Properties of BPA and PCP.
Abstract
Bentonite clay modified with cetyltrimethylammonium bromide (CTAB) has been investigated as an effective adsorbent for the removal of bisphenol A (BPA) and pentachlorophenol (PCP) from aqueous solutions. The adsorption performance of Bentonite-CTAB was evaluated by conducting batch adsorption experiments under different conditions. The adsorption isotherms of BPA and PCP on Bentonite-CTAB (BT-CTAB) were analyzed using the Langmuir and Freundlich models. The Langmuir model provided a better fit to the experimental data, suggesting the presence of monolayer adsorption. The adsorption kinetics of BPA and PCP on Bentonite-CTAB were studied using pseudo-first-order and pseudo-second-order models. The results indicate that the adsorption process follows pseudo-second-order kinetics, suggesting that the adsorption is controlled principally by chemisorption. Equilibrium time for both pollutants was achieved within 30–40 min. The results of adsorption studies indicated that Bentonite-CTAB exhibited excellent adsorption capacity for bisphenol A and pentachlorophenol. The high surface area and presence of active sites on Bentonite-CTAB favored adsorption of pollutants from aqueous solution. The adsorption process adopted pseudo-second-order kinetics, indicating the involvement of chemisorption. the adsorption isotherms of BPA and PCP on Bentonite-CTAB were analyzed using the Langmuir and Freundlich models. The Langmuir model provided a better fit to the data, suggesting monolayer adsorption.
Keywords
- bisphenol A
- pentachlorophenol
- bentonite
- BT-CTAB
- adsorption
1. Introduction
Adsorption, a technique commonly used to remove pollutants from contaminated water sources, including phenolic compounds, has gained in popularity. Bentonite, a clay mineral known for its high surface area and adsorption capacity, has been widely studied for its potential in adsorption processes. However, the pure form of Bentonite may have limitations in effectively adsorbing phenolic compounds, leading researchers to explore modifications to its surface properties using surfactants. Cetyltrimethylammonium bromide (CTAB), a cationic surfactant commonly used to modify clay minerals, showed promise in improving the adsorption performance of Bentonite. By enhancing hydrophobic interactions and increasing surface area, the addition of CTAB has improved the adsorption of phenolic compounds.
Recent studies have focused on the use of the Bentonite-CTAB composite for the adsorption of phenolic compounds. For example, Khenifi et al (2009) investigated removal of 2,4-dichlorophenol (2,4-DCP) from aqueous solutions by Bentonite CTAB and observed a significantly higher adsorption capacity. The improved performance was attributed to enhanced hydrophobic interactions and increased accessibility of phenolic compounds to the surface of the modified clay [1]. Similarly, Noufel et al. (2020) examined the adsorption of bisphenol A with a Bentonite-CTAB composite, reporting a substantial improvement in removal efficiency over pure Bentonite [2].
The use of modified Moroccan bentonite is highly effective in removing pollutants and improving water quality [3, 4], Bentonite used as a coagulant has a basic pH probably due to the presence of potassium feldspar and calco-sodium. Coagulant/bentonite mixtures in surface water clarification have very important consequences [5, 6, 7].
This study aims to evaluate the removal efficiency of phenolic compounds such as bisphenol A and pentachlorophenol using a Bentonite-CTAB composite. By manipulating experimental conditions and conducting batch adsorption experiments, various factors influencing the adsorption process, as well as adsorption capacity and kinetics, will be evaluated. Isothermal and kinetic models will be used to better understand the adsorption mechanism and identify the steps that control the rate. This study will provide valuable information on the performance and optimization of the Bentonite-CTAB composite for the removal of phenolic compounds.
2. Methodology
2.1 Bentonite-CTAB preparation
The synthesis procedure for the Bentonite-CTAB modification:
Activation of Bentonite: Add 50 g of bentonite sample and 12% hydrochloric acid solution (HCl) to the beaker to completely cover the bentonite and stir the mixture at 60°C for 4 h to activate. Centrifuge the mixture to separate the activated bentonite from the supernatant, discard and save the supernatant. Neutralize the activated bentonite, add 10% sodium hydroxide solution (2.5 mol/L) (NaOH) to neutralize, stir the mixture and repeat the neutralization step until the bentonite is neutral. The neutralized bentonite is washed 4–5 times alternately with deionized water and neutralized with ethanol. dry the final sample at 80°C and store it for later use.
Preparation of CTAB solution: 10 g of CTAB add it to a volume of deionized water to dissolve the CTAB while stirring, continue stirring until a homogeneous solution is obtained.
Modification of Bentonite with CTAB: Take 20 g of the activated Bentonite, add 700 mL of distilled water and stir the mixture at 25°C for 4 h to completely hydrated the bentonite, Defined volume (500 mL) of 6 g/L CTAB solution was slowly added to the above mixture at 60°C continue stirring for 4 h, wash the sample with distilled water three times to remove residual CTAB, repeat the washing step until a 0.1 mol/L silver nitrate solution,used to detect precipitation, shows no precipitation in the washing solution, dry the final modified sample at 80°C, store the modified Bentonite-CTAB for later use.
2.2 Bisphenol A (BPA) and Pentachlorophenol (PCP)
See Table 1
Proprieties | BPA | PCP |
---|---|---|
Chemical formula | C15H16O2 | C6HCl5O |
Molar mass | 228,28 g/mol | 266.35 g/mol |
Water solubility | 120–300 mg/l at pH 7 | 0.020 g/L at 30°C |
Melting point | 158°C | 190°C |
Boiling point | 250–252°C | 310°C |
Vapor pressure | 5 × 10−6 Pa (25°C) | 0.0001 mmHg (25°C) |
Table 1.
2.3 Determination of contaminant concentrations in waste water
A 10 ml sample of the pollutant was placed in a quartz cuvette and analyzed using a UV-VIS spectrophotometer. To determine the concentrations of bisphenol A (BPA) and pentachlorophenol (PCP) after each experiment, calibration curves for BPA and PCP were first set up. Six different concentrations of pure BPA and PCP were prepared, and their absorbance values were measured using a UV-Vis spectrometer at their respective wavelengths of maximum absorption (λ max = 276 nm for BPA and 320 nm for PCP). The maximum absorbance values obtained were plotted against each concentration of BPA and PCP. Based on this plot, the concentrations of BPA and PCP present in the wastewater sample were calculated.
2.4 Batch adsorption study
Batch experiments were conducted to assess the adsorption efficiency of Bentonite-CTAB for the removal of Bisphenol A (BPA) and Pentachlorophenol (PCP). A BPA and PCP solution was prepared by dissolving a specific quantity of solid in deionized water at a concentration of 1 g/L.
Adsorption experiments were performed by adding a fixed amount of 0.1 g of Bentonite-CTAB adsorbent to a series of 100 ml Erlenmeyer flasks containing 50 ml of the BPA and PCP solutions. The flasks were then stirred until equilibrium was attained. At specified time intervals, 10 ml samples were collected and filtered to eliminate any residual adsorbent present in the solution. The concentrations of the liquid samples were subsequently analyzed using a UV spectrophotometer (Rayleigh, UV-1800) at the maximum wavelength of BPA and PCP.
Several parameters were considered to study their influence on the adsorption capacity. These parameters included contact time (ranging from 10 to 120 min), initial concentration of the BPA and PCP solution (ranging from 10 to 100 ppm), pH values (ranging from 3 to 11), and temperature (ranging from 25 to 65°C).
To determine the adsorption capacity of Bentonite-CTAB for BPA and PCP, batch adsorption experiments were conducted. The concentration of BPA and PCP were determined using ultraviolet-visible spectrophotometry. The adsorption capacity (qe) of BPA and PCP on Bentonite-CTAB were calculated using equations (1):
Eq. (1) determines the equilibrium adsorption capacity (qe) of Bentonite-CTAB for the BPA and PCP solution based on the volume (V), initial and final concentrations (Ci and Cf), and the mass of the adsorbent (m).
These equations allow for the quantification of the adsorption performance of Bentonite-CTAB for BPA and PCP and facilitate the assessment of different experimental conditions on the adsorption process.
3. Discussion
3.1 Adsorption studies of Bentonite-CTAB
3.1.1 Adsorption kinetics experiments
Adsorption kinetics is an important parameter for understanding the mechanism of BPA and PCP adsorption onto the adsorbent surface. Batch adsorption versus time data using a fixed dose of adsorbent 0.1 g at 25°C were used for kinetic modeling of BPA and PCP with an initial concentration of 1000 mg/L.
Therefore, 30 min for BPA and 40 min for PCP, a contact time sufficient to reach adsorption equilibrium, was chosen for further adsorption studies. To study the adsorption kinetics, pseudo-first-order and pseudo-second-order models were applied to the experimental data in Figure 1. Correlation coefficients and adsorption kinetic parameters are summarized in Table 2. These results indicated that the adsorption of BPA and PCP on BT-CTAB can be explained by pseudo-second-order kinetics.
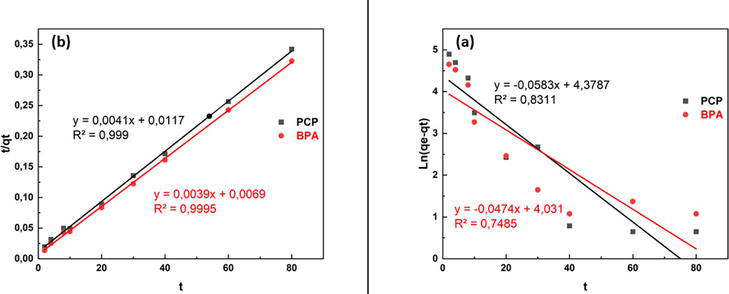
Figure 1.
Pseudo first order (a), Pseudo second order (b).
Model | Equation | Value of parameters | |||
---|---|---|---|---|---|
BPA | PCP | ||||
Pseudo-first-order | qe,cal (mg/g) k1 (min−1) R2 | 56.36 0.0002 0.74 | qe, cal (mg/g) k1 (min−1) R2 | 79.73 0.0003 0.83 | |
Pseudo-second-order | qe,cal (mg/g) k2 (g/mg/min) R2 | 256.41 0.0022 0.99 | qe, cal (mg/g) k2(g/mg/min) R2 | 243.90 0.0014 0.99 |
Table 2.
Kinetic model parameters for the adsorption process.
Contact time is an important parameter in the adsorption process. As shown in Figure 2, the adsorption of BPA and PCP on Bentonite-CTAB changes with increasing contact time. The results show that the rate of adsorption is initially high and then gradually slows down until equilibrium is reached. The adsorption capacities of BPA and PCP are very high, about 248 mg/g and 233 mg/g respectively. Adsorption equilibrium is reached in 30 min for BPA and 40 min for PCP, a study conducted by Ahari et al. in 2020 demonstrated that equilibrium sorption of chlorophenols onto geosynthetic clay liners’ geotextile was achieved after 72 h [8].
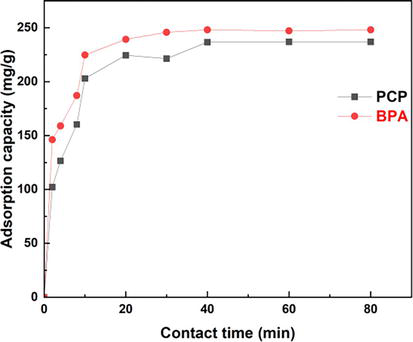
Figure 2.
Influence of contact time on adsorption of BPA and PCP.
Table 2 shows the experimental and calculated parameters for the pseudo-first-order and pseudo-second-order equations. The R2 values of the pseudo-first-order model showed no consistent trend, and the experimental qe values (qe, exp) did not agree well with the calculated values (qe, cal) obtained from the linear plots. This indicates that the resulting adsorption of the two contaminants on all adsorbents does not follow a pseudo-first-order kinetic model. However, all R2 values obtained from the pseudo-second-order model are close to 1, and the experimental q-values and the calculated values obtained from the linear plots are in good agreement. These results suggest that the second pseudo-order model better describes the experimental data. According to previous studies, the second pseudo-order model assumes that the rate-limiting step is the chemical bonding process in which electrons are shared or exchanged between adsorbent and adsorbate.
3.1.2 Adsorption isotherm experiments
Experimental equilibrium adsorption data for BPA and PCP on Bentonite-CTAB were modeled using Langmuir and Freundlich isotherms. A comparison of the experimental BPA and PCP adsorption data with the theoretical Langmuir and Freundlich isotherm plots is shown in Figure 3. Table 3 shows the optimal parameters for each model.
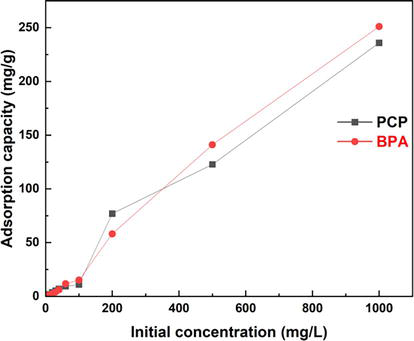
Figure 3.
Influence of initial concentration on the adsorption of BPA and PCP.
Isotherm | Equation | Value of parameters | |||
---|---|---|---|---|---|
BPA | PCP | ||||
Langmuir | qm(mg/g) KL(L/mg) RL R2 | 15.22 0.016 0.066 0.91 | qm(mg/g) KL(L/mg) RL R2 | 36.63 0.029 0.032 0.80 | |
Freundlich | KF (mg/g) n R2 | 0.014 0.247 0.85 | KF (mg/g) n R2 | 18.32 0.879 0.39 |
Table 3.
Langmuir and Freundlich constants for the adsorption of BPA and PCP onto BT-CTAB at temperature of 25°.
The experimental adsorption isotherms were compared with the theoretical models of Langmuir and Freundlich. Batch experiments were performed using 0.1 g of the adsorbent with different initial concentrations of PCP (10–1000 mg/L) under continuous stirring for a contact time of 40 min and at pH = 7. The Langmuir isotherm model is one of the most common adsorption models. It assumes monolayer coverage of the adsorbate on a homogeneous adsorbent surface; at equilibrium, a saturation point is reached where no further adsorption can occur. The essential characteristics and feasibility of the Langmuir isotherm are described in terms of a dimensionless constant separation factor (RL), which is defined as follows:
The Langmuir model was in better agreement with the experimental data than the Freundlich model for both contaminants, with R2 = 0.91 for BPA and R2 = 0.80 for PCP. Moreover, the RL is greater than zero, indicating good adsorption for both pollutants studied. This result means that pollutants can be homogeneously adsorbed by the active receptor sites on the surface of the Bentonite-CTAB adsorbent and a saturated adsorption capacity is expected due to the limited number of adsorbent sites on the surface [9]. This indicates that monolayer adsorption of the BPA and PCP occurred on surfaces that were uniform in terms of adsorption affinity. In this study, the maximum adsorption capacities for BPA and PCP resulting from the initial concentration effects were found to be 251.05 mg/g and 235.85 mg/g, respectively. Djebri et al. (2017) also studied the adsorption of an endocrine disruptor onto modified Bentonite clay. The authors identified that the Langmuir isotherm adequately fitted the adsorption data and that the maximum adsorption capacity was 127.7 mg/g for bisphenol A (Figure 4) [10].
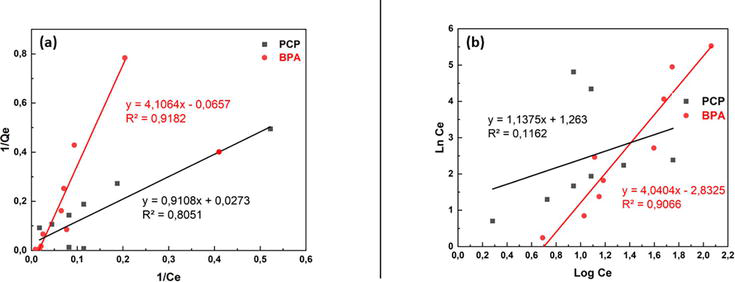
Figure 4.
Langmuir Isotherm (a); Freundlich Isotherm (b).
3.1.3 Effect of temperature and thermodynamics studies
As shown in Figure 5 The adsorption capacity of BPA and PCP decreases with increasing temperature in the range of 25–65°C. Thus, the adsorption capacity of BPA and PCP is high at low temperatures. However, at higher temperatures, a decrease in the adsorption capacity of BPA and PCP is observed. This behavior, in agreement with Arrhenius’ law, suggests that the BPA and PCP adsorption reaction, occurring on the Bentonite-CTAB surface, is exothermic, and indicates that with each increase in temperature.
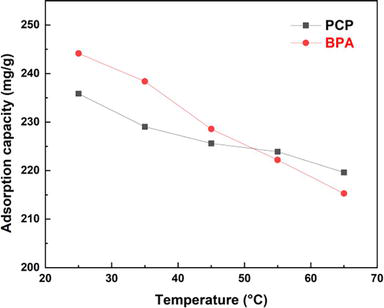
Figure 5.
Influence of temperature on the adsorption of BPA and PCP.
Table 4 shows the calculated values of the adsorption amounts
System | |||||||
---|---|---|---|---|---|---|---|
298 | 308 | 318 | 328 | 338 | |||
BPA | −40.14 | −116.36 | −5.8 | −4.19 | −2.59 | −1.89 | −1.23 |
PCP | −16.16 | −43.23 | −3.54 | −2.57 | −2.22 | −2.08 | −1.66 |
Table 4.
Thermodynamic parameters for adsorption of BPA and PCP.
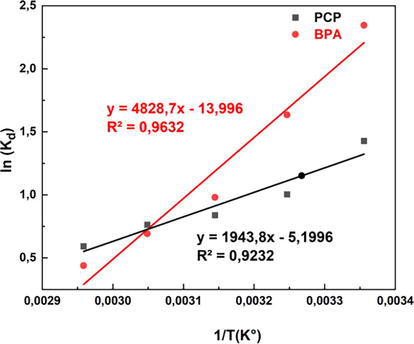
Figure 6.
Thermodynamic fitting curve of the adsorbed BPA and PCP.
3.1.4 Effect of solution pH
The initial pH of the solution plays a special role on adsorption because of its effect on the solution chemistry and the degree of ionization of the adsorbent surface. BPA and PCP removal was studied by varying the pH of the aqueous solution from 3 to 11 at 25°C with a fixed BPA and PCP concentration of 1000 mg/L and an adsorbent mass of 0.1 mg. The Figure 7 shows the adsorption capacity of BPA and PCP by Bentonite-CTAB at different pH values. As can be seen from the graph, the removal of BPA and PCP increased significantly as the pH of the starting solution was increased from 1 to 7, and then decreased from 7 to 11 as the pH increased. In this study, maximal removal of BPA and PCP was achieved at pH 7, with maximal removal of 200 mg/g for BPA and 250 mg/g for PCP.
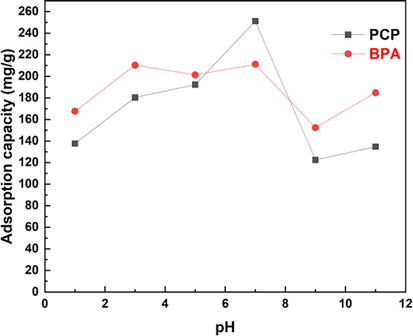
Figure 7.
Influence of pH on the adsorption of BPA and PCP.
The strong adsorption of BPA and PCP observed at pH (1–7) is due in part to the electrostatic interaction between several positively charged surface functional groups and negatively charged chlorophenolate ions for the process. BPA and PCP adsorption. As the pH moved into the more basic range, The adsorption capacity of bisphenol A (BPA) and pentachlorophenol (PCP) decreases significantly at pH values above 7. This phenomenon is due to the simultaneous decrease of negative surface charge and carbon content with increasing pH. As ionization increases and the surface becomes negatively charged, the electrostatic affinity between the adsorbent and the pollutant weakens. As a result, the electrostatic interaction weakens and the adsorption efficiency decreases. Adjustment of pH value has been proven to be a strategic regulator that influences the special physicochemical properties of pollutants and adsorbents [12].
3.1.5 Mechanism for BPA and PCP on Bentonite-CTAB
The adsorption mechanism for BPA and PCP on Bentonite-CTAB involved multiple interactions, including electrostatic attraction, and hydrogen bonding. The CTAB cations on the surface of Bentonite-CTAB provided positive charges that interacted with the negatively charged pollutants, facilitating their adsorption. Additionally, the presence of hydroxyl groups in the pollutant molecules enabled hydrogen bonding with the functional groups on the Bentonite-CTAB surface.
Initially, the active sites on the Bentonite-CTAB surface facilitate adsorption, with hydrophobic interactions likely playing a role in the initial binding of organic components from BPA and PCP. Electrostatic forces come into play as positively charged CTAB ions on the Bentonite surface interact with negatively charged functional groups on the pollutants, promoting electrostatic adsorption. Additionally, hydrogen bond formation between specific functional groups in BPA and PCP and active sites on Bentonite-CTAB enhances the adsorption process. Molecular rearrangement may occur as contaminants adjust to the surface, optimizing adsorption efficiency. Ultimately, as the active sites become saturated, adsorption reaches an equilibrium point, signaling a balance between the fixation of contaminants and their release. It’s important to recognize that this proposed mechanism simplifies a complex process, and experimental validation would be crucial to refining and confirming these interactions (Figure 8).
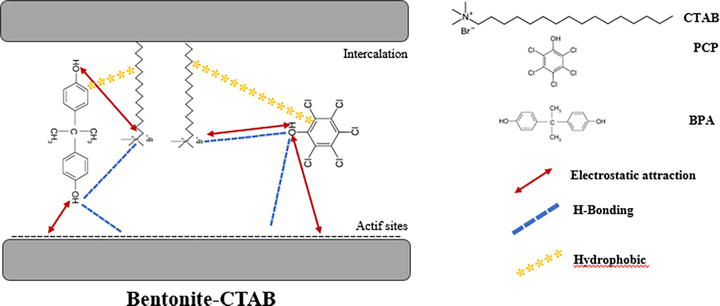
Figure 8.
Adsorption mechanism of Bentonite-CTAB on the removal of BPA and PCP.
3.1.6 Comparison of adsorption capacity
Adsorption capacity and removal efficiency of contaminants in water Bisphenol A (BPA) and pentachlorophenol (PCP) are important factors in evaluating the effectiveness of various water treatment methods. BPA is an endocrine-disrupting compound commonly found in spring water, and PCP is a toxic chlorine compound commonly resulting from industrial activities. Several studies have investigated the adsorption performance of different materials for the removal of BPA and PCP. Adsorption processes involve the attraction of contaminants to solid surfaces. Studies show that the adsorption capacity of BPA is often higher than that of PCP due to their different molecular properties and interactions with adsorbents. However, specific results depend on factors such as sorbent type, pH, and initial concentration.
Table 5 presents the results of various studies comparing the adsorption capacities of his BPA and PCP using different adsorbent materials. The results show that BPA generally has a higher adsorption capacity than PCP under similar conditions, although specific values may vary depending on the sorbent used and initial contaminant concentration.
Adsorbate | Adsorbent | qmax (mg/g) | Reference |
---|---|---|---|
PCP | Bentonite-CTAB | 243.90 | This study |
Bentonite | 10,63 | [13] | |
Magnetic recoverable carbon | 120.48 | [14] | |
Carbon nanotube hybrid polymer adsorbents | 20–100 | [15] | |
22-2-10 modified vermiculite | 85 | [16] | |
modified chitosan | 24.37 | [17] | |
BPA | Bentonite-CTAB | 256,41 | This study |
Organobentonite | 127.7 | [10] | |
Calcium alginate/organo activated bentonite (A-OAB) | 252.9 | [2] | |
Fe3O4-polyaniline | 28.1 | [18] | |
sulfur-doped titanium dioxide on magnetic bentonite (CST/γ-Fe2O3-BT) | 77.36 | [19] | |
Synthesized Chitosan (SC) | 34.48 | [20] |
Table 5.
Comparison of adsorption capacity.
4. Conclusion
The study investigated the use of Bentonite clay modified with cetyltrimethylammonium bromide (CTAB) as an effective adsorbent for the removal of bisphenol A (BPA) and pentachlorophenol (PCP) from aqueous solutions. The modified clay, Bentonite-CTAB, demonstrated high adsorption potential for both pollutants, the adsorption isotherms of BPA and PCP on Bentonite-CTAB were analyzed using the Langmuir and Freundlich models. The Langmuir model provided a better fit to the data, suggesting monolayer adsorption. The maximum adsorption capacities were determined to be 243,90 mg/g for PCP and 256,41 mg/g for BPA, indicating the significant adsorption capabilities of Bentonite-CTAB for these contaminants.
The adsorption kinetics of BPA and PCP on Bentonite-CTAB were studied using pseudo-first-order and pseudo-second-order models. The results indicated that the adsorption process followed pseudo-second-order kinetics, indicating chemisorption as the principal mechanism. Equilibrium was reached within 30 min for BPA and 40 min for PCP.
The study demonstrated that Bentonite-CTAB exhibited excellent adsorption capacity for BPA and PCP due to its high surface area and presence of active sites. The adsorption process was found to be pH-dependent, with higher adsorption capacity observed at lower pH values, attributed to increased positive charge on the surface of Bentonite-CTAB, facilitating electrostatic interactions with the pollutants. Temperature also influenced the adsorption process, with lower temperatures favoring higher adsorption capacities.
The adsorption mechanism for BPA and PCP on Bentonite-CTAB involved multiple interactions, including electrostatic attraction and hydrogen bonding. The CTAB cations on the surface of Bentonite-CTAB provided positive charges that interacted with the negatively charged pollutants, facilitating their adsorption. Additionally, the presence of hydroxyl groups in the pollutant molecules enabled hydrogen bonding with the functional groups on the Bentonite-CTAB surface.
Overall, the study highlights the effectiveness of Bentonite-CTAB as an adsorbent for the removal of BPA and PCP from aqueous solutions. The findings contribute to the understanding of the adsorption process and provide valuable insights for the development of efficient water treatment methods targeting these pollutants.
References
- 1.
Khenifi A, Zohra B, Kahina B, Houari H, Zoubir D. Removal of 2, 4-DCP from wastewater by CTAB/bentonite using one-step and two-step methods: A comparative study. Chemical Engineering Journal. 2009; 146 (3):345-354. DOI: 10.1016/j.cej.2008.06.028 - 2.
Noufel K, Djebri N, Boukhalfa N, Boutahala M, Dakhouche A. Removal of bisphenol A and trichlorophenol from aqueous solutions by adsorption with organically modified Bentonite, activated carbon composites: A comparative study in single and binary systems. Groundwater for Sustainable Development. 2020; 11 :100477. DOI: 10.1016/j.gsd.2020.100477 - 3.
Hadoudi N, Charki A, El Ouarghi H, Mourabit F, Salhi A, Amhamdi H, et al. Sorption of Bisphenol A from aqueous solutions by acid-activated bentonite clay. Desalination and Water Treatment. 2023; 285 :121-128. DOI: 10.5004/dwt.2023.29295 - 4.
Ahari M, Ddahim H, Ramadane R. Performance of bentonite clay as a coagulation aid on water quality. Desalination and Water Treatment. 2019; 143 :229-234. DOI: 10.5004/dwt.2019.23552 - 5.
Zaki N, Hadoudi N, Charki A, Bensitel N, Ouarghi H. E, Amhamdi H, Ahari M. H. Advancements in the chemical treatment of potable water and industrial wastewater using the coagulation–flocculation process. Separation Science and Technology. 2023; 58 :1-12. DOI: 10.1080/01496395.2023.2219381 - 6.
Charki A, El Ouarghi H, Ahari M. Synthesis of Leachate from the Al Hoceima Controlled Landfill and Characterization (Morocco, North of Africa). Moroccan. Journal of Chemistry. 2022; 10 (4):800-807. DOI: 10.48317/IMIST.PRSM/morjchem-v10i3.30231 - 7.
Charki A, El Ouarghi H, Ahari M. Treatability tests of synthetic leachate from the Al-Hoceima City Controlled Landfill. E3S Web of Conferences. EDP Sciences. 2021:07007. DOI: 10.1051/e3sconf/202131407007 - 8.
Ahari MH, Touze-Foltz N, Mazéas L. Sorption of Chlorophenols on Geotextile of the Geosynthetic Clay Liners. Environmental Engineering Research. 2020; 25 (2):163-170. DOI: 10.4491/eer.2019.004 - 9.
Hadoudi N, Charki A, El Ouarghi H, Mourabit F, Salhi A, Amhamdi H. Sorption of Pentachlorophenol from Aqueous Solution by Acid Activated Bentonite: A comparative study. Egyptian Journal of Chemistry. 2023; 66 (6):353-361. DOI: 10.21608/ejchem.2022.143001.6255 - 10.
Djebri N, Boutahala M, Chelali N. E, Boukhalfa N, Larbi Z. Adsorption of bisphenol A and 2, 4, 5-trichlorophenol onto organo-acid-activated bentonite from aqueous solutions in single and binary systems. Desalination and Water Treatment 2017;66:383-393. DOI: 10.5004/dwt.2017.20220 - 11.
Zhou L, Pan S, Chen X, Zhao Y, Zou B, Jin M. Kinetics and thermodynamics studies of pentachlorophenol adsorption on covalently functionalized Fe3O4@ SiO2–MWCNTs core–shell magnetic microspheres. Chemical Engineering Journal. 2014; 257 :10-19. DOI: 10.1016/j.cej.2014.07.060 - 12.
Hameed BH, Tan IAW, Ahmad AL. Adsorption isotherm, kinetic modeling and mechanism of 2, 4, 6-trichlorophenol on coconut husk-based activated carbon. Chemical Engineering Journal. 2008; 144 (2):235-244. DOI: 10.1016/j.cej.2008.01.028 - 13.
Kakavandi B, Jahangiri-rad M, Rafiee M, Esfahani AR, Babaei AA. Development of response surface methodology for optimization of phenol and p-chlorophenol adsorption on magnetic recoverable carbon. Microporous and Mesoporous Materials. 2016; 231 :192-206. DOI: 10.1016/j.micromeso.2016.05.033 - 14.
Xu L, Wang Z, Ye S, Sui X. Removal of p-chlorophenol from aqueous solutions by carbon nanotube hybrid polymer adsorbents. Chemical Engineering Research and Design. 2017; 123 :76-83. DOI: 10.1016/j.cherd.2017.04.034 - 15.
Koumanova B, Peeva-Antova P. Adsorption of p-chlorophenol from aqueous solutions on bentonite and perlite. Journal of Hazardous Materials. 2002; 90 (3):229-234. DOI: 10.1016/S0304-3894(01)00365-X - 16.
Ji Y, Zhong H, Chen P, Xu X, Wang Y, Wang H, et al. Single and simultaneous adsorption of methyl orange and p-chlorophenol on organo-vermiculites modified by an asymmetric gemini surfactant. Colloids and Surfaces A: Physicochemical and Engineering. 2019; 580 :123740. DOI: 10.1016/j.colsurfa.2019.123740 - 17.
Shankar A, Kongot M, Saini V. K., Kumar A. Removal of pentachlorophenol pesticide from aqueous solutions using modified chitosan. Arabian Journal of Chemistry 2020;13(1):1821-1830. DOI : 10.1016/j.arabjc.2018.01.016 - 18.
Zhou Q , Wang Y, Xiao J, Fan H. Adsorption and removal of bisphenol A, α-naphthol and β-naphthol from aqueous solution by Fe3O4@ polyaniline core–shell nanomaterials. Synthetic Metals. 2016; 212 :113-122. DOI: 10.1016/j.synthmet.2015.12.008 - 19.
Yang Q , Gao M, Luo Z, Yang S. Enhanced removal of bisphenol A from aqueous solution by organo-montmorillonites modified with novel Gemini pyridinium surfactants containing long alkyl chain. Chemical Engineering Journal. 2016; 285 :27-38. DOI: 10.1016/j.cej.2015.09.114 - 20.
Hadoudi N, Amhamdi H, Ahari MH. Sorption of bisphenol A from aqueous solutions using natural adsorbents: Isotherm, kinetic and effect of temperature. E3S Web of Conferences. EDP Sciences. 2021; 314 :07003. DOI: 10.1051/e3sconf/202131407003