Recommendations on patients with suspected or established carotid artery disease according to the guidelines on non-cardiac surgery provided by the European Society of Cardiology (ESC) and the European Society of Anaesthesiology (ESA).
Abstract
This book chapter provides neuropsychiatric morbidities related to perioperative patient care including both surgeries under general or regional anaesthesia and postoperative intensive care. While detailed guidelines have been already developed for the perioperative care for patients with cardiac comorbidities, guidelines for patients with pre-existing neuropsychiatric morbidities are currently limited. In particular, these limitations may affect non-cardiac surgical and non-neurosurgical patients, which is why it seems important to develop treatment guidelines which are applicable to patients undergoing major general surgeries under general or regional anaesthesia including postoperative intensive care. Hence—although provided neuropsychiatric aspects and morbidities related to anaesthesiologic perioperative patient care are applicable to all surgical disciplines—the provided treatment recommendations primarily apply to patients undergoing general surgeries.
Keywords
- neuropsychiatric morbidities
- perioperative anaesthesiologic care
- non-cardiac surgical patients
- postoperative intensive care
- perioperative treatment recommendations
1. Introduction
This book chapter initially describes important neuropsychiatric morbidities primarily in general surgical patients including delirium, postoperative cognitive dysfunction (POCD), postoperative posttraumatic stress disorder (PTSD), perioperative stroke, convulsive status epilepticus (CSE) and non-convulsive status epilepticus (NCSE), cerebral macro- and microangiopathy, Alzheimer’s disease (AD), cerebral autoregulation dysfunction and limited cognitive reserve (CR). Subsequently, identifying perioperative patients with underlying neuropsychiatric morbidities is specified. Finally, treatment recommendations for perioperative anaesthesiologic care of non-cardiac surgical patients with neuropsychiatric morbidities are described including both surgeries under general or regional anaesthesia and postoperative intensive care.
2. Neuropsychiatric morbidities in patients undergoing non-cardiac surgeries
2.1 Potential difficulties in the perioperative care of non-cardiac surgical patients
While anaesthesiologists usually have based on evidence-based guidelines an explicit concept for patients with pre-existing cardiac morbidities, potentially limited guidelines for patients with pre-existing neuropsychiatric morbidities may complicate perioperative anaesthesiologic care [1]. Concerning neuropsychiatric morbidities, degenerative cerebral morbidities based on cerebral macro- and microangiopathy may play the most significant role due to their high prevalence in general surgical patients. These patients frequently have a reduced cerebral perfusion, a disturbed cerebral autoregulation and an impaired carbon dioxide (CO2) reactivity [2].
In Europe around 19 million of patients undergo mid-sized or major surgeries—worldwide around 200 million of patients. Perioperative complication rates are about 7–11%, with a mortality rate of about 0.8–1.5%, whereby cardiac complications show the highest frequency (about 42%) [3]. While cardiac complications have been exactly investigated, the pathophysiology underlying neuropsychiatric complications is currently poorly explored—supposing a multifactorial pathophysiology including a potentially surgically induced systemic inflammatory response syndrome (SIRS) with subsequent neuroinflammation [4, 5, 6].
2.2 Postoperative delirium
The incidence of postoperative delirium varies within a wide range from 5 to 65% [5]. Since delirium significantly increases perioperative morbidity, mortality and the duration of hospital stay [7], it is important for anaesthesiologists to know specific risk factors for postoperative delirium. Firstly, predisposing risk factors include a high patient age, hypertension, alcohol- and nicotine abuse, sleep disturbances, vision and hearing disorders, sensory disturbance, pain, limited exposition to daylight, infections, physical immobilisation, social isolation, abstinence syndrome, dehydration, electrolyte and acid-base imbalance, metabolic disorders, seizures and critical illness requiring postoperative intensive care [8]. In addition, a pre-existing cerebral impairment—in particular, dementia—seems to be a major risk factor [7, 9]. Obviously, some of these pre-existing risk factors may be also applicable to intra- and postoperative patient care. Secondly, specific risk factors related to surgeries and perioperative anaesthesiologic care are polytrauma, emergency surgeries, medically induced coma, mechanical ventilation, high blood loss, blood transfusion, hypoxaemia and temperature fluctuations [6, 8]. Finally, considering postoperative intensive care, treatment with extracorporeal circulations—in particular venoarterial extracorporeal membrane oxygenation (ECMO)—may tend to increase the incidence of overall neuropsychiatric morbidities including postoperative delirium [10].
The cardinal symptoms of delirium are spatial and temporal disorientation and impaired attention. Since postoperative patients regularly may be hypoactive and lethargic—and these symptoms could be assigned to a preceding general anaesthesia, dementia or depression—especially a hypoactive delirium remains undetected at times [7]. In particular regarding postoperative intensive care, although clinical tests for delirium are currently increasing, a relatively high number of undetected postoperative delirium probably exist [6].
The assumed pathophysiology underlying postoperative delirium is primarily a cerebral neuroinflammation impairing the blood-brain barrier (BBB) based on a SIRS potentially induced by surgical stress. According to current knowledge, a multifactorial pathophysiology seems probable [6]. Concerning that up to 50% of patients with postoperative sepsis have delirium, microvascular alterations, metabolic disturbances and impaired aerobic processes probably contribute significantly to the multifactorial pathophysiology [11]. Moreover, significant intraoperative blood pressure fluctuations are associated with delirium, while the role of intraoperative hypotensive time periods remains a bit unclear [12]. Whereas specific medications used in the perioperative period (e.g. benzodiazepines, opiates, propofol, glucocorticoids and atropine) may promote delirium, dexmedetomidine seems being protective [8]. However, whether using regional anaesthesia instead of general anaesthesia to reduce delirium-associated medications decreases the occurrence of postoperative delirium remains currently unproven—one reason for this might be the complex pathophysiology [13]. The latest research investigates postoperative blood pressure regulation—suggesting that particularly postoperative hypotensive periods contribute to delirium and overall patient morbidity and mortality [14, 15].
2.3 Postoperative cognitive dysfunction (POCD)
POCD is characterised as a subtle decline in patient’s cognitive ability from its preoperative baseline including the following specific cognitive domains: learning and memory, language, perceptual-motor, social cognition, complex attention and executive function. In contrast to immediate cognitive impairment potentially related to multiple causes (e.g. recovery from anaesthesia or surgery, pain, anxiety and sleep disturbance), POCD onset starts several days later and may last for months or even years. POCD can significantly affect the quality of life, potentially outweighing the surgical benefits, which has more serious sequelae in the elderly population. In addition, POCD is associated with a higher postoperative 1-year mortality [16]. Finally, in particular, the elderly population should be perioperatively examined by multiple psychometric tests for POCD [17].
POCD occurs most often after cardiac (up to 80%), orthopaedic (up to 60%) and major general surgeries (up to 50%) [16, 18]. Specifically, after non-cardiac surgeries, the occurrence of POCD in patients with advanced age lies after 1 week, about 30%, after 3 months, about 13% and after 1 year, about 1%. Primary risk factors for POCD include a high age, a low educational level, pre-existing cerebrovascular or cardiovascular diseases and cognitive dysfunction at hospital discharge. Further risk factors may be a pre-existing cerebral impairment, prolonged anaesthesia and surgery, revision surgeries, postoperative infections and respiratory complications [16, 17].
The pathophysiology underlying POCD is currently not completely understood—a cerebral neuroinflammation potentially due to surgical stress-inducing cholinergic dysfunction and neuronal death seems to play a key role [17]. In addition, the exposition to cerebral microemboli—gaseous or solid—may contribute to POCD [18]. Cerebral microemboli exposition may occur in non-cardiac surgical patients primarily during major orthopaedic surgeries [18] and postoperative intensive care, if patients are treated with extracorporeal circuits—in particular, venoarterial ECMO may generate a high load of cerebral microemboli (Figure 1) [10].
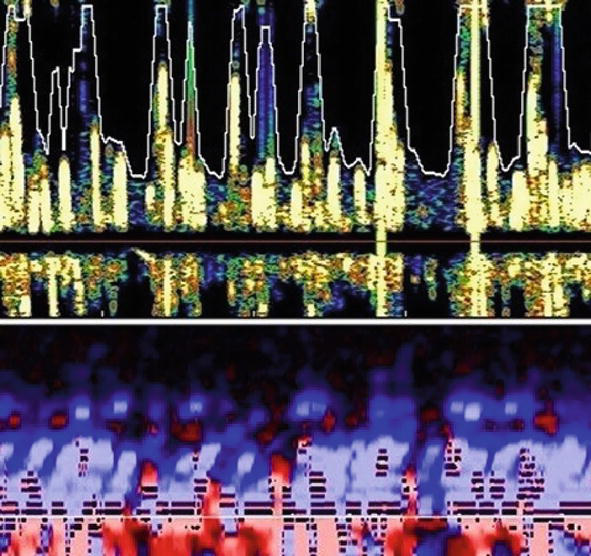
Figure 1.
Cerebral microemboli during venoarterial extracorporeal membrane oxygenation. The yellow spots represent a high load of cerebral microemboli in the middle cerebral artery using transcranial Doppler ultrasound.
Clinicians often believe that regional anaesthesia may reduce the incidence of POCD compared to general anaesthesia. However, current research could not prove a reduction of POCD using regional anaesthesia—in particular considering prolonged POCD [16]. While electroencephalography (EEG) guided total intravenous anaesthesia (TIVA) based on propofol seems being protective, clinical variables including hypoxia, hypotension and impaired cerebral perfusion could not be certainly associated with POCD [17]. Due to neuroprotective effects, dexmedetomidine is suggested to reduce POCD, whereas the potential benefits of ketamine and non-steroidal anti-inflammatory medications are currently unclear [8, 17]. Finally, keeping the postoperative hospital stay as short as possible consecutively returning the patient to everyday life may decrease a prolonged POCD [16].
2.4 Postoperative posttraumatic stress disorder (PTSD)
PTSD is generally characterised by behavioural avoidance, negative thoughts or mood and impaired physical or emotional reactions. Concerning young children (≤6 years), clinical symptoms may additionally include simulating the traumatic event during playing and frightening dreams commonly related to the traumatic event. In addition, symptoms may be aggravated by disturbed sleep patterns, frequent flashbacks, panic attacks and suicidal thoughts. The onset of postoperative PTSD may start within 1 month after surgery and may last for years—the intensity of symptoms typically varies related to peoples’ baseline stress level or coming across the event. The quality of life may be significantly impaired by social withdrawal, disability to carry out work or normal daily tasks and worse relationships [19, 20, 21].
In non-cardiac surgical patients, PTSD occurs frequently after major general surgeries (4–53%). The following perioperative risk factors for PTSD could be identified: intraoperative awareness (>2 minutes), postoperative intensive care, delirium, sepsis and SIRS [22, 23]. While most pre-existing neuropsychiatric morbidities increase the occurrence of postoperative PTSD, preoperative depression seems having protective effects [21]. Referring to benzodiazepines, intraoperative usage is protective due to their amnestic effects reducing intraoperative awareness [22], whereas benzodiazepines during postoperative intensive care may trigger PTSD [19]. According to current knowledge, a cerebral neuroinflammation potentially based on a SIRS may play a key role in the pathophysiology of PTSD [21].
The diagnosis of postoperative PTSD should consist of a physical examination and a psychiatric evaluation 2–6 hours, 2–36 hours and 30 days after surgery to identify also a prolonged onset of PTSD—using the criteria in the fifth edition of the Diagnostic and Statistical Manual of Mental Disorders (DSM-5) [24, 25]. Magnetic resonance imaging (MRI) research showed in PTSD patients reduced volumes of the hippocampus, the amygdala and the anterior cingulum [26].
The multifactorial treatment of PTSD currently prefers fast recovery concepts [27]. Most important is psychotherapy including cognitive and behavioural therapy. In addition, medical therapy consists of antidepressants (e.g. selective serotonin reuptake inhibitors, sertraline and paroxetine), anti-anxiety medications and prazosin in case of nightmares. A further application of anti-inflammatory drugs might be a promising option in the future. Moreover, treatment includes therapeutic exercise and nutritional therapy. Finally, eye movement desensitisation and reprocessing (EMDR) represent an additional modern therapy [27, 28].
2.5 Perioperative stroke
Perioperative stroke with clinical symptoms is a rare complication of general surgeries during the operation and postoperatively within 30 days (0.08–0.7%). However, since perioperative stroke is associated with a high mortality rate (18–26%) exceeding the mortality rate of strokes in the general population, it ranks among the most important neuropsychiatric perioperative complications [29, 30]. In contrast, perioperative silent strokes—strokes without any noticeable symptoms—have an incidence of up to 7% [31]. They typically affect a small part of the brain which is not necessary for vital functions. Hence, perioperative silent strokes regularly remain clinically undetected, but they can be diagnosed by MRI.
Risk factors for perioperative strokes in non-cardiac surgical patients are a high age, a pre-existing stroke or transient ischaemic attack (TIA), myocardial infarction within 6 months before surgery, atrial fibrillation, hypertension, hypercholesterolaemia, nicotine abuse, acute renal failure, dialysis and chronic obstructive pulmonary disease (COPD) [30].
Perioperative stroke is defined as brain infarction of ischaemic or haemorrhagic aetiology occurring during or within 30 days after surgery [32]. The majority is of ischaemic origin primarily based on either cardioembolisms - caused by atrial fibrillation or right-to-left shunt - or on embollisms due to vulnerable atherosclerosis of the aortic arch. Further ischaemic reasons include cerebral hypoperfusion, cerebral artery thrombosis and combined aetiologies. Although intraoperative hypotension may be less frequently associated with stroke than previously assumed, the perioperative ischaemic evaluation (POISE) trial showed a positive correlation between beta-blocker-induced intraoperative hypotension or bradycardia and perioperative stroke in non-cardiac surgical patients [33]. A further reason adding to perioperative stroke might be a disturbed cerebral autoregulation [34]. Finally—according to the NeuroVISION trial—silent strokes may primarily increase the risk for POCD [31].
2.6 Convulsive status epilepticus (CSE) and non-convulsive status epilepticus (NCSE)
CSE and NCSE have a little higher prevalence in general surgical patients than previously assumed (1–10%). During postoperative intensive care, the prevalence of NCSE further increases up to 30% [35]. Primary predisposing risk factors are structural brain diseases and impaired brain development. A CSE is defined as seizure lasting >30 minutes—importantly, seizures lasting >5 minutes are rarely self-limiting, requiring adequate treatment [36].
The pathophysiology underlying seizures is based on a disbalance of excitatory and inhibitory neuronal brain activity. As a consequence, brain metabolism, cerebral blood flow, blood glucose and lactate levels increase. Consecutively, an elevated cardiac output, tachycardia, hypertension and elevated central venous pressure occur. These pathophysiological changes may represent a compensation of the body potentially protecting the brain for 30–60 minutes. Afterwards, cerebral autoregulation is impaired—leading to cerebral hypoxia, hypoglycaemia, elevated intracranial pressure (ICP) and cerebral oedema. Finally, electrolyte imbalance, metabolic acidosis, disseminated intravascular coagulation and rhabdomyolysis may induce a multi-organ failure [36]. These pathophysiological changes likely apply to NCSE—typically proceeding slower [36, 37].
To prevent perioperative seizures, it is key continuing anti-epileptic medication. While most anaesthetics do not interfere with anti-epileptic medication, quantifying its plasma level in case of postoperative intensive care is recommended [35].
2.7 Cerebral macroangiopathy
Pathologies of cerebral arteries have significant clinical importance—in particular during anaesthesiologic perioperative patient care. Pre-existing cerebral macroangiopathy including a stroke or TIA, a significant carotid artery stenosis and a cerebral artery aneurysm may require a neurological council and subsequently, in individual patients, further diagnostic imaging such as computed tomography (CT) scan, MRI or transcranial Doppler (TCD) ultrasound. Importantly, a pre-existing peripheral artery occlusive disease (PAOD) is regularly combined with an occlusive cerebrovascular disease—potentially significantly affecting cerebral circulation. Among PAOD surgical patients with occlusive cerebrovascular disease, up to 14% have carotid artery stenosis >70% [3]. Hence, a preoperative carotid ultrasound may be a beneficial diagnostic tool to indicate a necessary cerebral revascularisation [38].
Currently, cerebral revascularisation—performed either surgically or by endovascular techniques—is usually done postoperatively as long-term prevention of stroke. Therefore, optimising cardiac output and maintaining adequate cerebral perfusion pressure (CPP) seem crucial. Considering patients with significant atherosclerosis, statins and beta-blockers should be perioperatively applied continuously, and the application of acetylsalicylic acid should be interrupted as short as possible. In addition, blood pressure levels should be targeted according to the guidelines on non-cardiac surgery provided by the European Society of Cardiology (ESC) and the European Society of Anaesthesiology (ESA) (Table 1) [3]. Finally, perioperative monitoring may be extended by invasive blood pressure monitoring and transoesophageal echo estimating stroke volume.
Recommendations | Class of recommendation | Level of evidence |
---|---|---|
Preoperative carotid artery and cerebral imaging are recommended in patients with a history of TIA or stroke in the preceding 6 months. | I | C |
Preoperative, routine carotid artery imaging may be considered in patients undergoing vascular surgery. | IIb | C |
Whenever possible, continuation of anti-platelet and statin therapies should be considered throughout the perioperative phase in patients with carotid artery disease. | IIa | C |
For patients with carotid artery disease undergoing non-cardiac surgery, the same indications for carotid revascularisation should apply as for the general population. | IIa | C |
Preoperative routine carotid artery imaging is not recommended in patients undergoing non-vascular surgery. | III | C |
Table 1.
2.8 Cerebral microangiopathy and Alzheimer’s disease (AD)
Cerebral microangiopathies—diseases of the terminal vessels—potentially impair significantly cerebral microcirculation and play a key role in the pathophysiology of perioperative neuropsychiatric morbidities. Cerebral microangiopathy primarily includes subcortical arteriosclerotic encephalopathy (Binswanger’s disease), cerebral amyloid angiopathy and inflammatory-based microangiopathies [39].
Binswanger’s disease can be clinically characterised by persistent hypertension and systemic vascular disease, acute strokes, subacute accumulation of focal neurologic symptoms with signs over weeks to months, long plateau periods, lengthy clinical course, dementia, prominent motor signs or pseudobulbar palsy and hydrocephalus. Pathologically, cerebrovascular atherosclerosis of small vessels, a diffuse white matter loss combined with gliosis and lacunar infarcts, can be detected. According to the findings of neuroimaging studies using MRI and CT scans, the term white matter disease has been established [39, 40, 41]. While it has been hypothesised that white matter hyperintensities (WMH) may result from degenerative or demyelinating processes, atherosclerotic vascular changes seem to be crucial for the progression of WMH [42].
Risk factors for Binswanger’s disease include in particular hypertension, atrial fibrillation and a high patient age [39, 42]. Accordingly, early treatment of hypertension may have a protective effect. However, hypotension (generally, orthostatically, nightly and postprandially) seems to be an additional risk factor. Therefore, blood pressure adjustment to a high-normal range (i.e. systolic blood pressure 135–150 mmHg) is currently recommended [43].
According to the current concept of mixed dementia, Binswanger’s disease promotes AD in the elderly population [44]. Pathophysiologically, AD onset is based on the accumulation of amyloid β inducing hyperphosphorylation of protein tau, leading to neurofibrillary tangles. Heritable factors play a key role in 60–80% of AD patients—more than 40 AD-associated genetic risk loci have already been identified, of which apolipoprotein E alleles have the strongest impact. Positron emission tomography scans and plasma assays may be used to detect amyloid β and phosphorylated tau. Current research further suggests that specific lifestyle factors may contribute to a positive outcome in AD patients. Prospectively, potentially beneficial medical treatment includes anti-amyloid β, anti-tau and anti-inflammatory strategies [45]. Finally, AD (60%) and Binswanger’s disease (20%) are the major causes of brain failure in the elderly population [40].
2.9 Cerebral autoregulation dysfunction
Cerebral autoregulation describes the physiological adaption of the cross-section of cerebral arterial vessels to the CPP [46]—thereby enabling a constant cerebral perfusion within a particular range of systemic blood pressure. Deceeding the lower limit of cerebral autoregulation (LLA) decreases cerebral perfusion, potentially impairing cerebral function and resulting in symptoms like seizures and neurocognitive dysfunction. In contrast, exceeding the upper limit of cerebral autoregulation (ULA) subsequently leads to cerebral hyperperfusion, potentially impairing the BBB with consecutive vasogenic cerebral oedema. Generally, cerebral autoregulation is probably controlled by myogenic and endothelial cerebrovascular factors and by metabolic mechanisms [47].
Since the Kety-Schmidt technique has been established in 1948, static cerebral autoregulation could be measured—describing the relationship between mean arterial pressure (MAP) and CPP during a steady-state MAP. Subsequently, Rune Aaslid has established TCD ultrasound in 1982, which had a key impact on measuring dynamic cerebral autoregulation—describing the MAP-CPP relationship during a non-steady-state MAP by his landmark study in 1989 [48]. Since systemic blood pressure slightly varies continuously (e.g. influenced by breathing or physiologically), nowadays, using dynamic cerebral autoregulation is paramount [49].
According to current knowledge, cerebral autoregulation functions correctly within a smaller range of systemic arterial blood pressure than previously assumed [46]—which has been firstly shown in 1997 and later confirmed (Figure 2) [47, 49]. Both the LLA seems to vary individually within 40–90 mmHg and the shape of the LLA may strongly vary. In addition, the plateau of the cerebral autoregulation curve (Figure 2) may be rather small—about 20 mmHg [47]. Importantly, various patients can probably better compensate exceeding the ULA than deceeding the LLA [49]. Accordingly, adjusting blood pressure individually during perioperative anaesthesiologic patient care seems to play a key role [47].
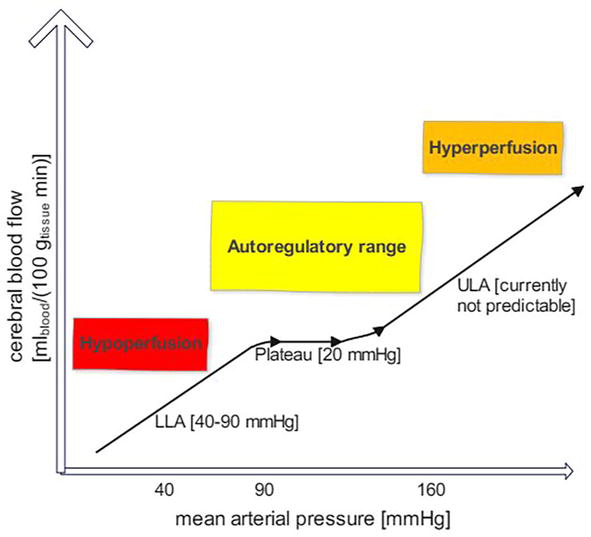
Figure 2.
Adjusted curve of cerebral autoregulation. Both the lower limit (LLA) and upper limit (ULA) of cerebral autoregulation vary significantly between individual surgical patients.
Dynamic cerebral autoregulation can be studied on one side by time domain analysis: by measuring the degree of correlation between blood pressure and various cerebral output signals, the Pearson correlation coefficient is calculated. While a positive correlation coefficient reflects synchrony between the two signals—suggesting an impaired cerebral autoregulation—a negative or near-zero correlation coefficient implies a physiological adaption to blood pressure changes, indicating an intact cerebral autoregulation. A further measuring approach is based on more complex analyses of time-frequency domains [50]. Currently, dynamic cerebral autoregulation is usually determined by two input signals—invasive or non-invasive arterial blood pressure combined with cerebral blood flow (e.g. estimated by TCD ultrasound), ICP or regional oxygen saturation (rSO2) of the brain estimated by near-infrared spectroscopy (NIRS) [51].
According to current research, cerebral autoregulation may be in particular impaired in elderly patients (e.g. based on micro- or macroangiopathy, diabetes and dementia) and perioperatively (e.g. based on mechanical ventilation, delirium, SIRS and dialysis)—indicating that a perioperative bedside monitoring might be beneficial [47]. In line with an international consensus paper regarding perioperative intensive care, monitoring of cerebral autoregulation is especially recommended for individually selected neurocritical care patients [52]. Currently, intraoperative monitoring of cerebral autoregulation cannot be routinely performed; however, it can be done in clinical research.
2.10 Limited cognitive reserve (CR)
CR is the capacity of the brain to compensate for a varying range of cerebral injuries without functional cerebral impairment—this concept has been firstly described at the end of the 80s [53]. CR is primarily based on good cerebral functionality and brain plasticity, which enables the brain to make neurostructural changes for adaption to potential impairments.
Since CR represents a theoretical concept, it is usually measured by proxy variables including general lifetime experiences, educational and occupational attainment, intelligence, lifestyle (e.g. social, physical and cognitive activities), socioeconomic status and early life experiences (e.g. perinatal and postnatal factors such as childhood intelligence). In addition, biomarker studies examining amyloid and tau protein may be used. The concept of CR—potentially playing a significant role in age-related cognitive dysfunction based on describing individual differences in susceptibility to cognitive, functional or clinical decline—may be applicable to multiple neuropsychiatric morbidities including AD, macro- and microangiopathy, delirium and other [54, 55]. Since only about 50% of inter-individual variability in cognitive dysfunction is based on the most common age-related neuropathology, such as AD, various neurodegenerative and cerebrovascular factors may significantly add to the pathophysiology underlying cognitive dysfunction in the growing elderly population [56]. Finally, there is strong evidence that a high level of CR proxy variables is associated with a lower risk of mild cognitive dysfunction and dementia in the elderly [54].
Current research suggests that specific features such as education, genetic factors, physical activity, older age at retirement and wealth increase CR, allowing individuals being more resilient by influencing the efficiency, capacity or flexibility of brain networks, cerebral vasculature, cerebral metabolism and neurochemical transmission—and in this way better coping with age-related brain diseases. On the contrary, specific features including smoking, obesity, genetics, an early onset or elevated rate of cerebral pathology, early life hardship (e.g. the death of a parent) and prenatal factors (e.g. small birth weight) may decrease CR—thus depressing the threshold of cerebral compensation of negative effects on brain health [54, 57, 58, 59, 60]. Latest research indicates that some cerebral mechanisms elevating the threshold at which cerebral compensation works seem to be similar to cerebral mechanisms in young adults managing highly challenging cognitive and behavioural tasks. Moreover, less age-related anatomic cerebral changes like brain atrophy, limited task-related networks and WMH, together with a low cerebral pathology accumulation over time, may increase CR [61]. Finally, in some elderly individuals, the anatomic or physiological cerebral processes involved in cognition may improve above current levels, which at least may attenuate typical age-related brain changes [54, 62].
According to Stern’s theory, a high level of CR may have a beneficial influence on perioperative neuropsychiatric patient outcome in two ways—by a higher preoperative level of cognitive performance and by a delayed onset of an induced perioperative cognitive decline. However, since a high level of CR may be associated with a better compensation of cerebral pathology accumulation, a high level of CR may be paradoxically associated with a faster rate of perioperative cognitive decline once cerebral injuries are severe enough to impair cognitive function [57]. This points out the potential benefits of future preoperative baseline and perioperative follow-up diagnosis of CR.
3. Identifying perioperative patients with pre-existing neuropsychiatric morbidities
3.1 Specific methods to identify pre-existing neuropsychiatric morbidities
Identifying neuropsychiatric morbidities is primarily based on exploring the central nervous system (CNS) and surrounding structures including the vasculature of the CNS and the peripheral nervous system. Related to perioperative anaesthesiologic patient care, the detection of pre-existing neuropsychiatric morbidities is of major importance. In particular, exploring exactly the preoperative anamnesis and a detailed clinical examination, ideally including psychometric test batteries should be performed. In addition, neuroimaging tools including an ultrasound of the carotid arteries, TCD ultrasound, CT scan, MRI, NIRS and EEG may be useful in individual patients.
3.2 Preoperative anamnesis
As part of the preoperative anamnesis neuropsychiatric morbidities including seizures, paralysis and sensibility disorders potentially based on a pre-existing stroke or TIA, dementia, cerebral macro- or microangiopathy, depression, anxiety disorders and more subtle cerebral impairments such as mild cognitive dysfunction should be explored. Since cerebral macro- and microangiopathy elevate the risk for perioperative neuropsychiatric disorders including disturbed cerebral autoregulation, and may be associated with potentially existing angiopathies such as coronary heart disease and PAOD and their underlying risk factors—hypertension, hypercholesterolaemia, diabetes and cigarette smoking—these facts should be considered [63]. A drug-abusing behaviour (e.g. alcohol, nicotine, drug and medical abuse) should be further explored. Finally, asking for family anamnesis may allow for detecting preoperative neurological morbidities which are partly associated with genes (e.g. multiple sclerosis (MS), dementia, seizures, migraine and stroke).
3.3 Neuropsychiatric testing and the postoperative quality of recovery scale (PostopQRS)
The Framingham Heart Study discovered that cognitive dysfunction is associated with higher patient morbidity and mortality [63]. This finding might be applicable to perioperative anaesthesiologic patient care, as suggested by research investigating non-cardiac surgical patients [64], which is why a preoperative evaluation of cognitive function seems to be beneficial. However, a complete neuropsychiatric examination including multiple psychometric tests would last about 60–120 minutes, and the specific types of tests usually vary depending on the specific hospital. The latter along with inconclusive research results underpin the difficulty of testing for cognitive dysfunction during the perioperative period. In addition, perioperative neuropsychiatric testing may be further complicated by various other factors (e.g. restless surroundings, pain and sleep disturbances).
The PostopQRS is a simple web-based digital tool to assess clinical outcomes in surgical patients related to the quality of postoperative recovery. Accordingly, the main domains of postoperative recovery consisting of physiologic, nociceptive, functional, cognitive and emotional recovery are assessed over time. The development of the PostopQRS was started in 2007 by an international research group [65]. Subsequently, a refinement was performed in accordance with sector good practices such as the United States Food and Drug Administration (FDA) guidance. Ongoing developments have further suggested that the PostopQRS allows for analysing normal cognitive performance variability, discriminating changes between patient groups and detecting cognitive decline prior to surgery. In addition, the PostopQRS has been validated for surgical patients ≥6 years, can be completed within 5–6 minutes and can also be done using a pen and paper completion questionnaire [65, 66, 67].
Since the quality of life after patient discharge from the hospital is of crucial importance, further simple tools evaluating the quality of recovery after major surgeries appropriate for clinical practice such as the PostopQRS may gain in importance in the future [67, 68].
3.4 Screening for dementia by psychometric tests
Psychometric tests can be used to screen for specific stages of dementia, which plays an important role in perioperative patient care. In 1975, the Mini-Mental-Status-Exam (MMSE) has been established as clinical tool allowing for grading perioperative cognitive dysfunction. Using MMSE, cognitive function is assessed by 30 tasks examining temporal and spatial orientation, retentiveness, memory capability, attention, language and speech comprehension, reading and writing capability, drawing and calculating. Each successfully resolved task gives one point and accordingly achievable a maximum score of 30 points. Typically, a score < 25 represents a mild cognitive impairment (MCI), a score < 20 a moderate cognitive impairment and a score < 10 a severe cognitive impairment or dementia—however, these cut-off points may not be appropriate for each patient [69, 70].
Although the MMSE can be used as an efficient clinical psychometric test for both grading cognitive impairment and detecting dementia perioperatively, sensitivity and specificity may be impaired by various features including patient age, individual education, reduced visual and hearing performance, tremors, difficulties in reading, a preceding stroke or TIA, nervousness, pain and the hospital environment. Hence, another psychometric test, the Montreal Cognitive Assessment (MoCA), has been developed, which might be superior to the MMSE—in particular in detecting MCI [70]. One more test, the Functional Assessment Staging Test (FAST) allowing for grading AD (1–7f) has been developed in 1984 [71, 72].
Finally, since, for one thing, MCI and dementia increase related to a growing elderly population and, on the other hand, improved cognitive abilities can be observed in individual elderly people, psychometric tests should be regularly updated to stay accurate in clinical practice [73].
3.5 Neuroimaging using magnetic resonance imaging (MRI) and computed tomography (CT) scan
Modern MRI algorithms have significantly contributed to current knowledge about perioperative neuropsychiatric morbidities, in particular concerning impaired perfusion of the white matter of the brain [41]. The term subcortical vascular dementia incorporates lacunar strokes, Binswanger’s disease and vascular dementia. Lacunar strokes represent small-sized infarcts based on the occlusion of small cerebral arteries penetrating the basal ganglia, the brain stem and the white matter—potentially associated with cerebral microangiopathy based on hypertension. Binswanger’s disease is represented as WMH using MRI, which shows bilaterally spotted brain areas diffusely localised in the white matter. In the T2 weighted image—one of the basic pulse sequences of MRI highlighting differences in the T2 relaxation time of tissues—WMH can be displayed. These WMH are probably caused by reduced perfusion of the white matter, while the grey matter is typically normally perfused [74]. Histopathologically, a gliosis, a demyelination and a loss of neuronal axons can be seen. Currently, it remains unclear whether vascular dementia is primarily based on a disturbed BBB or on chronic hypoperfusion inducing an inflammatory brain response—potentially depending on microangiopathy. MRI studies suggest that WMH may be a predictor for POCD onset [75]. The early clinical sign of a gait disorder with a tendency to fall can be seen in both vascular dementia and Binswanger’s disease.
Diffusion tensor imaging (DTI)—an MRI technique that uses anisotropic diffusion to estimate the white matter organisation of the brain—may allow for detecting early stages of cerebral injuries. In particular, pathological changes in the white matter can be precisely displayed, which can be used in the diagnosis of early strokes within the white matter. Further applications include the detection of an early onset of AD, schizophrenia, focal cortical dysplasia, MS including plaque assessment, early identification of peripheral nerve pathology and presurgical planning. Finally, fibre tractography is a three-dimensional reconstruction technique based on DTI to assess white matter tracts [76].
CT scans are less exact than modern MRI techniques; however, they are much more widely available. In clinical practice, performing a perioperative CT scan is quite easier than an MRI—in particular with critically ill patients. Therefore, CT scans are a good option to detect cerebral bleeding, while ischaemic strokes are later and less precisely seen on a CT scan than on MRI. Furthermore, computed tomography angiography (CTA)—using an injection of a contrast media into a venous blood vessel—allows for detecting blood vessel diseases like aneurysms, stenoses and occlusions. Both CT scans and MRI can beneficially support individual perioperative patient care.
3.6 Non-invasive screening tools for neuropsychiatric morbidities
Modern non-invasive screening tools to detect neuropsychiatric morbidities include measuring the intima-media thickness (IMT), TCD ultrasound, NIRS and processed EEG (pEEG).
Assessing the IMT allows for detecting subclinical atherosclerosis and should be done in every asymptomatic adult perioperative patient having a moderate risk for cardiovascular disease and in hypertensive patients. An IMT > 0.9 mm according to the ESC or over the 75th percentile according to the American Society of Echocardiography (ASE) is considered as pathological. A carotid artery ultrasound scan is the method of choice for measuring the IMT. However, the results of IMT may be biased by different factors including the present haemodynamic, blood pressure levels and the stretching of cerebral vessels [77].
Since 1982, intracerebral vessels can be evaluated by TCD ultrasound [48]. Currently, an advanced, multifrequency pulsed TCD ultrasound is the device of choice to investigate in particular both middle cerebral arteries through the temporal acoustic window. The cerebral blood flow velocity (CBFV) can be continuously recorded, and a reduced CBFV can potentially indicate that a reduced cerebral perfusion is present. However, a wide range of the mean CBFV (38–86 cm/s) of the middle cerebral artery—which further depends on the angle of the transducers’ position—has to be considered. Calculating the pulsatility index (PI [PI = (Systolic velocity – diastolic velocity)/mean velocity]) allows for examining cerebrovascular resistance. A PI >1 indicates an elevated vascular resistance typically combined with reduced vascular compliance—which is often based on arterial hypertension and other cardiovascular risk factors [78].
According to current literature, a high patient age is associated with a reduced CBFV and an elevated PI [79]. TCD ultrasound enables the detection of various cerebrovascular pathologies in real time including cerebral ischaemia [78]. Notably, reduced cerebral perfusion is suggested to be associated with higher cardiovascular and non-cardiovascular mortality [2].
A further application of TCD ultrasound is measuring dynamic cerebral autoregulation using CBFV and MAP as input signals [48]. This approach may optimise blood pressure adjustment perioperatively, which may be especially important since a disturbed cerebral autoregulation commonly impairs cerebral perfusion. Importantly, dynamic cerebral autoregulation may be frequently impaired in the elderly population and in multiple neuropsychiatric and other morbidities including MCI, AD, sepsis, hypothermia, anaemia, hypercapnia, obstructive sleep apnoea and acute respiratory distress syndrome—which all may occur during perioperative patient care [78]. In addition, modern multifrequency TCD devices allow for quantifying cerebral microemboli and further differentiate microemboli into gaseous or solid ones by TCD software algorithms [80]. Cerebral microemboli are for example detectable distal of a stent or during postoperative intensive care if patients are treated with extracorporeal circuits such as dialysis or ECMO—especially venoarterial ECMO may induce a high number of cerebral microemboli (Figure 1) [10]. According to current research, the clinical consequences of cerebral microemboli remain unclear—suggesting that they could play a role in the pathophysiology of more subtle neuropsychiatric morbidities like POCD and MCI [18, 81].
Currently, TCD ultrasound is routinely performed in neurocritical care patients, while in other critically ill patients, it is rarely done during the perioperative period. The main limitation of TCD ultrasound is an inadequate temporal acoustic window (10–15%) [78].
NIRS uses near-infrared light (wavelength of 700–900 nm) being able to penetrate living tissue. The technical principle is based on the fact that the ratio of infrared light absorbed to that scattered by haemoglobin changes depending on the degree of haemoglobin binding with oxygen. NIRS calculates this rate of change and the change in oxygenated haemoglobin concentration. These changes in rSO2 correlate with the regional cerebral oxygenation, estimating roughly regional cerebral blood flow. To indicate regional cerebral oxygenation, the tissue oxygen index (TOI; standard value = 50%) is calculated. NIRS-based analyses may indicate a reduced regional cerebral perfusion since rSO2 accordingly decreases, which is displayed as a lower level of TOI—a TOI < 35% indicates potential tissue damage based on cerebral ischaemia. However, rSO2 depends additionally on other features such as body temperature and present haemoglobin values. Nonetheless, NIRS provides an easily applicable tool to monitor rSO2 during the perioperative period [82].
Continuous pEEG monitoring is technically complex and rather prone to artefacts. During the last decades, different pEEG methods have been developed such as the bispectral index (BIS) and Narcotrend, to reduce, in particular, intraoperative awareness. In addition, pEEG monitoring should help anaesthesiologists in both targeting an optimised depth of anaesthesia and shortening the anaesthetic recovery phase [83]. The prevalence of intraoperative awareness is about 0.5–2/1000 patients. After reviewing the available literature, it remains unclear whether pEEG monitoring significantly reduces intraoperative awareness. In line with current research, pEEG-guided TIVA based on propofol may reduce intraoperative awareness, while a minimum alveolar concentration (MAC)-guided volatile anaesthesia may be equally effective [84, 85, 86]. Accordingly, pEEG-guided anaesthesia may be a beneficial tool but cannot replace the intraoperative clinical evaluation of the depth of anaesthesia (e.g. heart frequency, blood pressure levels, ventilation patterns, sweating and slight body movements) [86]. Finally, current research may show whether pEEG monitoring can be beneficially used during postoperative patient care—in particular in critically ill patients.
4. Treatment recommendations for perioperative anaesthesiologic care of non-cardiac surgical patients with neuropsychiatric morbidities
4.1 Perioperative hypotension and cerebral hypoxia
Multiple studies have shown that intraoperative hypotension is associated with increased general organ impairment, which may be also applicable to the brain [87]. Prolonged intraoperative hypotension during major surgeries may elevate the risk for postoperative stroke—however, research has produced inconclusive data [33, 87, 88]. In line with current knowledge, the accumulation of intraoperative hypotensive periods giving the complete time of intraoperative hypotension seems to be of significant clinical importance, since clinical practice has shown that multiple hypotensive periods during intraoperative anaesthesia can frequently happen. Accordingly, increasing total hypotensive periods may elevate in non-cardiac surgical patients the risk for postoperative cerebral impairment and may increase the postoperative mortality in elderly patients, while short periods of the lowest acceptable intraoperative blood pressures are suggested to be better tolerated [89].
Currently, it can be recommended to target blood pressure adjustment intraoperatively—applicable to both adults and infants—in relation to baseline levels present in a calm preoperative waking state within a range of ±20% (maximally ±30%), concerning additionally the evidence suggesting that a MAP threshold <55–60 mmHg is frequently associated with organ injury [90, 91]. Importantly, this threshold may be unsafe for individual patients including those with coronary artery or cerebrovascular disease potentially requiring a significantly higher MAP [91]. Accordingly, perioperative blood pressure adjustment should be performed based on individual patient demand, since an optimised blood pressure is crucial for sufficient cerebral oxygenation. Similar to the potential harm of intraoperative accumulation of hypotensive periods [89], an accumulation of diffuse cerebral hypoxia impairs postoperative recovery in patients undergoing major general surgeries [92]. Considering clinical practice, in non-cardiac surgical patients, an intermittent cerebral hypoxia, can occur in up to one out of four patients and is associated with POCD and a longer hospital stay—in particular in the elderly patient population [93].
4.2 Triple-low
The term triple-low has been established by Sessler’s research group in 2012 and refers to a low BIS (<45), a low MAP (<75 mmHg) and a low MAC (<0.8) during surgery—which has been shown being associated with an increased risk for patient mortality [94].
Since an association between a BIS <45 and postoperative mortality has been reported in non-cardiac surgical patients, the depth of anaesthesia has been discussed by many clinicians to be causally related to this finding [94]. However, aside from inducing hypotension, there is currently no evidence that anaesthetic agents are directly toxic to the brain or other organs. In addition, one would expect a decreased mortality in surgical patients undergoing regional instead of general anaesthesia—which could not be shown according to current research [91]. Although a cumulative time of BIS <45 in cardiac surgery has been related to postoperative patient mortality, a BIS <45 was paradoxically not associated with an increased volatile anaesthetic exposure. Even more surprisingly, an increased volatile anaesthetic exposure was not associated with patient mortality [95]. Hence, the occasional dissociation between a low BIS and a high volatile anaesthetic concentration remains to be elucidated.
Sessler’s large study including a non-cardiac surgical population showed that triple-low was highly predictive of postoperative death [94]. However, regarding controversies in science, some studies did not support this association [91]. Accordingly, Goodman’s study group recommended a standard approach for judging research reproducibility [96]. The main reason for conflicting study results is suggested to be the BIS algorithm. While both blood pressure and volatile anaesthetic concentrations can be measured using quantifiable units such as mmHg and kPa, allowing for a reliable comparison between patients, BIS algorithm includes a concept of a linear scale from 100 to 0, reflecting the depth of anaesthesia. This scale concept is further not supported by a biological rationale, and in addition, BIS algorithm has been regularly revised over time—which probably leads to a lack of reliable patient comparison. Furthermore, the BIS scale does not decrease consistently with the depth of anaesthesia and sometimes paradoxically increases though volatile anaesthetic concentration increases [97]. In addition, aside from being vulnerable to artefacts (e.g. extracranial electrical activity), the sole application of neuromuscular blocking agents significantly decreases BIS [98]. Hence, a low BIS might not be a reliable parameter within the triple-low concept to predict patient mortality, while other intraoperative EEG features like electrical suppression are related to impaired postoperative outcome [91].
Finally, in line with current knowledge, triple-low events can significantly predict a higher postoperative mortality rate but in particular a low BIS may be not related to the underlying causality [91, 99].
4.3 The 2-hit model of cerebral impairment
In line with current knowledge, an individually adapted perioperative anaesthesiologic patient care—including an optimised intraoperative anaesthesia—seems to be crucial for postoperative patient outcome [87, 91]. The 2-hit model of perioperative cerebral impairment describes a hypothetical theory based on current research [39, 89, 99] similar to a suggested 2-hit model of PTSD [100]. In particular, pre-existing cerebrovascular diseases (i.e. macro- and microangiopathy) together with a pathophysiological ageing process may lead to a first cerebral impairment (first hit) [39], which may be well compensated by individual elderly patients [41]. Subsequently, perioperative surgical and anaesthesiologic patient care may induce a second cerebral impairment (second hit), which seems to be much more difficult to compensate by the patient—particularly in cases of major surgeries, critical illness and postoperative intensive care [8, 18].
Depending on the severity of the first and second hit, considering individual patients’ resilience such as CR or patient age, neuropsychiatric morbidities may be induced (Figure 3). Concerning postoperative patient outcome, it is of major importance to significantly reduce the second hit by individually optimised perioperative anaesthesiologic patient care [91].
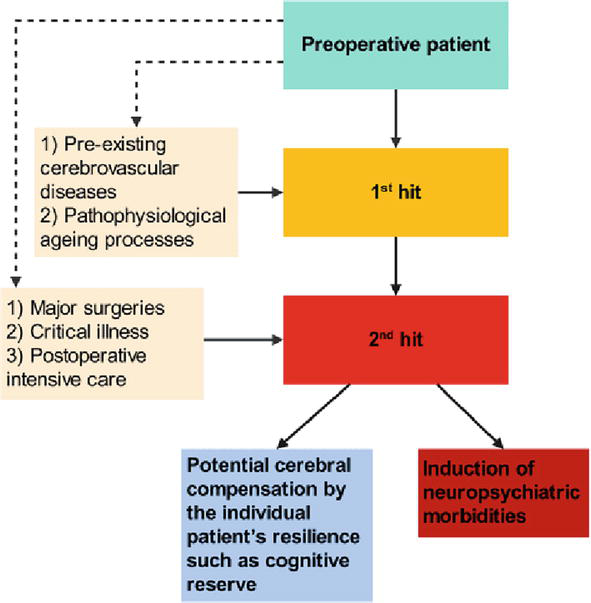
Figure 3.
The 2-hit model of cerebral impairment. While the first hit may be well compensated by individual elderly patients, the second hit seems being much more difficult to be compensated.
5. Conclusions
In summary, neuropsychiatric morbidities play a significant role in perioperative anaesthesiologic patient care—including preoperative assessment, intraoperative general or regional anaesthesia and postoperative intensive care in case of critical illness or major surgeries—due to their associated impaired postoperative quality of life and their affection of overall patient outcome. Therefore, a key factor is the maintenance of an individual’s patient homeostasis. Accordingly, this concept includes that baseline levels (e.g. blood pressure, heart frequency and specific blood values such as natrium and CO2) the individual patient has been getting used to potentially over the years, should be only adapted within a rather small range (i.e. ± 20%). In case of unknown preoperative baseline levels, the following targets should be aspired to: normotension, normovolaemia, normocapnia, normoxaemia, normothermia and normoglycaemia. Patients at higher risk for neuropsychiatric morbidities may benefit from additional neuromonitoring tools (e.g. NIRS, TCD ultrasound and pEEG-guided anaesthesia). In addition, surgical patients profit from early mobilisation, an optimised pain therapy and an early return to everyday life as soon as possible after surgery. Considering in particular postoperative intensive care and critical illness, these treatment steps should be completed with further individual anaesthesiologic patient care (e.g. early weaning from the respirator or at least targeting spontaneous breathing and a treatment of postoperative delirium in line with current guidelines). Finally, the development of new neuromonitoring techniques will may allow for reducing perioperative neuropsychiatric morbidities in the future.
Acknowledgments
I especially thank
All sources of funding were provided by the Department of General Anaesthesia and Critical Care, Medical University of Vienna, Austria. No external funding was obtained.
References
- 1.
Fleisher LA, Fleischmann KE, Auerbach AD, Barnason SA, Beckman JA, Bozkurt B, et al. 2014 ACC/AHA guideline on perioperative cardiovascular evaluation and management of patients undergoing noncardiac surgery: A report of the American College of Cardiology/American Heart Association Task Force on Practice Guidelines. Circulation. 2014; 130 :e278-e333. DOI: 10.1161/CIR.0000000000000106 - 2.
Sabayan B, van der Grond J, Westendorp RG, Jukema JW, Ford I, Buckley BM, et al. Total cerebral blood flow and mortality in old age: A 12-year follow-up study. Neurology. 2013; 81 :1922-1929. DOI: 10.1212/01.wnl.0000436618.48402.da - 3.
Kristensen SD, Knuuti J, Saraste A, Anker S, Botker HE, Hert SD, et al. 2014 ESC/ESA guidelines on non-cardiac surgery: Cardiovascular assessment and management: The Joint Task Force on non-cardiac surgery: Cardiovascular assessment and management of the European Society of Cardiology (ESC) and the European Society of Anaesthesiology (ESA). European Heart Journal. 2014; 35 :2383-2431. DOI: 10.1093/eurheartj/ehu282 - 4.
Kehlet H, Jensen TS, Woolf CJ. Persistent postsurgical pain: Risk factors and prevention. Lancet. 2006; 367 :1618-1625. DOI: 10.1016/S0140-6736(06)68700-X - 5.
Moyce Z, Rodseth RN, Biccard BM. The efficacy of peri-operative interventions to decrease postoperative delirium in non-cardiac surgery: A systematic review and meta-analysis. Anaesthesia. 2014; 69 :259-269. DOI: 10.1111/anae.12539 - 6.
Girard TD, Pandharipande PP, Ely EW. Delirium in the intensive care unit. Critical Care. 2008; 12 (Suppl. 3):S3. DOI: 10.1186/cc6149 - 7.
Rudolph JL, Marcantonio ER. Review articles: Postoperative delirium: Acute change with long-term implications. Anesthesia and Analgesia. 2011; 112 :1202-1211. DOI: 10.1213/ANE.0b013e3182147f6d - 8.
Zaal IJ, Devlin JW, Peelen LM, Slooter AJ. A systematic review of risk factors for delirium in the ICU. Critical Care Medicine. 2015; 43 :40-47. DOI: 10.1097/CCM.0000000000000625 - 9.
Lee HB, Mears SC, Rosenberg PB, Leoutsakos JM, Gottschalk A, Sieber FE. Predisposing factors for postoperative delirium after hip fracture repair in individuals with and without dementia. Journal of the American Geriatrics Society. 2011; 59 :2306-2313. DOI: 10.1111/j.1532-5415.2011.03725.x - 10.
Kietaibl C, Horvat Menih I, Engel A, Ullrich R, Klein KU, Erdoes G. Cerebral microemboli during extracorporeal life support: A single-centre cohort study. European Journal of Cardio-Thoracic Surgery. 2021; 61 :172-179. DOI: 10.1093/ejcts/ezab353 - 11.
Piva S, McCreadie VA, Latronico N. Neuroinflammation in sepsis: Sepsis associated delirium. Cardiovascular & Hematological Disorders Drug Targets. 2015; 15 :10-18. DOI: 10.2174/1871529x 15666150108112452 - 12.
Wachtendorf LJ, Azimaraghi O, Santer P, Linhardt FC, Blank M, Suleiman A, et al. Association between intraoperative arterial hypotension and postoperative delirium after noncardiac surgery: A retrospective multicenter cohort study. Anesthesia and Analgesia. 2022; 134 :822-833. DOI: 10.1213/ANE. 0000000000005739 - 13.
Mashour GA, Woodrum DT, Avidan MS. Neurological complications of surgery and anaesthesia. British Journal of Anaesthesia. 2015; 114 :194-203. DOI: 10.1093/bja/aeu296 - 14.
Khanna AK, Shaw AD, Stapelfeldt WH, Boero IJ, Chen Q , Stevens M, et al. Postoperative hypotension and adverse clinical outcomes in patients without intraoperative hypotension, after noncardiac surgery. Anesthesia & Analgesia. 2021; 132 :1410-1420. DOI: 10.1213/ANE.0000000000005374 - 15.
Smischney NJ, Shaw AD, Stapelfeldt WH, Boero IJ, Chen Q , Stevens M, et al. Postoperative hypotension in patients discharged to the intensive care unit after non-cardiac surgery is associated with adverse clinical outcomes. Critical Care. 2020; 24 :682. DOI: 10.1186/s13054-020-03412-5 - 16.
Newman S, Stygall J, Hirani S, Shaefi S, Maze M. Postoperative cognitive dysfunction after noncardiac surgery: A systematic review. Anesthesiology. 2007; 106 :572-590. DOI: 10.1097/00000542-200703000-00023 - 17.
Travica N, Lotfaliany M, Marriott A, Safavynia SA, Lane MM, Gray L, et al. Peri-operative risk factors associated with post-operative cognitive dysfunction (POCD): An umbrella review of Meta-analyses of observational studies. Journal of Clinical Medicine. 2023; 12 :1610. DOI: 10.3390/jcm12041610 - 18.
Silbert BS, Evered LA, Scott DA, Rahardja S, Gerraty RP, Choong PF. Review of transcranial Doppler ultrasound to detect microemboli during orthopedic surgery. AJNR. American Journal of Neuroradiology. 2014; 35 :1858-1863. DOI: 10.3174/ajnr.A3688 - 19.
Parker AM, Sricharoenchai T, Raparla S, Schneck KW, Bienvenu OJ, Needham DM. Posttraumatic stress disorder in critical illness survivors: A meta analysis. Critical Care Medicine. 2015; 43 :1121-1129. DOI: 10.1097/CCM.0000000000000882 - 20.
Wofford K, Hertzberg M, Vacchiano C. The perioperative implications of posttraumatic stress disorder. AANA Journal. 2012; 80 :463-470 PMID: 23409641 - 21.
Drews T, Franck M, Radtke FM, Weiss B, Krampe H, Brockhaus WR, et al. Postoperative delirium is an independent risk factor for posttraumatic stress disorder in the elderly patient: A prospective observational study. European Journal of Anaesthesiology. 2015; 32 :147-151. DOI: 10.1097/EJA.0000000000000107 - 22.
Mashour GA. Posttraumatic stress disorder after intraoperative awareness and high-risk surgery. Anesthesia and Analgesia. 2010; 110 :668-670. DOI: 10.1213/ANE.0b013e3181c35926 - 23.
Wintermann GB, Brunkhorst FM, Petrowski K, Strauss B, Oehmichen F, Pohl M, et al. Stress disorders following prolonged critical illness in survivors of severe sepsis. Critical Care Medicine. 2015; 43 :1213-1222. DOI: 10.1097/CCM.0000000000000936 - 24.
Regier DA, Kuhl EA, Kupfer DJ. The DSM-5: Classification and criteria changes. World Psychiatry. 2013; 12 :92-98. DOI: 10.1002/wps.20050 - 25.
Aceto P, Perilli V, Lai C, Sacco T, Ancona P, Gasperin E, et al. Update on post-traumatic stress syndrome after anesthesia. European Review for Medical and Pharmacological Sciences. 2013; 17 :1730-1737 PMID: 23852895 - 26.
O'Doherty DC, Chitty KM, Saddiqui S, Bennett MR, Lagopoulos J. A systematic review and meta-analysis of magnetic resonance imaging measurement of structural volumes in posttraumatic stress disorder. Psychiatry Research. 2015; 232 :1-33. DOI: 10.1016/j.pscychresns.2015.01.002 - 27.
Roberts MB, Glaspey LJ, Mazzarelli A, Jones CW, Kilgannon HJ, Trzeciak S, et al. Early interventions for the prevention of posttraumatic stress symptoms in survivors of critical illness: A qualitative systematic review. Critical Care Medicine. 2018; 46 :1328-1333. DOI: 10.1097/CCM.0000000000003222 - 28.
Hori H, Kim Y. Inflammation and post-traumatic stress disorder. Psychiatry and Clinical Neurosciences. 2019; 73 :143-153. DOI: 10.1111/pcn.12820 - 29.
Mashour GA, Shanks AM, Kheterpal S. Perioperative stroke and associated mortality after noncardiac, nonneurologic surgery. Anesthesiology. 2011; 114 :1289-1296. DOI: 10.1097/ALN.0b013e318216e7f4 - 30.
Ng JL, Chan MT, Gelb AW. Perioperative stroke in noncardiac, nonneurosurgical surgery. Anesthesiology. 2011; 115 :879-890. DOI: 10.1097/ALN.0b013e31822e9499 - 31.
Neuro VI. Perioperative covert stroke in patients undergoing non-cardiac surgery (NeuroVISION): A prospective cohort study. Lancet. 2019; 394 :1022-1029. DOI: 10.1016/S0140-6736(19)31795-7 - 32.
Mashour GA, Moore LE, Lele AV, Robicsek SA, Gelb AW. Perioperative care of patients at high risk for stroke during or after non-cardiac, non-neurologic surgery: Consensus statement from the Society for Neuroscience in Anesthesiology and Critical Care*. Journal of Neurosurgical Anesthesiology. 2014; 26 :273-285. DOI: 10.1097/ANA.0000000000000087 - 33.
Group PS, Devereaux PJ, Yang H, Yusuf S, Guyatt G, Leslie K, et al. Effects of extended-release metoprolol succinate in patients undergoing non-cardiac surgery (POISE trial): A randomised controlled trial. Lancet. 2008; 371 :1839-1847. DOI: 10.1016/S0140-6736(08)60601-7 - 34.
Selim M. Perioperative stroke. The New England Journal of Medicine. 2007; 356 :706-713. DOI: 10.1056/NEJMra062668 - 35.
Perks A, Cheema S, Mohanraj R. Anaesthesia and epilepsy. British Journal of Anaesthesia. 2012; 108 :562-571. DOI: 10.1093/bja/aes027 - 36.
Lee SK. Diagnosis and treatment of status epilepticus. Journal of Epilepsy Research. 2020; 10 :45-54. DOI: 10.14581/jer.20008 - 37.
Pollak L, Gandelman-Marton R, Margolin N, Boxer M, Blatt I. Clinical and electroencephalographic findings in acutely ill adults with non-convulsive vs convulsive status epilepticus. Acta Neurologica Scandinavica. 2014; 129 :405-411. DOI: 10.1111/ane.12200 - 38.
Kaproth-Joslin KA, Bhatt S, Scoutt LM, Rubens DJ. The essentials of extracranial carotid ultrasonographic imaging. Radiologic Clinics of North America. 2014; 52 :1325-1342. DOI: 10.1016/j.rcl.2014.07.010 - 39.
Group LS. 2001-2011: A decade of the LADIS (Leukoaraiosis and DISability) study: What have we learned about white matter changes and small-vessel disease? Cerebrovascular Diseases. 2011; 32 :577-588. DOI: 10.1159/000334498 - 40.
Pantoni L. Cerebral small vessel disease: From pathogenesis and clinical characteristics to therapeutic challenges. Lancet Neurology. 2010; 9 :689-701. DOI: 10.1016/S1474-4422(10)70104-6 - 41.
Hannawi Y, Vaishnav A, Coskun EP, Gangadhara S, Romero JR. Covert cerebral small vessel disease: Ready for clinical prime time. Journal of the American Heart Association. 2023; 12 :e029891. DOI: 10.1161/JAHA.123.029891 - 42.
Gottesman RF, Coresh J, Catellier DJ, Sharrett AR, Rose KM, Coker LH, et al. Blood pressure and white-matter disease progression in a biethnic cohort: Atherosclerosis risk in communities (ARIC) study. Stroke. 2010; 41 :3-8. DOI: 10.1161/STROKEAHA.109.566992 - 43.
Goldberg I, Auriel E, Russell D, Korczyn AD. Microembolism, silent brain infarcts and dementia. Journal of the Neurological Sciences. 2012; 322 :250-253. DOI: 10.1016/j.jns.2012.02.021 - 44.
Cavalieri M, Schmidt H, Schmidt R. Structural MRI in normal aging and Alzheimer's disease: White and black spots. Neurodegenerative Diseases. 2012; 10 :253-256. DOI: 10.1159/000333120 - 45.
Scheltens P, De Strooper B, Kivipelto M, Holstege H, Chetelat G, Teunissen CE, et al. Alzheimer's disease. Lancet. 2021; 397 :1577-1590. DOI: 10.1016/S0140-6736(20)32205-4 - 46.
Lassen NA. Cerebral blood flow and oxygen consumption in man. Physiological Reviews. 1959; 39 :183-238. DOI: 10.1152/physrev.1959.39.2.183 - 47.
Moerman A, De Hert S. Why and how to assess cerebral autoregulation? Best Practice & Research. Clinical Anaesthesiology. 2019; 33 :211-220. DOI: 10.1016/j.bpa.2019.05.007 - 48.
Aaslid R, Lindegaard KF, Sorteberg W, Nornes H. Cerebral autoregulation dynamics in humans. Stroke. 1989; 20 :45-52. DOI: 10.1161/01.str.20.1.45 - 49.
Willie CK, Tzeng YC, Fisher JA, Ainslie PN. Integrative regulation of human brain blood flow. The Journal of Physiology. 2014; 592 :841-859. DOI: 10.1113/jphysiol.2013.268953 - 50.
Silverman A, Petersen NH. Physiology, cerebral autoregulation. In: StatPearls. Treasure Island, FL: StatPearls Publishing; 2023 - 51.
Czosnyka M, Miller C. Participants in the international multidisciplinary consensus conference on multimodality monitoring of cerebral autoregulation. Neurocritical Care. 2014; 21 (Suppl. 2):S95-S102. DOI: 10.1007/s12028-014-0046-0 - 52.
Le Roux P, Menon DK, Citerio G, Vespa P, Bader MK, Brophy GM, et al. Consensus summary statement of the international multidisciplinary consensus conference on multimodality monitoring in neurocritical care: A statement for healthcare professionals from the Neurocritical Care Society and the European Society of Intensive Care Medicine. Neurocritical Care. 2014; 21 (Suppl. 2):S1-S26. DOI: 10.1007/s12028-014-0041-5 - 53.
Stern Y. The concept of cognitive reserve: A catalyst for research. Journal of Clinical and Experimental Neuropsychology. 2003; 25 :589-593. DOI: 10.1076/jcen.25.5.589.14571 - 54.
Pettigrew C, Soldan A. Defining cognitive reserve and implications for cognitive aging. Current Neurology and Neuroscience Reports. 2019; 19 :1. DOI: 10.1007/s11910-019-0917-z - 55.
Hoenig MC, Bischof GN, Hammes J, Faber J, Fliessbach K, van Eimeren T, et al. Tau pathology and cognitive reserve in Alzheimer's disease. Neurobiology of Aging. 2017; 57 :1-7. DOI: 10.1016/j.neurobiolaging.2017.05.004 - 56.
Boyle PA, Yu L, Wilson RS, Leurgans SE, Schneider JA, Bennett DA. Person-specific contribution of neuropathologies to cognitive loss in old age. Annals of Neurology. 2018; 83 :74-83. DOI: 10.1002/ana.25123 - 57.
Stern Y. Cognitive reserve. Neuropsychologia. 2009; 47 :2015-2028. DOI: 10.1016/j.neuropsychologia.2009.03.004 - 58.
Soldan A, Pettigrew C, Albert M. Evaluating cognitive reserve through the prism of preclinical Alzheimer disease. The Psychiatric Clinics of North America. 2018; 41 :65-77. DOI: 10.1016/j.psc.2017.10.006 - 59.
Cadar D, Lassale C, Davies H, Llewellyn DJ, Batty GD, Steptoe A. Individual and area-based socioeconomic factors associated with dementia incidence in England: Evidence from a 12-year follow-up in the English longitudinal study of ageing. JAMA Psychiatry. 2018; 75 :723-732. DOI: 10.1001/jamapsychiatry.2018.1012 - 60.
Mosing MA, Lundholm C, Cnattingius S, Gatz M, Pedersen NL. Associations between birth characteristics and age-related cognitive impairment and dementia: A registry-based cohort study. PLoS Medicine. 2018; 15 :e1002609. DOI: 10.1371/journal.pmed.1002609 - 61.
Stern Y, Arenaza-Urquijo EM, Bartres-Faz D, Belleville S, Cantilon M, Chetelat G, et al. Whitepaper: Defining and investigating cognitive reserve, brain reserve, and brain maintenance. Alzheimer's & Dementia. 2020; 16 :1305-1311. DOI: 10.1016/j.jalz.2018.07.219 - 62.
Cabeza R, Albert M, Belleville S, Craik FIM, Duarte A, Grady CL, et al. Maintenance, reserve and compensation: The cognitive neuroscience of healthy ageing. Nature Reviews. Neuroscience. 2018; 19 :701-710. DOI: 10.1038/s41583-018-0068-2 - 63.
Wolf PA. Contributions of the Framingham Heart Study to stroke and dementia epidemiologic research at 60 years. Archives of Neurology. 2012; 69 :567-571. DOI: 10.1001/archneurol.2011.977 - 64.
Monk TG, Weldon BC, Garvan CW, Dede DE, van der Aa MT, Heilman KM, et al. Predictors of cognitive dysfunction after major noncardiac surgery. Anesthesiology. 2008; 108 :18-30. DOI: 10.1097/01.anes.0000296071.19434.1e - 65.
Royse CF, Newman S, Chung F, Stygall J, McKay RE, Boldt J, et al. Development and feasibility of a scale to assess postoperative recovery: The post-operative quality recovery scale. Anesthesiology. 2010; 113 :892-905. DOI: 10.1097/ALN.0b013e3181d960a9 - 66.
Royse CF, Newman S, Williams Z, Wilkinson DJ. A human volunteer study to identify variability in performance in the cognitive domain of the postoperative quality of recovery scale. Anesthesiology. 2013; 119 :576-581. DOI: 10.1097/ALN.0b013e318299f72b - 67.
Bowyer AJ, Heiberg J, Sessler DI, Newman S, Royse AG, Royse CF. Validation of the cognitive recovery assessments with the Postoperative Quality of Recovery Scale in patients with low-baseline cognition. Anaesthesia. 2018; 73 :1382-1391. DOI: 10.1111/anae.14402 - 68.
Poitras S, Wood KS, Savard J, Dervin GF, Beaule PE. Assessing functional recovery shortly after knee or hip arthroplasty: A comparison of the clinimetric properties of four tools. BMC Musculoskeletal Disorders. 2016; 17 :478. DOI: 10.1186/s12891-016-1338-7 - 69.
Sakurai R, Kim Y, Inagaki H, Tokumaru AM, Sakurai K, Shimoji K, et al. MMSE cutoff discriminates hippocampal atrophy: Neural evidence for the cutoff of 24 points. Journal of the American Geriatrics Society. 2021; 69 :839-841. DOI: 10.1111/jgs.17010 - 70.
Jia X, Wang Z, Huang F, Su C, Du W, Jiang H, et al. A comparison of the Mini-Mental State Examination (MMSE) with the Montreal Cognitive Assessment (MoCA) for mild cognitive impairment screening in Chinese middle-aged and older population: A cross-sectional study. BMC Psychiatry. 2021; 21 :485. DOI: 10.1186/s12888-021-03495-6 - 71.
Sclan SG, Reisberg B. Functional assessment staging (FAST) in Alzheimer's disease: Reliability, validity, and ordinality. International Psychogeriatrics. 1992; 4 (Suppl. 1):55-69. DOI: 10.1017/s1041610292001157 - 72.
Na HR, Kim SY, Chang YH, Park MH, Cho ST, Han IW, et al. Functional assessment staging (FAST) in Korean patients with Alzheimer's disease. Journal of Alzheimer's Disease. 2010; 22 :151-158. DOI: 10.3233/JAD-2010-100072 - 73.
Tan JP, Wang X, Lan X, Li N, Zhang S, Zhao Y, et al. The Epoch effect on cognitive function requires regular updating of cognitive screening tests. Journal of Alzheimer's Disease. 2020; 77 :667-674. DOI: 10.3233/JAD-200112 - 74.
Schmidt R, Seiler S, Loitfelder M. Longitudinal change of small-vessel disease-related brain abnormalities. Journal of Cerebral Blood Flow & Metabolism. 2016; 36 :26-39. DOI: 10.1038/jcbfm.2015.72 - 75.
Price CC, Tanner JJ, Schmalfuss I, Garvan CW, Gearen P, Dickey D, et al. A pilot study evaluating presurgery neuroanatomical biomarkers for postoperative cognitive decline after total knee arthroplasty in older adults. Anesthesiology. 2014; 120 :601-613. DOI: 10.1097/ALN.0000000000000080 - 76.
Meoded A, Huisman T. Diffusion tensor imaging of brain malformations: Exploring the internal architecture. Neuroimaging Clinics of North America. 2019; 29 :423-434. DOI: 10.1016/j.nic.2019.03.004 - 77.
Lorenz MW, von Kegler S, Steinmetz H, Markus HS, Sitzer M. Carotid intima-media thickening indicates a higher vascular risk across a wide age range: Prospective data from the Carotid Atherosclerosis Progression Study (CAPS). Stroke. 2006; 37 :87-92. DOI: 10.1161/01.STR.0000196964. 24024.ea - 78.
Purkayastha S, Sorond F. Transcranial Doppler ultrasound: Technique and application. Seminars in Neurology. 2012; 32 :411-420. DOI: 10.1055/s-0032-1331812 - 79.
Tegeler CH, Crutchfield K, Katsnelson M, Kim J, Tang R, Passmore Griffin L, et al. Transcranial Doppler velocities in a large, healthy population. Journal of Neuroimaging. 2013; 23 :466-472. DOI: 10.1111/j.1552-6569.2012.00711.x - 80.
Russell D, Brucher R. Online automatic discrimination between solid and gaseous cerebral microemboli with the first multifrequency transcranial Doppler. Stroke. 2002; 33 :1975-1980. DOI: 10.1161/01.str.0000022809.46400.4b - 81.
Martin KK, Wigginton JB, Babikian VL, Pochay VE, Crittenden MD, Rudolph JL. Intraoperative cerebral high-intensity transient signals and postoperative cognitive function: A systematic review. American Journal of Surgery. 2009; 197 :55-63. DOI: 10.1016/j.amjsurg.2007.12.060 - 82.
Ghosh A, Elwell C, Smith M. Review article: Cerebral near-infrared spectroscopy in adults: A work in progress. Anesthesia & Analgesia. 2012; 115 :1373-1383. DOI: 10.1213/ANE.0b013e31826dd6a6 - 83.
Ellerkmann RK, Soehle M, Kreuer S. Brain monitoring revisited: What is it all about? Best Practice & Research: Clinical Anaesthesiology. 2013; 27 :225-233. DOI: 10.1016/j.bpa.2013.06.006 - 84.
Myles PS, Leslie K, McNeil J, Forbes A, Chan MT. Bispectral index monitoring to prevent awareness during anaesthesia: The B-Aware randomised controlled trial. Lancet. 2004; 363 :1757-1763. DOI: 10.1016/S0140-6736(04)16300-9 - 85.
Avidan MS, Jacobsohn E, Glick D, Burnside BA, Zhang L, Villafranca A, et al. Prevention of intraoperative awareness in a high-risk surgical population. New England Journal of Medicine. 2011; 365 :591-600. DOI: 10.1056/NEJMoa1100403 - 86.
Mashour GA, Shanks A, Tremper KK, Kheterpal S, Turner CR, Ramachandran SK, et al. Prevention of intraoperative awareness with explicit recall in an unselected surgical population: A randomized comparative effectiveness trial. Anesthesiology. 2012; 117 :717-725. DOI: 10.1097/ALN.0b013e31826904a6 - 87.
Bijker JB, Persoon S, Peelen LM, Moons KG, Kalkman CJ, Kappelle LJ, et al. Intraoperative hypotension and perioperative ischemic stroke after general surgery: A nested case-control study. Anesthesiology. 2012; 116 :658-664. DOI: 10.1097/ALN.0b013e3182472320 - 88.
Gottesman RF, Sherman PM, Grega MA, Yousem DM, Borowicz LM Jr, Selnes OA, et al. Watershed strokes after cardiac surgery: Diagnosis, etiology, and outcome. Stroke. 2006; 37 :2306-2311. DOI: 10.1161/01.STR.0000236024.68020.3a - 89.
Bijker JB, van Klei WA, Vergouwe Y, Eleveld DJ, van Wolfswinkel L, Moons KG, et al. Intraoperative hypotension and 1-year mortality after noncardiac surgery. Anesthesiology. 2009; 111 :1217-1226. DOI: 10.1097/ALN.0b013e3181c14930 - 90.
Michelet D, Arslan O, Hilly J, Mangalsuren N, Brasher C, Grace R, et al. Intraoperative changes in blood pressure associated with cerebral desaturation in infants. Paediatric Anaesthesia. 2015; 25 :681-688. DOI: 10.1111/pan.12671 - 91.
Willingham MD, Avidan MS. Triple low, double low: It's time to deal Achilles heel a single deadly blow. British Journal of Anaesthesia. 2017; 119 :1-4. DOI: 10.1093/bja/aex132 - 92.
Casati A, Fanelli G, Pietropaoli P, Proietti R, Tufano R, Danelli G, et al. Continuous monitoring of cerebral oxygen saturation in elderly patients undergoing major abdominal surgery minimizes brain exposure to potential hypoxia. Anesthesia and Analgesia. 2005; 101 :740-747. DOI: 10.1213/01.ane.0000166974.96219.cd - 93.
Casati A, Fanelli G, Pietropaoli P, Proietti R, Tufano R, Montanini S, et al. Monitoring cerebral oxygen saturation in elderly patients undergoing general abdominal surgery: A prospective cohort study. European Journal of Anaesthesiology. 2007; 24 :59-65. DOI: 10.1017/S0265021506001025 - 94.
Sessler DI, Sigl JC, Kelley SD, Chamoun NG, Manberg PJ, Saager L, et al. Hospital stay and mortality are increased in patients having a "triple low" of low blood pressure, low bispectral index, and low minimum alveolar concentration of volatile anesthesia. Anesthesiology. 2012; 116 :1195-1203. DOI: 10.1097/ALN.0b013e31825683dc - 95.
Kertai MD, Pal N, Palanca BJ, Lin N, Searleman SA, Zhang L, et al. Association of perioperative risk factors and cumulative duration of low bispectral index with intermediate-term mortality after cardiac surgery in the B-Unaware Trial. Anesthesiology. 2010; 112 :1116-1127. DOI: 10.1097/ALN.0b013e3181d5e0a3 - 96.
Goodman SN, Fanelli D, Ioannidis JP. What does research reproducibility mean? Science Translational Medicine. 2016; 8 :341ps12. DOI: 10.1126/scitranslmed.aaf5027 - 97.
Whitlock EL, Villafranca AJ, Lin N, Palanca BJ, Jacobsohn E, Finkel KJ, et al. Relationship between bispectral index values and volatile anesthetic concentrations during the maintenance phase of anesthesia in the B-unaware trial. Anesthesiology. 2011; 115 :1209-1218. DOI: 10.1097/ALN.0b013e3182395dcb - 98.
Schuller PJ, Newell S, Strickland PA, Barry JJ. Response of bispectral index to neuromuscular block in awake volunteers. British Journal of Anaesthesia. 2015; 115 (Suppl. 1):i95-i103. DOI: 10.1093/bja/aev072 - 99.
Sessler DI, Turan A, Stapelfeldt WH, Mascha EJ, Yang D, Farag E, et al. Triple-low alerts do not reduce mortality: A real-time randomized trial. Anesthesiology. 2019; 130 :72-82. DOI: 10.1097/ALN.0000000000002480 - 100.
Georgopoulos AP, James LM, Christova P, Engdahl BE. A two-hit model of the biological origin of posttraumatic stress disorder (PTSD). Journal of Mental health and Clinical Psychology. 2018; 2 :9-14