5 SIV/HIV chimeric virus infected macaque. Proceedings of the National Academy of Sciences of the United States of America. 2011; 108 :20125-20129. DOI: 10.1073/pnas.1117531108 - 66.
Georgiev IS, Doria-Rose NA, Zhou T, Kwon YD, Staupe RP, Moquin S, et al. Delineating antibody recognition in polyclonal sera from patterns of HIV-1 isolate neutralization. Science. 2013; 340 :751-756. DOI: 10.1126/science.1233989 - 67.
Shingai M, Nishimura Y, Klein F, Mouquet H, Donau OK, Plishka R, et al. Antibody-mediated immunotherapy of macaques chronically infected with SHIV suppresses viraemia. Nature. 2013; 503 :277-280. DOI: 10.1038/nature12746 - 68.
Del Prete GQ , Eilers B, Moldt B, Keele BF, Estes JD, Rodriguez A, et al. Selection of unadapted, pathogenic SHIVs encoding newly transmitted HIV-1 envelope proteins. Cell Host & Microbe. 2014; 16 :412-418. DOI: 10.1016/j.chom.2014.08.003 - 69.
Ko SY, Pegu A, Rudicell RS, Yang ZY, Joyce MG, Chen X, et al. Enhanced neonatal fc receptor function improves protection against primate SHIV infection. Nature. 2014; 514 :642-645. DOI: 10.1038/nature13612 - 70.
Shingai M, Donau OK, Plishka RJ, Buckler-White A, Mascola JR, Nabel GJ, et al. Passive transfer of modest titers of potent and broadly neutralizing anti-HIV monoclonal antibodies block SHIV infection in macaques. The Journal of experimental medicine. 2014; 211 :2061-2074. DOI: 10.1084/jem.20132494 - 71.
Klein F, Nogueira L, Nishimura Y, Phad G, West AP Jr, Halper-Stromberg A, et al. Enhanced HIV-1 immunotherapy by commonly arising antibodies that target virus escape variants. The Journal of Experimental Medicine. 2014; 211 :2361-2372. DOI: 10.1084/jem.20141050 - 72.
Francica JR, Sheng Z, Zhang Z, Nishimura Y, Shingai M, Ramesh A, et al. Analysis of immunoglobulin transcripts and hypermutation following SHIV(AD8) infection and protein-plus-adjuvant immunization. Nature. Communications. 2015; 6 :6565. DOI: 10.1038/ncomms7565 - 73.
Reeves RK, Li H, Jost S, Blass E, Li H, Schafer JL, et al. Antigen-specific NK cell memory in rhesus macaques. Nature Immunology. 2015; 16 :927-932. DOI: 10.1038/ni.3227 - 74.
Gautam R, Nishimura Y, Pegu A, Nason MC, Klein F, Gazumyan A, et al. A single injection of anti-HIV-1 antibodies protects against repeated SHIV challenges. Nature. 2016; 533 :105-109. DOI: 10.1038/nature17677 - 75.
Li H, Wang S, Kong R, Ding W, Lee FH, Parker Z, et al. Envelope residue 375 substitutions in simian-human immunodeficiency viruses enhance CD4 binding and replication in rhesus macaques. Proceedings of the National Academy of Sciences of the United States of America. 2016; 113 :E3413-E3422. DOI: 10.1073/pnas.1606636113 - 76.
Nishimura Y, Gautam R, Chun TW, Sadjadpour R, Foulds KE, Shingai M, et al. Early antibody therapy can induce long-lasting immunity to SHIV. Nature. 2017; 543 :559-563. DOI: 10.1038/nature21435 - 77.
Gautam R, Nishimura Y, Gaughan N, Gazumyan A, Schoofs T, Buckler-White A, et al. A single injection of crystallizable fragment domain-modified antibodies elicits durable protection from SHIV infection. Nature Medicine. 2016; 24 :610-616. DOI: 10.1038/s41591-018-0001-2 - 78.
Madani N, Princiotto AM, Mach L, Ding S, Prevost J, Richard J, et al. A CD4-mimetic compound enhances vaccine efficacy against stringent immunodeficiency virus challenge. Nature Communications. 2018; 9 :2363. DOI: 10.1038/s41467-018-04758-9 - 79.
Borducchi EN, Liu J, Nkolola JP, Cadena AM, Yu WH, Fischinger S, et al. Antibody and TLR7 agonist delay viral rebound in SHIV-infected monkeys. Nature. 2018; 563 :360-364. DOI: 10.1038/s41586-018-0600-6 - 80.
Fan J, Liang H, Ji X, Wang S, Xue J, Li D, et al. CTL-mediated immunotherapy can suppress SHIV rebound in ART-free macaques. Nature Communications. 2019; 10 :2257. DOI: 10.1038/s41467-019-09725-6 - 81.
Pegu A, Borate B, Huang Y, Pauthner MG, Hessell AJ, Julg B, et al. A meta-analysis of passive immunization studies shows that serum-neutralizing antibody titer associates with protection against SHIV challenge. Cell Host & Microbe. 2019; 26 :336-346.e3. DOI: 10.1016/j.chom.2019.08.014 - 82.
Terrade G, Huot N, Petitdemange C, Lazzerini M, Orta Resendiz A, Jacquelin B, et al. Interests of the non-human primate models for HIV cure research. Vaccines (Basel). 2021; 9 :958. DOI: 10.3390/vaccines9090958 - 83.
Liang B, Li H, Li L, Omange RW, Hai Y, Luo M. Current advances in HIV vaccine preclinical studies using Macaque models. Vaccine. 2019; 37 :3388-3399. DOI: 10.1016/j.vaccine.2019.04.094 - 84.
Nomaguchi M, Doi N, Matsumoto Y, Sakai Y, Fujiwara S, Adachi A. Species tropism of HIV-1 modulated by viral accessory proteins. Frontiers in Microbiology. 2012; 3 :267. DOI: 10.3389/fmicb.2012.00267 - 85.
Fujita M, Yoshida A, Miyaura M, Sakurai A, Akari H, Koyama AH, et al. Cyclophilin A-independent replication of a human immunodeficiency virus type 1 isolate carrying a small portion of the simian immunodeficiency virus SIV(MAC) gag capsid region. Journal of Virology. 2001;75 :10527-10531. DOI: 10.1128/JVI.75.21.10527-10531.2001 - 86.
Kamada K, Yoshida A, Khamsri B, Piroozmand A, Yamashita T, Uchiyama T, et al. Construction of gag -chimeric viruses between HIV-1 and SIVmac that are capable of productive multi-cycle infection. Microbes and Infection. 2006;8 :1075-1081. DOI: 10.1016/j.micinf.2005.11.006 - 87.
Nomaguchi M, Yokoyama M, Kono K, Nakayama EE, Shioda T, Saito A, et al. Gag-CA Q110D mutation elicits TRIM5-independent enhancement of HIV-1mt replication in macaque cells. Microbes and Infection. 2013; 15 :56-65. DOI: 10.1016/j.micinf.2012.10.013 - 88.
Nomaguchi M, Doi N, Fujiwara S, Saito A, Akari H, Nakayama EE, et al. Systemic biological analysis of the mutations in two distinct HIV-1mt genomes occurred during replication in macaque cells. Microbes and Infection. 2013; 15 :319-328. DOI: 10.1016/j.micinf.2013.01.005 - 89.
Kawamura M, Ishizaki T, Ishimoto A, Shioda T, Kitamura T, Adachi A. Growth ability of human immunodeficiency virus type 1 auxiliary gene mutants in primary blood macrophage cultures. Journal of General Virology. 1994; 75 :2427-2431. DOI: 10.1099/0022-1317-75-9-2427 - 90.
Adachi A, Gendelman HE, Koenig S, Folks T, Willey R, Rabson A, et al. Production of acquired immunodeficiency syndrome-associated retrovirus in human and nonhuman cells transfected with an infectious molecular clone. Journal of Virology. 1986; 59 :284-291. DOI: 10.1128/JVI.59.2.284-291.1986 - 91.
Kamada K, Yamashita T, Hatcho K, Adachi A, Nomaguchi M. Evasion from CypA- and APOBEC-mediated restrictions is insufficient for HIV-1 to efficiently grow in simian cells. Microbes and Infection. 2009; 11 :164-171. DOI: 10.1016/j.micinf.2008.11.002 - 92.
Kuroishi A, Saito A, Shingai Y, Shioda T, Nomaguchi M, Adachi A, et al. Modification of a loop sequence between alpha-helices 6 and 7 of virus capsid (CA) protein in a human immunodeficiency virus type 1 (HIV-1) derivative that has simian immunodeficiency virus (SIVmac239) vif and CA alpha-helices 4 and 5 loop improves replication in cynomolgus monkey cells. Retrovirology. 2009;6 :70. DOI: 10.1186/1742-4690-6-70 - 93.
Hatziioannou T, Del Prete GQ , Keele BF, Estes JD, McNatt MW, Bitzegeio J, et al. HIV-1-induced AIDS in monkeys. Science. 2014; 344 :1401-1405. DOI: 10.1126/science.1250761 - 94.
Schmidt F, Keele BF, Del Prete GQ , Voronin D, Fennessey CM, Soll S, et al. Derivation of simian tropic HIV-1 infectious clone reveals virus adaptation to a new host. Proceedings of the National Academy of Sciences of the United States of America. 2019; 116 :10504-10509. DOI: 10.1073/pnas.1818059116 - 95.
Thippeshappa R, Ruan H, Wang W, Zhou P, Kimata JT. A variant macaque-tropic human immunodeficiency virus type 1 is resistant to alpha interferon-induced restriction in pig-tailed macaque CD4+ T cells. Journal of Virology. 2013; 87 :6678-6692. DOI: 10.1128/JVI.00338-13 - 96.
Nomaguchi M, Nakayama EE, Yokoyama M, Doi N, Igarashi T, Shioda T, et al. Distinct combinations of amino acid substitutions in N-terminal domain of Gag-capsid afford HIV-1 resistance to rhesus TRIM5α. Microbes and Infection. 2014; 16 :936-944. DOI: 10.1016/j.micinf.2014.08.017 - 97.
Soll SJ, Wilson SJ, Kutluay SB, Hatziioannou T, Bieniasz PD. Assisted evolution enables HIV-1 to overcome a high TRIM5α-imposed genetic barrier to rhesus macaque tropism. PLoS Pathogens. 2013; 9 :e1003667. DOI: 10.1371/journal.ppat.1003667 - 98.
Dorfman T, Göttlinger HG. The human immunodeficiency virus type 1 capsid p2 domain confers sensitivity to the cyclophilin-binding drug SDZ NIM 811. Journal of Virology. 1996; 70 :5751-5757. DOI: 10.1128/JVI.70.9.5751-5757.1996 - 99.
McCarthy KR, Schmidt AG, Kirmaier A, Wyand AL, Newman RM, Johnson WE. Gain-of-sensitivity mutations in a Trim5-resistant primary isolate of pathogenic SIV identify two independent conserved determinants of Trim5α specificity. PLoS Pathogens. 2013; 9 :e1003352. DOI: 10.1371/journal.ppat.1003352 - 100.
Ganser-Pornillos BK, Chandrasekaran V, Pornillos O, Sodroski JG, Sundquist WI, Yeager M. Hexagonal assembly of a restricting TRIM5alpha protein. Proceedings of the National Academy of Sciences of the United States of America. 2011; 108 :534-539. DOI: 10.1073/pnas.1013426108 - 101.
Biris N, Yang Y, Taylor AB, Tomashevski A, Guo M, Hart PJ, et al. Structure of the rhesus monkey TRIM5α PRYSPRY domain, the HIV capsid recognition module. Proceedings of the National Academy of Sciences of the United States of America. 2012; 109 :13278-13283. DOI: 10.1073/pnas.1203536109 - 102.
Yang H, Ji X, Zhao G, Ning J, Zhao Q , Aiken C, et al. Structural insight into HIV-1 capsid recognition by rhesus TRIM5α. Proceedings of the National Academy of Sciences of the United States of America. 2012; 109 :18372-18377. DOI: 10.1073/pnas.1210903109 - 103.
Biris N, Tomashevski A, Bhattacharya A, Diaz-Griffero F, Ivanov DN. Rhesus monkey TRIM5α SPRY domain recognizes multiple epitopes that span several capsid monomers on the surface of the HIV-1 mature viral core. Journal of Molecular Biology. 2013; 425 :5032-5044. DOI: 10.1016/j.jmb.2013.07.025 - 104.
Nomaguchi M, Miyake A, Doi N, Fujiwara S, Miyazaki Y, Tsunetsugu-Yokota Y, et al. Natural single-nucleotide polymorphisms in the 3′ region of the HIV-1 pol gene modulate viral replication ability. Journal of Virology. 2014;88 :4145-4160. DOI: 10.1128/JVI.01859-13 - 105.
Nomaguchi M, Doi N, Sakai Y, Ode H, Iwatani Y, Ueno T, et al. Natural single-nucleotide variations in the HIV-1 genomic SA1prox region can alter viral replication ability by regulating Vif expression levels. Journal of Virology. 2016; 90 :4563-4578. DOI: 10.1128/JVI.02939-15 - 106.
Doi N, Yokoyama M, Koma T, Kotani O, Sato H, Adachi A, et al. Concomitant enhancement of HIV-1 replication potential and neutralization-resistance in concert with three adaptive mutations in Env V1/C2/C4 domains. Frontiers in Microbiology. 2019; 10 :2. DOI: 10.3389/fmicb.2019.00002 - 107.
Yokoyama M, Nomaguchi M, Doi N, Kanda T, Adachi A, Sato H. In silico analysis of HIV-1 Env-gp120 reveals structural bases for viral adaptation in growth-restrictive cells. Frontiers in Microbiology. 2016;7 :110. DOI: 10.3389/fmicb.2016.00110 - 108.
Koma T, Yokoyama M, Kotani O, Doi N, Nakanishi N, Okubo H, et al. Species-specific valid ternary interactions of HIV-1 Env-gp120, CD4, and CCR5 as revealed by an adaptive single-amino acid substitution at the V3 loop tip. Journal of Virology. 2021; 95 :e0217720. DOI: 10.1128/JVI.02177-20 - 109.
Kammler S, Otte M, Hauber I, Kjems J, Hauber J, Schaal H. The strength of the HIV-1 3′ splice sites affects rev function. Retrovirology. 2006; 3 :89. DOI: 10.1186/1742-4690-3-89 - 110.
Exline CM, Feng Z, Stoltzfus CM. Negative and positive mRNA splicing elements act competitively to regulate human immunodeficiency virus type 1 vif gene expression. Journal of Virology. 2008; 82 :3921-3931. DOI: 10.1128/JVI.01558-07 - 111.
Mandal D, Exline CM, Feng Z, Stoltzfus CM. Regulation of vif mRNA splicing by human immunodeficiency virus type 1 requires 5′ splice site D2 and an exonic splicing enhancer to counteract cellular restriction factor APOBEC3G. Journal of Virology. 2009;83 :6067-6078. DOI: 10.1128/JVI.02231-08 - 112.
Widera M, Erkelenz S, Hillebrand F, Krikoni A, Widera D, Kaisers W, et al. An intronic G run within HIV-1 intron 2 is critical for splicing regulation of vif mRNA. Journal of Virology. 2013;87 :2707-2720. DOI: 10.1128/JVI.02755-12 - 113.
Brillen AL, Walotka L, Hillebrand F, Müller L, Widera M, Theiss S, et al. Analysis of competing HIV-1 splice donor sites uncovers a tight cluster of splicing regulatory elements within exon 2/2b. Journal of Virology. 2017; 91 :e00389-e00317. DOI: 10.1128/JVI.00389-17 - 114.
Nomaguchi M, Doi N, Yoshida T, Koma T, Adachi S, Ode H, et al. Production of HIV-1 vif mRNA is modulated by natural nucleotide variations and SLSA1 RNA structure in SA1D2prox genomic region. Frontiers in Microbiology. 2017;8 :2542. DOI: 10.3389/fmicb.2017.02542