Comparison of the efficiency of different types of electric motors.
Abstract
Compared with internal combustion engine vehicles, the trend toward electric vehicles that do not pollute the air, are highly efficient, and require less maintenance is increasing. In parallel with the increasing trend toward electric vehicles, research studies to provide optimal performance in electric vehicles are gaining speed. The choice of motor used in electric vehicles is a very serious decision as it will affect all the performance data. In the selection of the motor used in electric vehicles, simple design, high power density, low maintenance cost, and easy controllability features come to the fore. In electric vehicles, different types of electric motors are used according to the designer’s preference, including DC motor, brushless DC motor, induction motor, permanent magnet synchronous motor, and switched reluctance motor. Although different types of motors used in electric vehicles are included in the literature, induction motors are ahead of other types of electric motors in the market with their simple structures, high power density, durability, low cost, and advanced speed control techniques.
Keywords
- electrical vehicle
- induction motor
- high speed
- efficiency
- comparison
1. Introduction
Today, the trend toward electric vehicles (EVs), which are alternative solutions, is increasing, due to the fact that internal combustion engine vehicles increase carbon emissions, and countries remain dependent on oil-importing countries. Unlike traditional ICVs, EVs use electric motors instead of internal combustion motor to offer several outstanding features such as wide-torque speed, high power density, higher efficiency, longer range, reduced maintenance costs, and reduction in air pollution.
EVs provide the necessary power to push the vehicle by converting the electrical energy stored in the battery or other energy storage units into mechanical power with one or more electric motors. An electric vehicle consists of subcomponents such as battery, electric motor, power controller, and mechanical transmission (Figure 1).

Figure 1.
Main components of electric vehicles.
In addition to determining the optimal battery and power management system in order to increase the driving range and provide higher performance and efficiency in electric vehicles, the choice of electric motor is also a very important decision. In the selection of motors used in electric vehicles, simple design, high power density, low maintenance cost, and easy controllability features come to the fore [1]. In electric vehicles, different types of electric motors are used: DC motor, brushless DC motor (BLDC), induction motor (IM), permanent magnet synchronous motor (PMSM), and switched reluctance motor (SRM).
The first electric motors used in many prototype electric vehicles were DC series motors in the 1980s and before. DC motors are one of the simplest types of motors that can be connected directly to the vehicle’s battery, which can be controlled simply where speed regulation is provided by voltage change without the need for complex power electronics applications.
However, EVs where brushed DC motors are used meet the demand for high torque at low speeds, but cannot meet the torque demand required for high speeds due to the friction between the brush and collector used to transfer the armature current. In addition, lower efficiency due to lower power density than other types of motors in the same volume and the need for maintenance of brush-collector arrangements (about every 3000 h) are other negative aspects. Although the power density in DC motor is provided by commutation poles and compensation windings in the motor design, this is not preferred because it will lead to an increase in cost and size.
With the use of BLDC motors instead of brushed DC Motors, the need for high torque needed at high speeds is met. This motor can provide high torque under high current and voltage compared with other motor types [2]. In BLDC motors, commutation occurs by electronic commutation instead of the brush-collector arrangement in DC motors. A rotor position sensor is needed for electronic commutation to take place. However, the high cost of permanent magnets used in the rotors of these motors is a disadvantage. This negative situation constitutes an obstacle to the priority of use in EVs. In addition, the risk of demagnetization of magnets, which are one of the most affected parts of PMSMs by temperature, is also a negative feature. If such machines are preferred in EVs, extra liquid cooling systems and temperature-dependent current limitation via the motor drive are needed (9). Although the liquid cooling systems used to continuously increase the output power in EVs are seen as a solution to the temperature increase, they are not preferred much in low-power light electric vehicles (1.5 kW <Po> 15 kW) due to the cost increase and the integration problem that the additional unit will bring. Although DC motors have the appropriate torque-speed characteristics for EVs, the maximum speed restriction of DC motors and the magnet cost of BLDC motors restrict their use in electric vehicles.
In parallel with the advances in EV technology, the scarcity of magnetic materials and the increase in their cost have increased the trend toward SRM as an alternative motor type [3]. The robust salient-pole rotor structure is inexpensive and does not show sensitivity to temperature [4]. Due to its wide speed range, power-to-weight ratio, cost of motor and its controller, torque-speed characteristic, high starting torque, inherent fault-tolerance capability, EV is seen as a suitable choice option [5]. However, SRMs have the most important disadvantages such as high noise, vibrations, and torque fluctuation during operation. Although the efficiency value is identical to IM over a wide speed range, the utilization rate in EVs lags far behind IM due to high torque fluctuations that lead to high noise and vibrations.
The absence of the risk of demagnetization due to temperature increase in the use of IMs in EVs, a uniform air gap, simple structures, durability, low costs, advanced speed control techniques have made it preferable to other types of motors used in EVs. IMs’ efficiency standards are set by two institutions: IEC (International Electrotechnical Commission) and NEMA (National Electrical Manufacturers Association). The high efficiency of an electric motor selected for electric vehicles means that it draws a good working curve over a wide speed range. According to the relevant standards, an IM with efficiency class IE3 has an efficiency of over 95%. The electric motor operating at low speeds has an efficiency value of 75%, and that operating at high speeds has an efficiency value of 90%, which means that the motor works efficiently [6].
However, the use of induction motors in electric vehicles has some disadvantages such as eddy-current loss at high speed, a very limited transient overload power, and high uncontrolled generator voltage.
2. The use of induction motors in electric vehicles
2.1 Dynamic equation of the EVs with IMs
The three-phase AC supply is connected to the stator winding for the purpose of establishing the rotating magnetic field. This rotating magnetic field interacts with the constant rotor conductors, and the induced current flows through the rotor conductors. The induced current creates its own magnetic field. The interaction between the rotating magnetic field and the field due to induced currents leads to unidirectional torque that provides EVs movement.
Since the movement in terrestrial EVs will be one-way, one-dimensional lateral dynamics are taken into account in the calculations. There are five forces: inertial force, longitudinal traction force, air drag, tire rolling resistance, and gravity force responsible for the movement of an EV going up or down an inclined road. In Figure 2, the mathematical expression of the traction force of the EV vehicle in relation to other forces is given. In this figure,

Figure 2.
Lateral components of a vehicle.
In Eq. (1), the mathematical expression of the traction force of the EVs in relation to other forces is given.
The aerodynamic force,
The rolling resistance varies depending on the rotational dynamics of the wheel and the relationship between the road and the surface area. The total rolling resistance is calculated by using Eq. (5). The rolling coefficient of a radial wheel typically takes a value between 0.009 and 0.015 [9].
2.2 Performance evaluation of EVs with IMs
2.2.1 Power-to-weight ratio (P/W)
In electric motors, Power-to-Weight Ratio (
The high
Figure 3 shows the
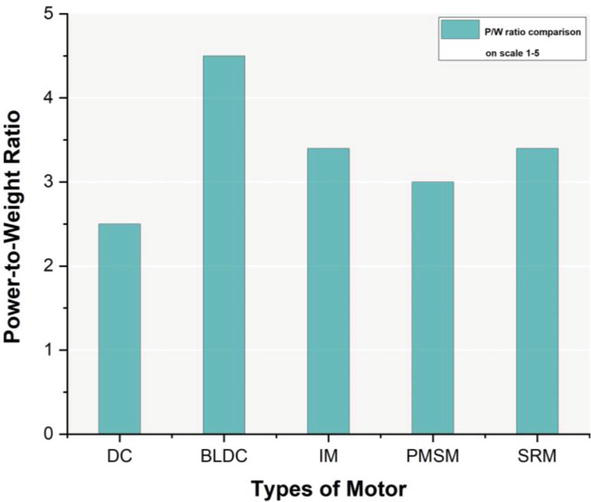
Figure 3.
2.2.2 Speed-torque characteristics of EVs with IMs
The torque-speed characteristic is a deterministic criterion in the selection of the electric motor with the most suitable performance to be used in a particular application. An ideal EV is expected to have the torque-speed characteristic given in Figure 4.
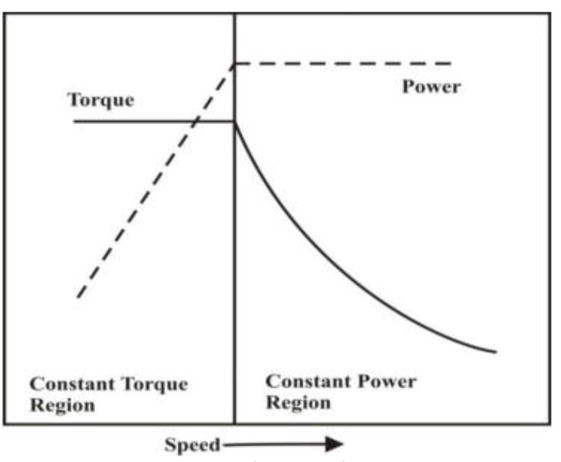
Figure 4.
Ideal EV motor speed-torque characteristics.
The ideal electric vehicle motor torque-speed curve consists of two different operating zones: a constant power zone and a fixed torque zone. This is due to the fact that the torque-speed changes are different according to the driving demands of electric vehicles. In order to provide the necessary torque in EV uses, where continuous stop/start, that is, speed needs to be constantly increased/decreased in heavy urban traffic, the motor must operate in the constant torque zone. In order to meet the demand for high speed in out-of-town use, the motor must operate in the constant power zone in areas that are not often stop-and-go areas that are expected to work.
Although DC motors provide high starting torque, the speed decreases as the torque increases. Therefore, these motors are suitable for urban EV use where there is no expectation of high speed. Similarly, it is suitable for constant speed applications in synchronous motors that are operated at synchronous speeds that vary depending on the load. Since there is no winding or permanent magnet in the rotors of the switched reluctance motors, it is possible to reach very high speeds in electric vehicles where such motors are used. However, torque fluctuation, which is one of the most important disadvantages of ARM motors, causes undesired speed fluctuations at high speeds and errors in motion control at low speeds [10].
IMs have a torque-speed characteristic very close to the mechanical characteristics expected from EVs. When the motor is loaded up to the breakdown torque, high speeds can be reached because the speed will increase as the torque increases linearly. The breakdown torque limits the value of the maximum speed or the constant power zone.
An ideal electric motor with EVs must have high efficiency, strong overload capacity, and a wide speed range in all modes of operation. The mechanical characteristics of IMs can provide stable operation in both the constant power and constant speed zones expected from EVs, which has made it stand out as the optimal type of motor [11].
2.2.3 Efficiency of EVs with IMs
Efficiency in electric motors is a magnitude that describes the amount the electrical energy at the input of the motor conversion into mechanical energy at the output. A high-efficiency motor means that it provides maximum mechanical power with less loss.
The electric motors used in EVs operate under different loading conditions. Therefore, when choosing an electric motor in EVs, the efficiency values of the motor should be taken into account under all load conditions from no-load to over load operation.
In Table 1, the efficiency values of different types of electric motors are presented comparatively. According to the relevant table, it has been concluded that asynchronous motors will exhibit an efficient working performance under different loading conditions from minimum load to maximum load in the use of electric vehicles.
Types of motor | Under maximum load | Under minimum load |
---|---|---|
DCM | 85–90 | 80–85 |
BLDC | >95 | 70–80 |
IM | >90 | >90 |
PM | >92 | 80–85 |
SRM | <95 | >90 |
Table 1.
2.2.4 Cost of EVs with IMs
EV manufacturers must choose a low-cost motor and controller if they want to produce low-cost EVs. IMs stand out in the market as low-cost and low-maintenance motors. Figure 5 shows the cost comparison of different types of motors and controllers. The data in the table confirm that IM is the optimal choice for manufacturers’ choice of low-cost motors and controllers.

Figure 5.
Cost of controller (a) and motor (b) comparison for different electric motor [
3. Conclusions
This chapter is focused on EVs with IMs.
DC motors are an ideal option for low speeds because of simple and less expensive power electronics. However, when evaluated for a wide speed range, it is not preferred to use it in EVs due to its high maintenance cost, large size, and low efficiency.
Although BLDC and PMSM have higher power-to-weight ratio and good efficiency [12], they lag behind IMs due to the cost of the rare earth element and scarcity of its supplier resource used in these type of motors.
In the EVs, SRM is used as an alternative motor type despite the rare magnet element barrier of BLDC and PMSM motors. Compared with these two motor types, ARMs with cheaper motor and control costs, reliability, and good efficiency lag behind IMs due to their high noise and vibration.
Recently, IMs are seen as the best motor choice for EVs because they are of low cost, are robust, are of less maintenance, and are inherently safe in case of inverter fault. Although the literature includes different types of motors used in electric vehicles, PMSM or IM is used in most EVs today [13, 14]. However, although PMSMs have higher torque density and better efficiency than IMs, SMSMs lag behind IMs in the market due to high magnet cost and price instability [15].
IMs only lag behind PMSM due to the space limitation where there is only space limitation such as electric rear wheel drive and “in-wheel motor” (IWM) applications [16].
References
- 1.
Jape SR, Thosar A. Comparison of electric motors for electric vehicle application. IJRET: International Journal of Research Engineering and Technology. 2017; 6 :12-17. DOI: 10.15623/ijret.2017.0609004 - 2.
Ramesh MV, Rao GS, Amarnath J, Kamakshaiah S, Jawaharlal B. Speed torque characteristics of brushless DC motor in either direction on load using ARM controller. In: Proceedings of the IEEE International Conference on Innovative Smart Grid Technologies (ISGT ‘11); 01–03 December 2011; India. Kollam: IEEE; 2012. pp. 1-6 - 3.
Takano Y, Takeno M, Hoshi N, Chiba A, Takemoto M, Ogasawara S, et al. Design and analysis of a switched reluctance motor for next generation hybrid vehicle without PM materials. In: Proceedings of the IEEE International Conference on Power Electronics (ECCE’ 10); 21–24 June 2010; Japan. Sapporo: IEEE; 2011. pp. 1801-1806 - 4.
Chen H, Gu JJ. Implementation of the three-phase switched reluctance machine system for motors and generators. IEEE/ASME Transactions on Mechatronics. 2010; 15 :421-432. DOI: 10.1109/TMECH.2009.2027901 - 5.
He C, Hao C, Qianlong W, Shaohui X, Shunyao Y. Design and control of switched reluctance motor drive for electric vehicles. In: Proceedings of the IEEE International Conference on Control, Automation, Robotics and Vision (ICARCV’ 16); 1315 November 2016; Thailand. Phuket: IEEE; 2017. pp. 1-6 - 6.
Pathak S, Prakash R. Development of high performance AC drive train. In: Proceedings of the IEEE International Conference on Electric and Hybrid Vehicles; 18–20 December 2006; India. Pune: IEEE; 2007. pp. 1-3 - 7.
Demir U, Aküner MC. Design and optimization of in-wheel asynchronous motor for electric vehicle. Journal of the Faculty of Engineering and Architecture of Gazi University. 2018; 33 :1517-1530. DOI: 10.17341/gazimmfd.416448 - 8.
Giri F, editor. AC Electric Motors Control: Advanced Design Techniques and Applications. 1st ed. United States: Wiley; 2013. p. 475. DOI: 10.1002/9781118574263.ch1 - 9.
Nam KH, editor. AC Motor Control and Electrical Vehicle Applications. 2nd ed. Boca Raton: CRC Press; 2018. p. 375. DOI: 10.1201/9781315200149 - 10.
Turhan E. Dış rotorlu fırçasız doğru akım motorlarında moment iyileştirmesi. İstanbul: İstanbul Teknik Üniversitesi; 2014 - 11.
Yue W, Dawei G. A comparison of different types of motors used for low speed electric vehicles: Experiments and simulations. In: Proceedings of the IEEE International Conference and Expo Transportation Electrification Asia-Pacific (ITEC Asia-Pacific’ 14); 31 August–03 September 2014; China. Beijing: IEEE; 2014. pp. 1-5 - 12.
Dorrell DG, Knight AM, Popescu M, Evans L, Staton DA. Comparison of different motor design drives for hybrid electric vehicles. In: Proceedings of the IEEE International Conference on Energy Conversion Congress and Exposition (ECCE’ 2010); 12–16 September 2010; USA. Atlanta: IEEE; 2010. pp. 3352-3359 - 13.
Santiago JD, Bernhoff H, Ekergård B, Eriksson S, Ferhatovic S, Waters R, et al. Electrical motor drivelines in commercial all-electric vehicles: A review. IEEE Transactions on Vehicular Technology. 2011; 61 :475-484. DOI: 10.1109/TVT.2011.2177873 - 14.
Boldea I, Tutelea LN, Parsa L, Dorrell D. Automotive electric propulsion systems with reduced or no permanent magnets: An overview. IEEE Transactions on Industrial Electronics. 2014; 61 :5696-5711. DOI: 10.1109/TIE.2014.2301754 - 15.
Cui J, Kramer M, Zhou L, Liu F, Gabay A. Current progress and future challenges in rare-earth-free permanent magnets. Acta Materialia. 2018; 158 :118-137. DOI: 10.1016/j.actamat.2018.07.049 - 16.
Márquez-Fernández F, Reinap A, Alaküla M. Design, optimization and construction of an electric motor for an electric rear wheel drive unit application for a hybrid passenger car. In: Proceedings of the IEEE International Conference on Electrical Machines (ICEM’ 2010); 06–08 September 2010; Italy. Rome: IEEE; 2010. pp. 1-6