Advanced wound therapies.
Abstract
The landscape of neonatal and pediatric care has changed dramatically in the last decade. As more immature and critically ill neonates survive, newer treatments are offered, innovative approaches are tried and novel medications are developed. The growth and capabilities of clinical care have exploded, but with those, new complications in the care of neonatal and pediatric skin have surfaced. The intricacies of neonatal skin development are many. Birth and survival of extremely premature and critically ill infants facilitated the development of wounds of “immaturity” and wounds of “critical illness” such as extravasations, ostomies, and pressure injuries (PI) related to medical devices. Surgical wound dehiscence has become a familiar entity in neonatal units, as treatments for critical, previously incompatible-with-life conditions offer surgical hope every day. Understanding of pathophysiology of neonatal wounds, challenges in healing, nuances of treatment product compatibility, and safety can guide optimal care pathways. This chapter will offer a discussion on the common neonatal and pediatric wounds, healing stages, and approaches to safe and effective wound healing products, including amniotic membranes and umbilical cord dressings, collagen products, and fish skin grafts.
Keywords
- pediatrics
- neonate
- wound healing
- collagen products
- umbilical membranes
- fish skin graft
1. Introduction
We live in the era of cell therapies renaissance in neonatal and pediatric medicine. Cell therapies do not always imply multipotent “stem” cells, but any biological precursors that have the potential to repair and grow body tissues. Discoveries in the regenerative medicine field identified various components of tissues as an effective therapy to induce the generation and differentiation of cells. These cells can be sourced from many sources, including human and mammalian tissues or synthetic, laboratory- grown preparations. Amniotic membranes and umbilical cord tissues, mammalian, piscine, or synthetic collagens combinations, or fish skin grafts offer new horizons in modern wound care. Research in the regenerative and anti-inflammatory capabilities of these tissues extends into the neonatal lung, brain, and gastrointestinal injury arena. Many are promising to change the trajectory of neonatal medicine but are investigational at this point. However, cutaneous applications of these therapies have been widespread in the adult wound arena and have gained acceptance in the pediatric and neonatal wound community.
The landscape of neonatal care has changed dramatically in the last decade. As more immature and critically ill neonates survive, newer treatments are offered, innovative approaches are tried and novel medications are developed. The growth and capabilities of neonatal care have exploded, but with those, new complications and old inadequacies have come to the surface. The intricacies of neonatal skin development are many. Birth and survival of extremely premature and critically ill infants facilitated the development of wounds of “immaturity” such as medical adhesive related skin injury (MARSI), peripheral venous catheters (PIV) extravasations (PIVE), and wounds of “critical illness” such as pressure injuries (PI) related to medical devices and life-threatening conditions [1, 2, 3, 4]. Surgical wound dehiscence has become a familiar entity in neonatal units. Conditions necessitating gastrostomy, tracheostomy, and intestinal stoma placement have propelled the enterostomal field forward, but have left the neonatal products manufacturers trailing behind. On the flip side of that, care for congenital conditions such as epidermolysis bullosa, ichthyosis, aplasia cutis congenita, and omphalocele have evolved tremendously over the last decade. Understanding of pathophysiology of neonatal wounds, challenges in healing, nuances of treatment product compatibility, and safety can guide optimal care pathways. This chapter will offer a discussion on common neonatal and pediatric wounds, healing stages, and approaches to safe and effective wound healing products, including amniotic membranes and umbilical cord grafts, collagen products, and fish skin grafts.
2. Neonatal skin
Skin is the largest organ and, in a neonate, the one injured the most frequently. Preterm neonatal skin is poorly prepared for the transition to the dry, cool, organism-rich outside environment. The stratum corneum (SC) layer is barely present, leading to increased transepidermal water loss, electrolyte and temperature fluctuations, increased transcutaneous absorption of caustic substances, decreased protection from microbes as acid mantle takes time to develop, and increased susceptibility to dermatitis [1, 2, 3, 4, 5, 6]. Unlike a full-term infant’s skin, preterm skin lacks vernix, important for the formation of the acid mantle, hydration via fatty acids donation, and provision of an antimicrobial barrier. SC cell cohesiveness, important for preventing mechanical trauma, is lacking. Fewer dermal/epidermal fibrils connect the layers, leading to stronger adhesion between the epidermis and an outer dressing than between the dermal/epidermal bond [1, 2, 3, 4, 5, 6]. The dermis is 30% of adult thickness, with minimal subcutaneous fat support. These characteristics amplify the risks for pressure injury and extravasations. Absorption of transcutaneous substances is greater in neonates, leading to a concern of toxicity and suppression of cellular growth, often rendering topical products unsafe or simply not studied and therefore not used in the neonatal population [1, 2, 3, 4, 5, 6, 7].
Infants treated in the neonatal intensive care unit (NICU) are at increased risk of bacterial colonization [8]. Birth via cesarian section predisposes skin to colonization by pathogenic bacteria [8]. Prolonged exposure to antibiotics and invasive devices changes the microbiome milieu, supporting pathogenic microbes’ growth [8, 9, 10]. Critical illness, suboptimal nutrient intake, and hypercatabolic state decrease calorie and protein available for wound healing. In addition, many neonates are deficient in vitamins and trace elements such as zinc, copper, and selenium [11, 12, 13, 14, 15]. Their frequent exposure to oxygen and lack of adequate oxygen radicals’ scavengers leaves tissues exposed to radical damage and inflammation.
3. Neonatal skin injuries
3.1 Medical adhesive related skin injury (MARSI)
Advancements in technology and survival led to the increasing number of gadgets and monitoring devices placed on tiny neonatal bodies. Removal and attachment of adhesive products happens many times a day, leading to a prevalence of MARSI up to 40% in neonatal units [16, 17, 18, 19, 20]. Epidermal stripping, skin tears, and irritant contact dermatitis dominate this class of injuries, but tension blisters, allergic contact dermatitis, and maceration have been reported as well [16, 17]. In 2001 an evidence-based practice project determined that adhesive removal was the primary cause of neonatal skin breakdown, with tape removal contributing 17% of all injuries [17, 18, 19, 20]. A consensus statement on MARSI was published in 2013, including risk factors and prevention guidance [16]. A variety of papers have addressed neonatal MARSI. Functional and structural immaturity of the skin is the most important factor predisposing to MARSI [18, 19, 20]. Yet, because adhesives are so widespread, familiar, and necessary, caregivers fail to recognize the imminent danger they represent; 10–15% of neonatal graduates leave the NICUs with a scar; approximately 15% are functionally and cosmetically significant.
Epidermal stripping is a subtype of MARSI. Studies have documented those certain dressings (films with acrylate adhesives and hydrocolloids) increase the risk of epidermal stripping [16, 17, 18, 19]. Silicone-based is the only atraumatic dressing on the market; it should be used as much as possible in the neonatal population [16]. Few products mitigate the risk for MARSI. A nonalcoholic liquid skin barrier may provide a protective layer between epidermal and adhesives as well as minimize irritation from caustic substances, and bonding agents should not be used in the neonatal population. These agents can increase epidermal stripping, and cases of skin necrosis have been described. A silicone-based adhesive remover should be used with all adhesives; it releases the adhesive bond without leaving the residue (the downfall of oil-based solvent or the toxicity of alcohol-based one), allowing immediate dressing repositioning if desired or complete removal without pain and injury to the SC [17, 18, 19]. Techniques of dressing removal, such as stabilizing the skin and rolling tape/dressing on itself may lessen MARSI [16, 17]. Finally, awareness and understanding of the risks of MARSI, along with an emphasis on prevention reduces these injuries.
3.2 Peripheral intravenous vascular extravasation (PIVE)
Peripheral intravenous vascular catheter placement is the most common procedure performed in the neonatal intensive care unit, yet over 60% of PIV catheters are removed before their intended time due to complications such as occlusion, dislodgement, leaking, phlebitis, infiltration, or extravasation. Up to 70% are reported to be infiltrations and up to 25% are extravasations [21]. Infusion Nurses Society defines extravasation as an inadvertent administration of a vesicant solution or medication that causes the formation of blisters leading to tissue apoptosis or necrosis, whereas infiltration causes damage via hypoxia and mass effect, eventually leading to tissue necrosis [22, 23]. Neonates are at increased risk for extravasations. Immature skin, lack of robust subcutaneous tissue, small vessel diameter, disadvantaged site of IV placement (joint), and inability to verbalize pain are just a few factors [23, 24]. The nature of infuscate neonates receives places them at risk every day. Vesicants injure endothelial cells through direct medication effects, increased osmolality, poor solubility, and non-neutral pH [21, 22, 23, 24]. Once injured, cells trigger an inflammatory cascade by releasing oxygen radicals and pro-inflammatory cytokines. Preterm neonates and sick children are not able to counterbalance this process due to ongoing hypoxemia, mitochondrial injury, lack of energy resources, and limited oxygen radicals’ scavengers. A vicious cycle leads to cellular apoptosis and necrosis, skin, and soft tissue injury, leading to partial or full-thickness wounds [21, 22, 23, 24]. Wound development following PIVE occurs in about 6% [21, 22, 23].
3.3 Pressure injury
Skin is one of the most underdeveloped organs in neonates and, therefore least prepared to withstand the effects of multiple forces exerted by devices. PI due to intrinsic factors (such as immobility, nutrition, and perfusion) are fairly uncommon in neonates. The majority of PI are device-related and non-invasive respiratory devices are most commonly involved [25, 26, 27, 28, 29]. These injuries are followed by injuries from the PIV hub, oxygen saturation probe, and electroencephalography lead [25, 27]. The most common areas injured in neonates as a result of a medical device are the nose and its surrounding area [28]. Transmitted pressure leads to deformation of the cellular cytoskeleton as well as obstructed blood flow, ischemia, further inflammation, and cell death [28]. The sensation can be diminished in the tiniest and sickest babies, and so is mobility as special developmental positioners limit body and head movement. National and international agencies have recommended various bundle elements to reduce PI in general and device-specific PI [29]. Literature questions the utility of available PI skin assessment scales and their ability to statistically prevent pediatric PI occurrence [30]. This translates into the importance of clinical risk understanding. National Pressure Injury Advisory Panel states that all patients with medical devices are considered at risk; certain clinical scenarios (intubation, hypotension, edema, sepsis) multiply the risk [1]. Preventative measures are at the forefront of pediatric PI eradication. Once developed, pressure wounds are treated as wounds from other etiologies.
3.4 Surgical wounds
Surgical wounds in neonates are often a consequence of immaturity, such as dehisced wounds after necrotizing enterocolitis, ostomies due to congenital and acquired conditions, and unanticipated complications following congenital defects repairs. Dehisced wounds are always concerning for developing surgical site infection and optimal wound preparation is required. Effective debridement means biofilm removal and prevention of further formation, followed by antimicrobial coverage, appropriate packing, moisture balance, and protection from the outside environment. Deeper and slow-healing wounds are not uncommon in the high acuity intensive care units. Understanding of cells important in healing stages enhances care and appropriate product selection for these patients.
4. Wound healing stages
Despite the functional and structural immaturity of neonatal skin, the physiologic principles of wound healing are similar along the age continuum. Healing wounds evolve through four distinct dynamic and intertwined stages of healing [31]. Stage 1 starts with hemostasis. Hemostasis is initiated through the activation of a coagulation cascade, attracting various cells, and releasing cytokines as chemical messengers. Platelets are paramount for the fibrin plug formation, local vasodilation, differentiation of cells, and release of chemoattractants. Chemoattractants such as epidermal growth factor, fibroblast growth factor, insulin-like growth factor, and keratinocyte growth factor are instrumental in facilitating the beginning of the inflammatory stage (stage2) and targeted cell movement within the wound in addition to the targeted cellular differentiation [31, 32]. Vasoactive substances such as bradykinin, serotonin, and prostaglandins increase endothelial permeability and cell influx [31, 32]. Cell-to-cell communication and cytokines attract neutrophils, monocytes, and lymphocytes. These cells debride the area from the bacteria, dead cells, and inflammatory milieu. In addition, monocytes differentiate into macrophages, ensuring optimal pro-inflammatory/anti-inflammatory macrophage subtypes ratio. The macrophage becomes the main cell to orchestrate the transition between inflammatory and stage 3, the proliferative stage [31, 32]. Many ancillary chemokines affect this stage. For example, omega-6 polyunsaturated fatty acids intermediates such as linoleic acid, arachidonic acid, leukotrienes, and endothelin are integral in pro-inflammatory chemokines communication. Concomitantly anti-inflammatory omega-3 pathway mediators, such as eicosapentaenoic and docosahexaenoic acids increase over time, leading the wound to the proliferative stage. As the inflammatory stage progresses macrophages signal an influx of fibroblasts and endothelial growth factors; together they facilitate collagen production, and vascular growth, mediated by the production of nitric oxide. The proliferation stage starts with the extracellular matrix deposition via granulation tissue and angiogenesis. Along with the above-mentioned cells, chemokines, growth factors, and matrix elements (such as elastin, hyaluronic acid, proteoglycans, and fibronectin) orchestrate the new tissue formation [31, 32]. Adequate energy and protein building blocks are essential in this stage, together with communication co-factors such as vitamin C, vitamin B, iron, zinc, and copper. Optimal oxygen delivery promotes angiogenesis. Antioxidants and anti-inflammatory mediators are delivered continuously to the area, removing oxygen radicals, pro-inflammatory mediators, and excessive matrix metalloproteinases. Micronutrients such as selenium and Vitamin E are integral for this and inflammatory stages, especially in patients who do not have an adequate supply of superoxide dismutase and other oxygen radical scavengers. As the proliferation stage progresses, the new epithelial tissue growth over the granulation tissue signals the beginning of the final stage- remodeling. Remodeling can last months, ensuring collagen transformation toward mostly type 1 and 3, wound contraction, and scar tissue thinning by selective apoptosis of the described cells [31, 32]. The injured area will not have the same tensile strength as once intact tissue, but the remodeling stage ensures the optimal capacity the tissue can obtain.
Clinical implications of understanding these stages are essential. It is well documented that slowly healing wounds tend to have delayed inflammatory and/or proliferative stages. Such wounds may benefit from a scaffold for the ingrowing cells, exogenous matrix components, growth factors and at times anti-inflammatory mediators as slowly healing wounds may show an overabundance of matrix proteases or may need of a proliferative boost.
4.1 Wound care management
Comprehensive and holistic approach to wound care is recommended. All wounds should be assessed for the debridement need. While sharp debridement is non-specific, painful and rarely performed in pediatrics, the mechanical, enzymatic and autolytic types are common. For wounds with minimal slough I use medical grade honey, surfactant-based gel and rarely hydrogel. I favor medical honey gel for its acidic, hyperosmolar properties, anti-bacterial action and concomitant extracellular matrix growth inducer. Enzymatic collagenase ointment is a potent and safe debrider, especially when thicker slough or eschar is encountered. Finally, mechanical debridement with a monofilament fiber pad or lolly is an effective, quick and relatively painless option when scrubbing is considered. The product needs to be copiously moistened with normal saline, although I often use stabilized hypochlorous acid as my moisture. Hypochlorous acid is non-cytotoxic, gentle and safe. All debridement products I use are safe even in the smallest preterm neonates.
Neonatal and pediatric wound care is a relatively young field. Standard-of-care is mostly established from many case series, opinion leaders and somewhat recently from the societal guidelines. Prior to cellular therapies, packing with hydrogel, hydrofiber, silver-based products and recently medical honey has been the standard. The last decade brought an increased use of NPWT both single-use and canister based. While all the products mentioned are still the standard-of-care in many pediatric practices, newer, advanced therapies are changing the landscape of what pediatric wound doctors are using as their first choice now. Atraumatic wound dressings, NPWT and other supportive measures are out of scope of this chapter.
5. Advanced wound care products
5.1 Collagen
Collagen, produced by fibroblasts, accounts for 30% of the protein in humans and 77% of the dry weight of the skin. Collagen molecules are composed of 3 polypeptide chains that are aligned in a parallel manner and coiled in a left-handed helix. Types 1, 2, 3, 5, and 11 collagens have fibrillary quaternary structures. Type 1 collagen is the most abundant type in animals and most often used in medicine [33, 34, 36].
Collagen is paramount for the healing cascade, stimulating cellular migration in inflammatory and proliferative stages, contributing to the extracellular matrix, and ensuring tissue elasticity and strength in the regenerative stage [35]. Natural collagens can be prepared in many ways, but overall belong to two categories: a decellularized matrix that maintains original tissue properties and extracellular structure or a collagen scaffold prepared by extraction, purification, or polymerization [36, 37]. Collagen dressings take form as sponges, powders, gels, films, membranes or collagen–impregnated cellulose dressings, as well as varieties of skin substitutes auto and allografts [33, 34, 35, 36, 37].
5.2 Use of collagen in wounds
Challenges in neonatal or pediatric wound’s proliferative stage may stem from insufficient amino acids and therefore protein and collagen secondary to frequent hypercatabolic or hyperproliferative state. Most critically ill neonates have increased protein consumption due to ongoing growth, losses (renal disease, congenital heart disease and ostomy patients), cellular differentiation, pro- and anti-inflammatory mediators’ formation, and at times inadequate provision. Most pediatric wounds can benefit from collagen dressing. Given the patient’s small size and at times challenging location of these wounds, collagen gel or powder preparations can be advantageous in application. Wounds with larger wound beds and an easier placement benefit from classic dressing varieties. Most neonatal practitioners use pure collagen preparations, without antimicrobial additives as systemic absorption of additive substances through the immature neonatal skin can be significant.
In my neonatal practice, most wounds respond well to collagen products. Clinically, I see faster and more robust extracellular matrix deposition compared to plain packing or application of hydrogel or medical-grade honey. Appropriate gentle debridement must take place first as wound bed slough slows down ECM growth. A combination of collagen with NPWT works well with dehisced surgical wounds.
Adult and pediatric literature has reported improved outcomes with various collagen preparations, including gels, sheets, and powders. Product versatility, faster healing rates due to the immediate bioavailability and expedited cellular matrix incorporation, and robust fibroblast response are cited [33, 34, 35, 36, 37]. This becomes important as the recruitment of fibroblasts, the cells dominant in the proliferative phase of normal wound healing, can be retarded [35]. In addition, the expression of the collagen gene in some fibroblasts can be suppressed [36]. Collagen products diminish the inflammatory process and decrease matrix metalloprotease destruction of the native intact collagen in the ECM [36, 37].
Case 1 illustrates a dehisced thoracic wound. This patient was born with congenital heart disease and needed a repair at 1 week of age. His wound dehisced by 5 days (Figure 1A). After gentle debridement and decolonization with medical- grade honey for 2 days, collagen gel was applied and covered by portable NPWT. Figure 2B illustrates the wound after 2 applications on Day 3 and Day 9. Robust ECM is appreciated. Collagen gel was reapplied on Days 10 and 15 (Figure 1C). The wound was completely closed in 3 weeks.

Figure 1.
A. Dehisced thoracic wound in a full term, now 2 weeks old neonate. Wound was colonized with MSSA and had slough over the wound bed. After decolonization and debridement, collagen gel was applied under NPWT. B. 11 days later wound is contracting and granulation tissue is appreciated. Wound had 2 applications of collagen gel and portable NPWT. C. Day 15. Collagen gel is applied under a secondary silicon-based dressing as wound is almost fully closed.

Figure 2.
A. Appearance of an eschar from severe peripheral intravenous extravasation. Once debrided, deep thickness wound through the dermis was appreciated. B and C. Collagen powder was applied every 5 days. D. Soft scar with minimal pigmentation loss. Full range-of-motion of the wrist was appreciated at 1-month follow-up clinic visit, despite location over a joint.
Extravasation wounds can be deep in neonates. Collagen dressings offer scaffold to exogenous cells and afford faster healing compared to common management with hydrogel or medical grade honey and secondary dressings. Case 2 shows severe extravasation (Figure 2A) that was deep (once the eschar was debrided) and slow to heal. Using collagen powder allowed for easy and precise application (Figure 2B and C). This wound took 3 weeks to heal, but as you can see on the follow-up 1 month later the scar is soft, pliable, and no limitation of motion despite the location over a wrist joint (Figure 2D).
5.3 Amniotic membranes (AM) and umbilical cord (UC)-based dressings
The fetal membrane is the original cell-based biologic scaffold that is well suited to integrate with the host tissue and provide an optimal environment for cell growth and differentiation. The main mechanisms of action in animals seem to be immune modulation, pro-angiogenic, anti-oxidant, anti-fibrotic, and pro-fibroblast growth. Lack of graft vs. host reaction and human leukocyte antigens immunization makes this product ideal in preterm neonates and critically ill children, where strong immune responses may cause systemic instability. First introduced in 1910 for skin transplantation, it was subsequently found to be useful in the management of burns, and diabetic ulcers, the creation of surgical dressings, and the reconstruction of various tissues [38].
The fetal membranes are composed of two layers: the outer chorion that is in contact with maternal tissue and the thin, avascular inner amniotic membrane that surrounds the embryo. Amnion has 5 layers: epithelium, basement membrane, compact layer, fibroblast layer, and spongy layer. Amniotic epithelial cells produce essential growth factors for epithelization, including epidermal growth factor (EGF), transforming growth factor-a (TGF-a), keratinocyte growth factor, hepatocyte growth factor, nerve growth factor (NGF) and brain-derived neurotrophic factor (BDNF) in addition to transforming growth factor-b (TGF-b) and basic fibroblast growth factor (bFGF), important for angiogenesis.
The epithelium sits on a basement membrane (BM), which is the most pertinent structural part of the amnion from the perspective of application in wound healing. The organizational framework of the basement membrane, provided by the ECM, contains not only an intricate network of macromolecules produced and assembled by surrounding cells but also numerous growth factors along with collagens, laminins, nidogen-1 and 2, fibronectin, fibulin-2, fibrillin-2, perlecan, and agrin. Collagen in BM decreases microbial accumulation, along with cystatin-E, an anti-infective inhibitor, and beta 3-defensin, an antimicrobial peptide [38, 39, 40, 41]. Additionally, many growth factors are found within basal membrane ECM. ECM also contains fibroblasts and neonatal mesenchymal cells (MSCs). MSCs release soluble factors that stimulate proliferation and migration within the wound [39, 40]. MSCs also provide anti-scarring properties via stimulation of the release of vascular endothelial growth factor (VEGF) and hepatocyte growth factor (HGF). Modern evidence shows that MSC cells may exert their effects via MSC-derived extra-cellular vesicles (EV), small secreted membrane vesicles formed through the fusion of endosomes with the cell membrane. EVs are known for their cell-to-cell communication, genetic information transfer via mRNA, and guidance to macrophage differentiation from pro-inflammatory to pro-repair phenotype [38, 40]. The anti-inflammatory effect is moderated by the release of interleukin 10 and thrombospondin-1 [39].
Umbilical cord tissue contains Wharton’s jelly, a rich source of proteoglycans, heavy-chain hyaluronic acid, and glycoproteins. Many dressings incorporate umbilical cord tissue to incorporate the glycoprotein complex for inhibition of pro-inflammatory cell adhesion and their apoptosis, promotion of anti-inflammatory cytokines, and increased dressing integrity.
Both AM and UC dressings have an analgesic effect by covering nerve endings and minimizing wound desiccation. Multiple studies from adult patients revealed the efficacy of AM/UC dressings, including those for chronic wounds, burns, diabetic foot ulcers, and PI. All show successful epithelization with minimum dressing manipulation, softer scars, and shortened time to closure compared to other modalities. Pediatric literature focuses on burn care, while neonatal report superior results with extravasation injuries [39, 40, 41, 42, 43, 44, 45].
In my experience, AM or UC dressings have been excellent in utilization for pressure injuries, deep extravasation wounds, dehisced surgical wounds, and complex congenital conditions. Case 4 images illustrate challenging neonatal PIVI wounds. Location over a joint was concerning for potential contractures affecting limb function, if significant scarring occurred in the healing process. Dehydrated amniotic membrane graft and NPWT were used and resulted in timely healing, with a soft scar and no contractures or limitation of walking.
Case 3 illustrates the application of AM in a stagnant deep sacral PI. This pediatric patient was in a critical state for a few weeks after his diagnosis of cancer and the transplant that followed. He was intubated, septic, immunocompromised and suffered from protein-calorie malnutrition. His repositioning and offloading were dependent on the caregivers and medical staff but were challenging due to a limited reserve and movement tolerance. The sacral pressure injury developed and continued to progress as evidenced by significant depth, tunneling, and undermining. His medical team tried packing the wound with different products but failed to stimulate tissue growth (Figure 3A). At this point, the wound was gently cleaned with hypochlorous acid as aggressive debridement was contraindicated in this coagulopathic patient and packed with cryopreserved umbilical cord allograft. Figure 3B shows tremendous progress after 2.5 weeks and 2 applications of UC products. As wound became shallower, we transitioned to a dehydrated amniotic allograft membrane (Figure 3C). As you can see Figure 3D illustrates healthy, robust granulation tissue we achieved after 2 weeks of AM dressings.

Figure 3.
A. Stage 3 sacral pressure injury. Tunneling and undermining are present. Thin slough covers wound bed and senescent edges are appreciated. B. Day 8. Wound after 1 application of cryopreserved UC. C. Day 17. As wound is smaller and shallow dehydrated amnion allograph placed. D. Day 30. Wound bed is filled-in with robust granulation tissue.
5.4 Intact fish graft
The newest development in xenograft therapies is an intact acellular fish skin graft (FSG), the only non-mammalian piscine xenograft minimally processed from the skin of North Atlantic cod or Nile Tilapia. Research demonstrated that this graft is rich in fats, collagen, fibrin, proteoglycans, and glycosaminoglycans [46, 47]. Omega-3 polyunsaturated fatty acids are particularly plentiful; their anti-inflammatory messengers (resolvins and protectins) promote fibroblast proliferation, endothelial revascularization, and ECM remodeling. Its unique porosity and infrastructure mimic human three-dimensional skin, facilitating cell ingrowth [46, 47, 48]. Since no known viral or prion diseases can be transmitted via this fish to humans, mild chemical skin alteration preserves the original architecture, which seems to be homologous to the human skin [46, 47, 48].
Pediatric studies report on the theoretical advantages of using FSG in young patients, eliminating the need for donor sites and minimizing the risk of infection [49, 50]. Pediatric and especially neonatal dermis is much thinner than adult, which makes pediatric allograft suboptimal. Anti-inflammatory properties of omega-3 PUFAs decrease the propensity for hypertrophic scars, not an uncommon concern in pediatrics. Ciprandi et al. described a large series of pediatric wounds treated successfully and expeditiously with GSG, with shorter application time, faster healing time and shorter hospital stays [49]. Literature demonstrated its effectiveness across burns, surgical wounds, trauma, PI, and in adult diabetic ulcers [46, 47, 48, 49, 50]. Interestingly, all papers noted faster healing compared to previous standards of care(some compared to AM, dermal allogeneic grafts, or intestinal submucosa) and decreased use of antibiotics in adult studies, likely secondary to omega-3 fatty acids support of production of anti-bacterial and anti-viral messengers [47, 48, 49, 50].
I have found success in using novel FSG in challenging wounds, such as Case 4. This neonatal wound was a result of severe necrotizing enterocolitis, requiring extensive surgical bowel resection, ostomy, and mucous fistula creation followed by two bouts of dehiscence. This infant was critically ill, edematous, and malnourished due to a limited remaining bowel. The wound proved challenging to dress due to its proximity to ostomy where an appliance hindered access to the full wound and very limited tolerance of any “hands-on” care. His first dehiscence was successfully closed with collagen therapy; the second though did not respond as well. The skin was thin and ragged, and overall immune and proliferative systems were weak and deficient in nutrients and growth factors. A solid FSG sheet was cut into multiple small pieces, rehydrated with normal saline, and applied across the wound surface (Figure 4A shows dehiscence before FSG application), followed by hydrofiber packing and a hydrocolloid sheet to serve as a base for ostomy appliance placement. Application of FSG was repeated in 10 days for a total of 2 applications (Figure 4B). the wound closed completely in 4 weeks (Figure 4C shows the wound at 10 weeks) and importantly with minimal manipulations, which was essential given the patient’s propensity to deteriorate with clinical care.
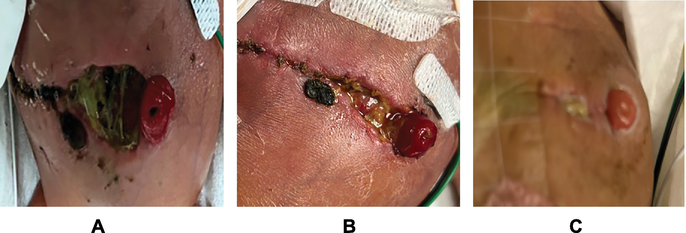
Figure 4.
A. Dehisced abdominal wound. Wound is colonized by enteric organisms as stoma opening is within the wound dehiscence. B. Day 15. S/P 2 GSG applications. Significant wound contraction. C. Follow-up visit 2 months later before ostomy reversal. Soft, pliable scar with normal pigmentation.
6. Discussion
While comparisons of FSG and AM/UC or collagens are scant in pediatric literature, adult -based data has been accumulating. Systematic review of literature comparing FSG and alternative wound dressings was published in 2023 [51]. Overall, literature supports superior healing with FSG in the variety of wounds, comparing to amniotic membranes, collagen-based dressing, silver sulfadiazine cream or bovine dermis. Kirshner et al. compared FSG with dehydrated human amnio-chorion membrane (dHACM) in diabetic ulcers. Fish graft afforded healing more rapidly, was 75% less expensive and had no difference between adverse outcomes in 2 groups [52]. Stone et al. compared FSG and fetal bovine dermis (FBD) as treatments for deep burns [53]. FSG integrated quicker into the wound, wounds required fewer treatments and resulted in faster re-epithelization and wound contraction. Level of blood flow and hydration was superior in FSG treated wounds compared to FBD wounds. Wallner et al. published similar results in the study of deep burns [54] as well as Lima, who compared FSG to a common standard-of-care burn dressing with silver sulfadiazine [55]. His results highlighted 3 times less dressing application and decreased analgesia requirement, decreasing cost of care overall in the group treated with FSG. Winters et al. compared cost-effectiveness of FSG to collagen dressings [55]. The cost savings with FSG was significant, about 25%, in addition to a higher number of fully healed wounds. A large trial by Lullove et al. compared collagen alginate dressings with FSG [56]. Number of required applications was 3 times higher in the collagen group and twice as many patients in the FSG had complete wound resolution.
With time more sophisticated dressings are developed. FSG might have superior qualities compare to many cellular therapies available today (Table 1). Superior anti-inflammatory properties due to LCPUFAs and unique anti-bacterial properties compete head-to-head with various stem cells, cytokines and growth factors that amniotic membranes and umbilical cord dressings provide. While UM/UC dressings cost hundreds to thousands of dollars, cost of FSG starts at 80 to about 500 dollars on average, depending on the size. Comparing to many SOC products used in pediatrics such as silver, honey or hydrogel creams as well as collagen products, both FSG and UM grafts are more expensive per product application. However, faster and complete wound closure and fewer required applications decreased the cost overall.
Advanced wound therapies | |||
---|---|---|---|
Collagen | AM/UC | FSG | |
Origin | Human Bovine Porcine Equine Piscine Synthetic Plant-based | Human | Piscine |
Preparation forms | Dehydrated Powder Gel | Dehydrated Cryopreserved Fresh Lyophilized | Dehydrated |
Immunogenicity | Depends on preparation | Low | Low |
Anti-inflammatory effect | Yes, indirectly | Yes | Yes |
Anti-fibrotic effect | No | Yes | No |
Anti-microbial effect | No | No | Yes |
Pain relieving effect | No | Yes | Yes |
Extracellular matrix growth | Direct | Indirect | Indirect |
Reduction in healing time | ↓ | ↓↓ | ↓↓ |
Cost | ↑--↑↑ | ↑↑↑--↑↑↑↑ | ↑↑ |
Table 1.
7. Conclusion
Advanced cellular therapies for neonatal and pediatric skin have to be safe, effective, easy to apply, and take into consideration the challenging size and location of a wound. While adult patients are commonly taken to an operating suite to apply therapeutic products, neonate transport from the neonatal unit is not advisable. Connection to many invasive devices, tube dislodgement, risk of hypothermia, and side effects of anesthesia provide strong deterrents from the treatments necessitating outside transports. Therapies with prolonged shelf-life, easy application, and bolstering are attractive to neonatal and pediatric providers. Collagen products represent such therapy to me. Multiple easy-to-apply preparations and shortened healing time make sense in many hypercatabolic and critically ill children. The price point is reasonable and most pediatric practitioners can apply these products without the need of sub-specialists. I often think of collagen preparations as standard-of-care for every day, easy application by any practitioner in pediatrics.
As advanced biologic cellular therapies developed amniotic membranes and umbilical cord preparations, I incorporated them into my armamentarium. The proliferative and anti-inflammatory boost these products provide is crucial for stagnant wounds. I found these therapies even more integral in areas where contractures, scars, and disfigurement are potentially life-altering. It has become my “first-choice” therapy in a fresh wound located over a joint, hands, feet, or face. These products are very easy to apply (especially the dehydrated amniotic variety), only need re-application once in 7–10 days and a simple secondary dressing or NPWT is sufficient as an outer dressing.
Finally, as FSG has entered the neonatal and pediatric wound arena I can see its reasonable cost, ease of application, anti-inflammatory and, especially anti-microbial properties, leading to a wide-spread use, especially in patients with nutritional deficiencies, bacterial colonization, and minimal tolerance for hand-on handling.
The safety of these products has been evaluated and shown via available literature and I encourage pediatric practitioners to develop the knowledge and skills necessary to apply these therapies to our fragile patients.
References
- 1.
Ness M, Davis D, Carey W. Neonatal skin care: A concise review. International Journal of Dermatology. 2013; 52 :14-22 - 2.
Kusari A, Han A, Virgen A, et al. Evidence-based skin care in preterm infants. Pediatric Dermatology. 2019; 36 :16-23 - 3.
Metzker A, Brenner S, Merlob P. Iatrogenic cutaneous injuries in the neonate. Archives of Dermatology. 1999; 135 :697-703 - 4.
Fox M. Wound care in the neonatal intensive care unit. Neonatal Network. 2010; 30 (5):291-302 - 5.
Johnson D. Extremely preterm infant skin care. Advances in Neonatal Care. 2016; 16 (5S):S26-S32 - 6.
Telofski A, Morello P, Mack Correa C, Stamatas G. The infant skin barrier: Can we preserve, protect, and enhance the barrier? Dermatology Research and Practice. 2012; 2012 :198789 - 7.
Amer Y, Bridges C, Marathe K. Epidemiology, pathophysiology and management strategies of neonatal wound care. Neoreview. 2021; 22 (7):e452-e460 - 8.
Bhatta D, Subramanya S, Hamal D, et al. Bacterial contamination of neonatal intensive care unit: How safe are the neonates? Antimicrobial Resistance & Infection Control. 2021; 26 :1-6 - 9.
Senn V, Bassler D, Choudhury R, et al. Microbial colonization from fetus to early childhood—A comprehensive review. Frontiers in Cellular and Infectious Microbiology. 2020; 10 :1-12 - 10.
Visscher M, McKeown K, Nurre M, et al. Skin care for the extremely low-birthweight infant. Neoreview. 2023; 24 (4):229-242 - 11.
Dao D, Anez-Bustillos L, Cho B, et al. Assessment of micronutrient status in critically ill children: Challenges and opportunities. Nutrients. 2017; 9 :1185 - 12.
Marino L, Valla F, Beattie R, Verbruggen S. Micronutrient status during pediatric critical illness: A scoping review. Clinical Nutriton. 2020; 39 :3571-3593 - 13.
Molnar J, Underdown M, Clark W. Nutrition and wounds. Advances in Wound Care. 2014; 3 (11):663-681 - 14.
Irving S, Berry K, Morgan S, et al. Nutrition associated with skin integrity and pressure injury in critically ill pediatric patients. Nutrition in Clinical Practice. 2023; 38 :S125-S138 - 15.
Berry K, Seiple S, Stellar J, et al. A scoping review to inform a multi-disciplinary approach for nutrition therapy in critically ill children with pressure injuries. Translational Pediatrics. 2021; 10 (10):2799-2813 - 16.
Fumarola S, Allaway R, Callaghan R, et al. Overlooked and underestimated: Medical adhesive-related skin injuries. Best practice consensus document on prevention. Journal of Wound Care. 2020; 29 (S3C):S1-S24 - 17.
Lund C. Medical adhesives in the NICU. Newborn and Infant Nursing Reviews. 2014; 14 :160-165 - 18.
McNichol L, Lund C, Rosen T, Gray M. Medical adhesives and patient safety: State of the science. Journal of Wound, Ostomy and Continence Nursing. 2013; 40 (4):365-380 - 19.
Kim M, Jang J, Kim H, et al. Medical adhesives-related skin injury in a pediatric intensive care unit: A single-center observational study. Journal of Wound, Ostomy, and Continence Nursing. 2019; 46 (6):491-496 - 20.
August D, New K, Ray R, Kandasamy Y. Frequency, location and risk factors of neonatal skin injuries from mechanical forces of pressure, friction, shear and stripping: A systematic literature review. Journal of Neonatal Nursing. 2018; 24 :173-180 - 21.
Pettit J. Assessment of an infant with a peripheral intravenous device. Advances in Neonatal Care. 2003; 3 (5):230-240 - 22.
Infusion Nurses Society. Infusion nursing standards of practice. Journal of Infusion Nursing. 2011; 34 :91S, S65-S67 - 23.
Amjad I, Murphy T, Nylander-Housholder L, et al. A new approach to management of intravenous infiltration in pediatric patients: Pathophysiology, classification and treatment. Journal of Infusion Nursing. 2011; 34 :242-249 - 24.
Frank L, Hummel D, Connel K, et al. The safety and efficacy of peripheral intravenous catheters in all neonates. Neonatal Network. 2001; 20 :33-38 - 25.
Baharestani M. An overview of neonatal and pediatric wound care knowledge and considerations. Ostomy Wound Management. 2007; 53 (6):34-40 - 26.
Baldwin KM. Incidence and prevalence of pressure ulcers in children. Advances in Skin and Wound Care. 2002; 15 (3):121-124 - 27.
Baharestani M. Pressure ulcers in neonates and children: An NPUAP white paper. Advances in Skin & Wound Care. 2007; 20 (4):208-220 - 28.
Gefen A. A new consensus on medical-related pressure ulcers. Journal of Wound Care. 2019; 28 :315 - 29.
Schluer A. Pressure ulcers in maturing skin—A clinical perspective. Journal of Tissue Viability. 2017; 26 :2-5 - 30.
Stellar J, Hasbani N, Kulik L, et al. Medical device-related pressure injuries in infants and children. Journal of Wound, Ostomy, and Continence Nursing. 2020; 47 :459-469 - 31.
Schultz G, Davidson J, Kirsner R, et al. Dynamic reciprocity in the wound environment. Wound Repair and Regeneration. 2011; 19 (2):134-148 - 32.
Gurtner G, Werner S, Barrandon Y, et al. Wound repair and regeneration. Nature. 2008; 453 :314-321 - 33.
Fleck C, Simman R. Modern collagen dressings: Function and purpose. The Journal of the American College of Certified Wound Specialists. 2010; 2 :50-54 - 34.
Brett D. A review of collagen and collagen-based wound dressings. Wounds. 2008; 20 (12):347-356 - 35.
Reinke J, Sorg H. Wound repair and regeneration. European Surgical Research. 2012; 49 :35-43 - 36.
Chattopadhyay S, Raines R. Collagen-based biomaterials for wound healing. Biopolymers. 2014; 101 (8):821-833 - 37.
Hochstein A, Bhatia A. Collagen: Its role in wound healing. Podiatry Management. 2014; 8 (103-106):109-110 - 38.
Schmiedova I, Dembickaja A, Kiselakova L. et al, Using of amniotic membrane derivatives for the treatment of chronic wounds. Membranes. 2021; 11 :941-955 - 39.
Singh S, Srivastava N, Ahmed I, et al. Human amniotic membrane usage from bench to bedside in congenital defects and wounds in pediatric surgery: A systematic review. Medical Research Archives. 2023; 11 (2):1-7 - 40.
Pourmoussa A, Gardner D, Johnson M, Wong A. An update and review of cell-based wound dressings and their integration into clinical practice. Annals of Translational Medicine. 2016; 4 (23):457-467 - 41.
Acevedo P. Successful treatment of painful chronic wounds with amniotic and umbilical cord tissue: A case series. SAGE Open Medical Case Reports. 2020; 8 :1-6 - 42.
Koob T, Lim J, Massee M, et al. Properties of dehydrated human amnion/ chorion composite grafts: Implications for wound repair and soft tissue regeneration. Journal of Biomedical Materials Research B: Applied Biomaterials. 2014; 102B (6):1-10 - 43.
Zelen C, Snyder R, Serena T, Li W. The use of human amnion/ chorion membrane in the clinical setting for lower extremity repair: A review. Clinics in Podiatric Medicine and Surgery. 2015; 32 :135-146 - 44.
Branski L, Herndon D, Celis M, et al. Amnion in the treatment of pediatric partial-thickness facial burns. Burns. 2008; 34 :393-399 - 45.
Tenenhaus M. The use of dehydrated human amnion/chorion membranes in the treatment of burns and complex wounds. Annals of Plastic Surgery. 2017; 78 (1):s11-s13 - 46.
Kim T, Park J, Jeong H, Wee S. The utility of novel fish-skin derived acellular dermal matrix (keresis) as a wound dressing material. Journal of Wound Management and Research. 2021; 17 (1):39-47 - 47.
Esmaelli A, Biazar E, Ebrahimi M, et al. Acellular fish skin for wound healing. International Wound Journal. 2023; 20 :2924-2941 - 48.
Yoon J, Yoon D, Lee H, et al. Wound healing ability of acellular fish skin and bovine collagen grafts for split-thickness donor sites in burn patients: Characterization of acellular grafts and clinical application. International Journal of Biological Macromolecules. 2022; 205 :454-461 - 49.
Ciprandi G, Kjartansson H, Grussu F, et al. Use of acellular intact fish skin grafts in treating acute pediatric wounds during the COVID-19 pandemic: A case series. Journal of Wound Care. 2022; 31 (10):824-831 - 50.
Mitri M, Di Carmine A, Thomas E. Fish skin graft: Narrative review and first application for abdominal wall dehiscence in children. PRS Global Open. 2023; 11 (9):e5244-50. DOI: 10.1097?GOX.0000000000005244 - 51.
Ibrahim M, Ayyoubi H, Alkhairi L, et al. Fish skin grafts versus alternative wound dressings in wound care: A systematic review of the literature. Cureus. 2023; 15 (3):e36348 - 52.
Kirshner R, Margolis D, Baldursson B, et al. Fish skin grafts compared to human amnion/chorion membrane allografts: A double-blind, prospective, randomized clinical trial of acute wound healing. Wound Repair and Regeneration. 2020; 28 :75-80 - 53.
Stone R, Saathoff E, Larson D, et al. Accelerated wound closure of deep partial thickness burns with acellular fish skin graft. International Journal of Molecular Sciences. 2021; 22 :1590 - 54.
Wallner C, Holtermann J, Drysch M, et al. The use of intact fish skin as a novel treatment method for deep dermal burns following enzymatic debridement: A retrospective case-control study. European Burn Journal. 2022; 3 :43-55 - 55.
Lima Junior E, De Moraes F, Costa B, et al. Innovative burn treatment using tilapia skin as a xenograft: A phase II randomized controlled trial. Journal of Burn Care & Research. 2020; 41 :585-592 - 56.
Lullove EJ, Liden B, Winters C, et al. A multicenter, blinded, randomized controlled clinical trial evaluating the effect of omega-3-rich fish skin in the treatment of chronic, nonresponsive diabetic foot ulcers. Wounds. 2021; 33 :169-177