Summary of the latest study on the therapeutic effect of quercetin in gastric cancer.
Abstract
Gastrointestinal (GI) cancers were included in the top 10 most common cancers in 2020. Future incidences are expected to rise due to the varying risk factors and aetiologies. With high incidences and mortality rates, current cancer treatments fail to reduce mortality and morbidity in GI cancer patients. A large part of cancer research has been geared towards targeted and personalized medicine, although effective, it may not be the most cost-friendly and feasible option to treat patients from varying socioeconomic backgrounds. Hence, natural compounds may present as an attractive alternative treatment in the management of GI cancers. Quercetin is a well-known flavonoid compound, found in almost all fruits and vegetables. It has also been widely studied for its anticancer properties, such as anti-oxidative, anti-inflammatory, anti-proliferative and anti-angiogenic properties. In this chapter, the authors discuss the potential of quercetin in treating GI cancers, which includes the biosafety and toxicity of quercetin, applications of quercetin in common GI cancers, such as gastric, hepatic, colorectal, pancreatic and oesophageal cancers, along with the corresponding molecular mechanisms. The authors also present evidences of quercetin as an adjuvant therapeutic agent with other anticancer drugs.
Keywords
- gastrointestinal cancers
- quercetin
- natural products
- cancer treatment
- molecular mechanism
1. Introduction
Gastrointestinal (GI) cancers constitute a formidable health challenge globally, exhibit varying prevalence, epidemiological patterns and mortality rates. Colorectal cancer, with an estimated 1.9 million new cases in 2020, ranks among the most prevalent type of cancer, particularly in developed nations, which is largely influenced by lifestyle factors [1]. Gastric cancer accounts for 1.1 million new cases annually and displays diverse epidemiology linked to regional variations and risk factors such as
The journey towards identifying novel therapeutic strategies for GI cancers begins with understanding current treatments’ shortcomings. Despite advancements in medical science, such as surgical interventions, chemotherapy and targeted therapies, current treatment modalities have not yielded a substantial reduction in mortality and morbidity associated with these malignancies [4]. One glaring example is colorectal cancer, a prevalent GI malignancy that continues to exert a significant toll on global health. Despite extensive research and therapeutic interventions, colorectal cancer remains a leading cause of cancer-related deaths worldwide [5].
The limitations of existing treatments highlight the pressing need for innovative and more effective therapeutic strategies. Responding to this imperative, attention has turned towards natural compounds with potential therapeutic benefits [6]. One compound that has emerged in the spotlight is quercetin, a polyphenolic flavonoid abundantly found in fruits, vegetables and certain beverages [7]. Quercetin has garnered attention not only for its antioxidant and anti-inflammatory properties but also for its intriguing anticancer potential, making it a subject of intense scientific investigation [8]. Extensive preclinical research has explored the potential of quercetin in inhibiting the growth of cancer cells, inducing apoptosis and suppressing the development of new blood vessels that support tumour growth [9]. Importantly, quercetin has been reported to enhance the efficacy of conventional chemotherapy agents when combined, potentially overcoming resistance mechanisms [10].
The appeal of quercetin lies not only in its anticancer properties but also in its favourable safety profile. Unlike some conventional cancer treatments associated with significant toxicities, quercetin has demonstrated low toxicity in various preclinical and clinical studies [11]. This characteristic is particularly crucial in cancer treatment where minimizing adverse effects on normal tissues is paramount for improving patients’ quality of life during and after therapy [11]. In addition to its direct effects on cancer cells, quercetin has been investigated for its potential to modulate the tumour microenvironment, which plays a critical role in cancer progression. Interventions that can modify this milieu hold promise for disrupting the supportive conditions for tumour growth and metastasis [12]. Quercetin’s ability to influence the tumour microenvironment further expands its potential therapeutic impact. Its molecular structure, depicted in Figure 1, lays the foundation for understanding its multifaceted interactions within biological systems. Its chemical composition contributes to its ability to modulate cellular pathways, making it an intriguing candidate for cancer therapy [13].
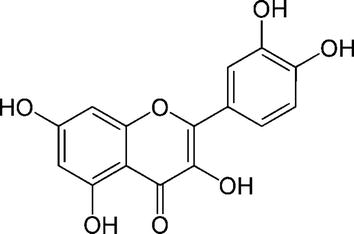
Figure 1
Chemical structure of quercetin.
This chapter aims to comprehensively review and analyse the preclinical therapeutic effects of quercetin against GI cancers, shedding light on its potential as a therapeutic agent. We seek to provide a thorough overview of quercetin’s biosafety and toxicity profiles, ensuring a nuanced understanding of its safety considerations, essential for assessing its potential as a therapeutic agent. Then, exploring quercetin’s pharmacokinetics will elucidate its journey from laboratory studies to potential clinical applications. Understanding the pharmacokinetics of quercetin is crucial, and this section will encompass
Pursuing novel and effective therapeutic strategies for GI cancers is imperative, given the persistent challenges associated with current treatments. With its multifaceted properties and promising preclinical data, quercetin represents a beacon of hope in the quest for innovative cancer therapies. As research advances and clinical trials progress, the true potential of quercetin in reshaping the landscape of GI cancer treatment may unfold, offering new avenues for improving patient outcomes and addressing the unmet needs in cancer care.
2. Biosafety and toxicity profiles of quercetin
Quercetin possesses a variety of pharmacological activities, and for further medical application, it is important to evaluate its biosafety and toxicity profiles in experimental and clinical studies. With oral administration to mice and rats, quercetin consistently did not induce any significant changes in several mutagenicity/genotoxicity endpoints, such as micronuclei, chromosomal aberrations, sister chromatid exchange, unscheduled DNA synthesis and alkali-labile DNA damage in somatic cells [14]. Furthermore, based on the Ames test and combined
On the other hand, a clinical study [16] indicated that total sperm motility was significantly inhibited following exposure to 100, 200 and 400 μM quercetin for 6 and 12 hours in a dose-dependent manner, as compared to the controls (
3. Pharmacokinetics of quercetin
Quercetin exhibits established pharmacokinetic characteristics in both laboratory animals and humans. The previous research finding suggested that unmodified quercetin material has limited bioavailability when administered orally, restricting its practical application in clinical settings. For example, quercetin is consumed in the glycoside form, with glycosyl groups being liberated during chewing, digestion and absorption. Subsequently, the enzymes known as
As of now, the bioavailability of quercetin glycosides has been investigated in several human studies. For example, a previous clinical study reported pharmacokinetic characteristics of corvitin (quercetin) in healthy participants. The study shows that the primary metabolites of quercetin are its methoxy derivatives, specifically isorhamnetin and tamarixetin, together with their conjugates of glucurone and sulfatide. About 20% of quercetin dosages are subject to metabolic changes in 20 minutes, free quercetin conjugates by 33% and isorhamnetin by 70% in 20–30 minutes. Corvitin is eliminated with urine as conjugates of quercetin and isorhamnetin, with an average maximal clearance rate of 1.39 mg/h occurring 1.63 hours after drug administration [22]. Hollman et al. reported that patients with ileostomy exhibited a higher absorption percentage of quercetin glycosides from onions compared to the pure aglycone form [23].
On the other hand, Mansour et al. reported that individuals who had eaten an onion-containing lunch had ileostomy fluid that included considerable levels of aglycone. A significant amount of quercetin aglycone and a small amount of quercetin glycosides were found in the fluid. One conceivable reason is that
Moreover, a study reported the pharmacokinetic characteristics of intravenous administration of quercetin in cancer patients at dosages ranging from 60 to 2000 mg/m2. The researchers determined that the safe amount of quercetin was 945 mg/m2, but the hazardous level was reported to produce nephrotoxicity, hypertension, vomiting and a drop in blood potassium. Intravenous quercetin was discovered to have the following half-lives: 3.8–86 minutes for its elimination half-life and 0.7–7.8 minutes for its distribution; 0.23–0.84 L/min/m2 for its clearance and 3.7 L/m2 for its distribution volume [26]. Following that, experimental research conducted on rabbits showed that the oral and parenteral treatment of quercetin along with pectin enhanced GI absorption by about 11 times and bioavailability by about 10 times compared to quercetin alone. According to the study, quercetin’s pharmacokinetic characteristics and solubility are improved when combined with pectin for medicinal purposes [20]. Subsequent research conducted by Salehi et al. revealed quercetin’s pharmacokinetic properties, indicating that its Tmax and Cmax were 0.7 ± 0.3 hour and 2.3 ± 1.5 μg/ml, respectively, at a dose of up to 200 mg [8].
4. Preclinical therapeutic effects and associated molecular mechanisms of quercetin against gastrointestinal cancers
Gastrointestinal (GI) cancers refer to cancers occurring in the GI tract, including liver and pancreatic cancers. The most common types of GI cancers are colorectal, gastric, liver, oesophageal and pancreatic cancers [27]. According to a report in 2020, GI cancers were among the top 10 most common cancers occurring worldwide. Colorectal cancer ranks 3rd in occurrences and 2nd in mortality, gastric and liver cancers ranked 5th in incidences, while oesophageal cancers ranked 7th in incidences [3].
Gastric cancers arise from malignancies of the stomach; there are two classifications of gastric cancers depending on the topographical subsite of the tumour, which are cardia and non-cardia gastric cancers, referring to the upper stomach and the lower stomach, respectively [3, 27]. Both of these subtypes present with different risk factors, carcinogenesis and epidemiology. For example, non-cardia gastric cancers are primarily attributed to
Liver cancers were reported to be more prevalent in men than in women, with rates of both incidences and mortality being two and three times higher in men than in women [3]. Several more common types of liver cancers include hepatocellular carcinoma (HCC) and intrahepatic cholangiocarcinoma (ICC). Common risk factors of HCC include Hepatitis B virus (HBV) and Hepatitis C virus (HCV) infections, aflatoxins, heavy alcohol intake, obesity, type 2 diabetes and use of tobacco [3, 27]. Meanwhile, incidences of ICC have been linked to primary sclerosing cholangitis (PSC), liver fluke infections, fibropolycystic liver disease and biliary stones [27, 30].
Colorectal cancer was observed to be one of the most common cancers in incidences, ranking 3rd overall, with 2nd in terms of mortality. One observed trend in colorectal cancer is the shift in socioeconomic development, with incidence rates higher in transitioned countries than transitioning countries [3]. Changes in lifestyle, such as increased meat consumption, reduced physical activity and excess body weight, may be among the risk factors for colorectal cancer [31]. Other risk factors include increased alcohol intake, heavy tobacco use and increased consumption of processed or red meats [3].
Pancreatic cancer was reported to be the 7th leading cause of cancer death in males and females, largely due to its poor prognosis. Incidence rates were up to 4- to 5-fold higher in developed countries, with the highest incidences recorded in Europe, North America and Australia/New Zealand [3]. Risk factors of pancreatic cancer include obesity, diabetes and heavy alcohol consumption [27].
Oesophageal cancer ranks 7th in terms of incidence and 6th in mortality worldwide. The most common subtypes of oesophageal cancer are oesophageal squamous cell carcinoma (ESCC) and oesophageal adenocarcinoma (EAC). The geographical distribution of the subtypes may signify the corresponding risk factors associated with the type of cancer. ESCC was more prevalent in Eastern Asia (largely in China), Southern Africa and Eastern Africa. Possible risk factors may include nutritional deficiencies and exposure to nitrosamines [32]. However, other risk factors include heavy tobacco and alcohol use. While EAC was reported to be more prevalent in Western countries. Incidences in high-income countries were attributed to excess body weight and incidences of gastroesophageal reflux disorder [3].
With the common occurrence of GI cancers, developing new strategies to combat and manage GI cancers can be advantageous. Using natural products in medicine has also been a well-known strategy in drug discovery. Examples of common natural products in cancer treatment are taxanes, vinca alkaloids and anthracyclines [33]. Quercetin is a flavonoid found in most fruits and vegetables, possibly one of the most common dietary flavonols [34]. Quercetin can exert antioxidant effects at low doses, which provide a chemopreventive effect, yet also exert a pro-oxidant effect at higher doses, providing a chemotherapeutic effect [35].
4.1 Gastric cancer
Quercetin’s chemopreventive effects, demonstrated in both
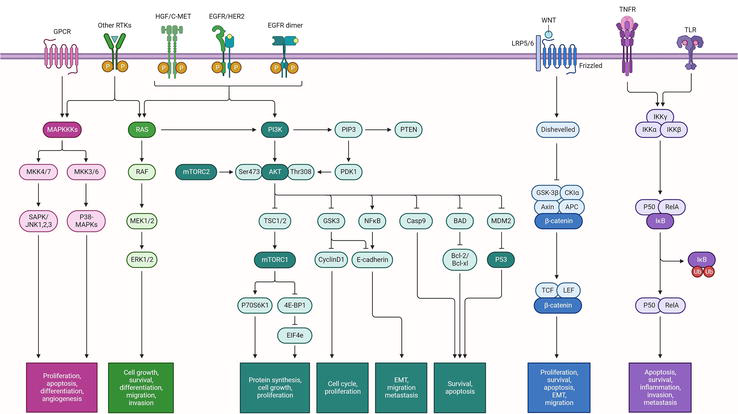
Figure 2
Signalling pathway in gastric cancer.
Zeng et al. conducted a bioinformatics analysis to assess the impact of quercetin on gastric cancer. The study aimed to discover the specific targets of quercetin in NCI-N87 gastric cancer cells exposed to this compound. The researchers exposed human gastric cancer cells (NCI-N87) to a concentration of 15 μM quercetin for 48 hours, using dimethyl sulphoxide (DMSO) as a control. The HiSeq 2500 DNA sequence data were utilized to compare differentially expressed genes (DEGs) between groups. A sophisticated technique was employed to evaluate the protein-protein interaction (PPI) network. The regulatory network of transcription factors (TFs)-DEGs was determined using Cytoscape. The DEGs identified in the study were Fos proto-oncogene (FOS, degree = 12), aryl hydrocarbon receptor (AHR, degree = 12), Jun proto-oncogene (JUN, degree = 11) and cytochrome P450 family 1 subfamily A member 1 (CYP1A1, degree = 11). These DEGs showed significant associations with other proteins in the protein-protein interaction (PPI) network, particularly with proteins having higher degrees. The expression levels of Early growth response 1 (EGR1), FOS like 1 (FOSL1), FOS and JUN were elevated among the five TFs-DEGs, while AHR was downregulated. The Wnt signalling pathway exhibited an abundance of FOSL1, JUN and Wnt family member 7B (WNT7B). CYP1A1 exhibited a strong association with AHR in the PPI network. Thus, quercetin may have specifically affected FOS, AHR, JUN, CYP1A1, EGR1, FOSL1 and WNT7B in gastric cancer [36].
Apart from that, quercetin has also been investigated in combination studies. In a study conducted by Lei et al., the effectiveness of a combination of quercetin and irinotecan in mitigating the spread of gastric cancer was examined. This investigation was done by assessing the expression of genes and proteins [37]. This study compared the effects on β-catenin expression, cell viability and apoptosis of low-dose SN-38 (irinotecan metabolite) in combination with quercetin and high-dose SN-38 alone. In addition, the effects of quercetin and low-dose irinotecan on gastric cancer metastasis were examined using
A distinct investigation was conducted to assess the mechanism and impact of quercetin on the spread of gastric cancer. Additionally, the study aimed to determine if the involvement of urokinase plasminogen activator and urokinase plasminogen receptor (uPA/uPAR) played a role in this process [38]. The purpose of the study was to determine whether quercetin could have an impact on the uPA/uPAR system, which is crucial to gastric cancer metastasis. Precancerous tissues’ uPA and uPAR activity levels were measured, and the results were compared to the migration and invasion patterns of various gastric cancer cell lines [37]. According to the data, uPA and uPAR activities in precancerous tissues were lower than those in gastric cancer cells, and there was a correlation between uPAR expression and gastric cancer cell line migration and invasion. After gastric cancer BGC823 and AGS cells were treated with quercetin (10 μM for 72 hours), uPA and uPAR protein expression levels were decreased along with migration and invasion. The combination of quercetin and uPAR knockdown reduced in matrix metalloproteinase-9 (MMP-9) and matrix metalloproteinase-2 (MMP-2) activities, effectively inhibiting p21-activated kinase 1 (Pak1)-LIM kinase 1 (Limk1)-cofilin signalling. Treatment with quercetin suppressed the activation of adenosine monophosphate (AMP)-activated protein kinase α (AMPKα), nuclear factor-kappa B (NF-κB), extracellular signal-regulated kinase 1/2 (ERK1/2) and protein kinase C-delta (PKC-δ), decreasing uPA and uPAR expression. Ultimately, quercetin can potentially turn out to be an emerging factor in the treatment of gastric cancer, effectively diminishing metastasis and invasion [38].
Furthermore,
The impact of anti-proliferative drugs (doxorubicin (DOX) and quercetin) and small interfering RNA (si-RNA) directed against CDC20 (cell division cycle protein 20 homolog) on GC was assessed by Hemati et al. The researchers examined the use of niosome-encapsulated delivery vehicles to deliver si-RNA and medicines. It was discovered that the administration of si-RNA, together with anticancer medications, resulted in the suppression of CDC20 expression, hence enhancing the effectiveness of gastric cancer treatment [40]. In order to enhance the loading capacity and improve the physicochemical properties of si-RNA, the researchers altered the amount of cationic lipid in cationic PEGylated niosomes. Quercetin, DOX and anti-CDC20 si-RNA were incorporated into the co-delivery system. The system was then evaluated for its physicochemical qualities, controlled release, thermosensitivity, rates of apoptosis and gene silencing efficacy. Interestingly, the data showed that the co-delivery system, intended explicitly for loading si-RNA, had a suitable and significant positive charge for delivering drugs. In addition, the researchers demonstrated a thermosensitive drug release pattern that effectively suppressed CDC20 expression, surpassing the individual delivery of either si-RNA or the drug alone. Additionally, their approach efficiently suppressed the proliferation of gastric cancer cells. Their findings indicated that PEGylated niosomes containing both CDC20 si-RNA and anticancer medicines could serve as an innovative approach for treating gastric cancer [40]. A few studies on quercetin’s therapeutic effects in gastric cancer are listed in Table 1.
Gastrointestinal cancer | Preclinical model ( | Anticancer mode | Molecular mechanism | Reference |
---|---|---|---|---|
Gastric cancer | Anti-proliferative effects | EGR1, FOS-1, FOS and JUN expression levels were elevated while AHR was downregulated. | [36] | |
Inhibition on cell viability, anti-apoptosis and anti-proliferation of gastric cancer metastasis | The combination of quercetin and a low dose of SN-38 reduced β-catenin protein levels in AGS cells compared to quercetin alone. | [37] | ||
Anti-proliferation of gastric cancer metastasis | Inhibition of uPA, uPAR and downstream target expression | [38] | ||
Apoptosis induction | Apoptosis induction via ROS increased the levels of pro-apoptotic proteins (Bax, Bad and Bid), decreasing the levels of anti-apoptotic proteins (Bcl-x, Bcl-2 and Mcl-1) | [39] | ||
Inhibition on gastric cancer cell growth | Suppression of CDC20 by si-RNA which inhibited cancer cell growth | [40] |
Table 1.
4.2 Liver cancer
Fruits, vegetables and plants all contain quercetin having anti-inflammatory, anticancer and antioxidant qualities. Its advantageous properties, including hepatoprotective benefits against liver diseases, have been established in various human illnesses. Hepatocellular carcinoma (HCC) is a primary liver tumour with a high death rate and a delayed diagnosis, which makes it a promising target for research on quercetin effects. HCC is the most prevalent type of primary liver cancer and the sixth most often occurring tumour globally, making it the fourth most deadly neoplasm [41]. Hepatitis C and B virus (HCV and HBV, respectively) and other aetiological agents cause liver damage that leads to the development of HCC through the phases of liver fibrosis and cirrhosis, which can take years or even decades to manifest. Due to its intricate aetiology and molecular heterogeneity, curative therapy for liver cancer is met with challenges [41, 42]. In the first-line scenario for advanced liver cancer, systemic treatment is employed in these instances, employing the two tyrosine kinase inhibitors (TKIs) that are currently available: lenvatinib and sorafenib [43]. After prolonged treatment, liver cancer cells can become resistant to sorafenib, regardless of its efficacy [42], where several TKIs (regorafenib and cabozantinib) and monoclonal antibody have been approved by the FDA for HCC previously treated with sorafenib. Several studies have examined the anticancer benefits of natural substances against liver cancers, such as resveratrol, curcumin and melatonin, to reduce the toxicity and adverse responses brought on by these chemotherapeutic drugs [44].
According to Yamada et al. [45], myricetin and quercetin can block the protein kinase B (AKT) signalling axis, which may subsequently impede HuH7 cell migration driven by transforming growth factor-α (TGF-α) and hepatocyte growth factor (HGF). The study examined how quercetin affected HuH7 cancer cells’ migration in response to TGF-α or HGF. In a dose-dependent manner, quercetin significantly impeded HuH7 cell migration induced by both TGF-α and HGF. Moreover, myricetin, an additional flavonol molecule, significantly inhibited the migration of cancer cells. TGF-α- and HGF-mediated receptor autophosphorylation was unaffected by myricetin or quercetin. Furthermore, quercetin did not affect the TGF-α- or HGF-induced activation of p38 MAPK. However, the growth factors-mediated AKT phosphorylation was inhibited by myricetin and quercetin. Through inhibiting the AKT signalling pathway but not p38 MAPK, their study demonstrated that quercetin might reduce growth factor-driven cell migration of liver cancer [45].
Quercetin can reduce phosphorylation of ERK1/2 and limit proteasome activity, as demonstrated by Ding et al. Proteasome chymotrypsin-like activity rose in response to increasing ERK1/2 activity, while proteasome chymotrypsin-like activity decreased in response to increased MEK1 (mitogen-activated protein kinase kinase 1) activity. The administration of quercetin reduced the amount of proteasome β subunits expressed. According to Ding et al., their investigation demonstrated that the proteasome β subunits’ expression may be modulated by the MEK1/ERK1/2 signalling pathway, resulting in a decrease in the proteasome’s chymotrypsin-like activity [46]. HepG2 cells continued to exhibit steady levels of caspase and trypsin-like protease activity as well as elevated c-Jun N-terminal kinase (JNK) and p38 MAPK activity and reduced phosphorylation of ERK1/2. The inhibition of the JNK and p38 MAPK signalling pathways may not repair the reduced proteasome activity caused by quercetin. Proteasome chymotrypsin-like activity increased or decreased depending on whether MEK1 was upregulated or downregulated. MEK1/ERK1/2 inhibition and quercetin treatment decreased the expression of the proteasome’s β subunits [46].
Quercetin targets apoptosis by upregulating Bax, caspase-3 and p21 and downregulating Akt, polo-like kinase 1 (PLK-1), cyclin-B1, cyclin-A, cell division control 2 (CDC-2), cyclin-dependent kinase 2 (CDK-2) and Bcl-2. It has been observed to decrease signal transducer and activator of transcription 3 (STAT-3) activation and promote STAT-3 protein degradation in liver cancer cells. Numerous studies have demonstrated quercetin’s anticancer properties, suggesting a possible role for the compound in chemoprevention. When quercetin was combined with other anticancer substances, it demonstrated synergistic benefits. Research has demonstrated that quercetin and 5-fluorouracil (5-FU) work synergistically in liver cancer cell lines. Compared to quercetin treatment alone, this combination resulted in increased growth inhibition in some cell lines [47]. Another research found that the anti-inflammatory, proliferative and angiogenesis-related genes TNF-α, VEGF, P53 and NF-κB were all downregulated by quercetin, either alone or in combination with sorafenib, the first medication licensed to treat advanced liver cancer. Treatment with sorafenib plus quercetin resulted in cell cycle arrest, apoptosis and necrosis, in addition to a considerable inhibition of liver tumour growth [48]. Research on the synergistic antitumour effects of quercetin and oncolytic adenovirus in liver cancer cells suggests that quercetin might enhance oncolytic adenovirus ZD55-TRAIL (tumour necrosis factor-related apoptosis-inducing ligand)-mediated growth inhibition and death in liver cancer cells.
These findings point to a distinct anti-proliferative and pro-apoptotic action of quercetin in liver cancer as well as a possible regulating function for the tumour cell cycle progression that requires more research. Through the modification of several cellular processes and signalling pathways, this flavonoid appears to have antitumoural efficaciousness; nevertheless, more research is necessary to fully understand its mechanisms of action against liver cancer. In addition to the emerging usage of quercetin derivatives with anticancer efficaciousness, emerging tactics of combination and drug-delivery systems may enhance such features of cancer inhibition and expand treatment choices for patients with liver cancer [44]. Figure 3 illustrates the common mechanistic pathways of quercetin. A few research on quercetin’s therapeutic benefits in liver cancer are listed in Table 2.
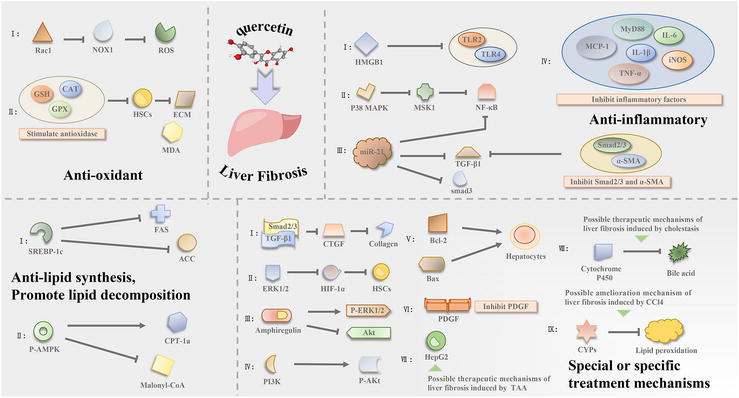
Figure 3
Diagram of the mechanism of quercetin in the treatment of liver fibrosis. Source: Adapted from Ref. [
Gastrointestinal cancer | Preclinical model ( | Anticancer mode | Molecular mechanism | Reference |
---|---|---|---|---|
Liver cancer | Inhibition of cell proliferation | Inhibition of Akt/mTOR, reduced glucose uptake and lactate production, reduced p-Akt/Akt and p-mTOR/mTOR rates, increased cell growth inhibition | [51] | |
Cell growth inhibition, autophagy induction, apoptosis induction | Akt/mTOR inhibition and MAPK activation lead to increase in LC3A/LC3B-II and Beclin1 protein levels and decrease in p62 protein expression, increase in the percentage of apoptotic cells | [52] | ||
Chemosensitizing effect towards 5-FU | FZD7/β-catenin inhibition, reduction in ABCB1, ABCC1 and ABCC2 expression, as well as inhibition of ABCC2 function | [53] | ||
Apoptosis induction | MEK1/ERK1/2 inhibition, increased levels of cleaved caspase-3, cleaved PARP and Bax proteins, reduced levels of Bcl-2 protein | [46] | ||
Decrease of cell proliferation, apoptosis induction | Quercetin combined with ZD55-TRAIL inhibited NF-κB which increased apoptotic activity (nuclear fragmentation, chromatin condensation) as well as apoptotic markers | [49] | ||
Reduced cell viability | Synergistic activity of sorafenib and quercetin, with reduced IC50 of both drugs and increased uptake of both drugs | [54] | ||
Tumour growth inhibition | Quercetin 100 mg/kg was orally administered, reduced in tumour volume, increased necrosis and TUNEL-positive cells | [51] | ||
Quercetin 50 mg/kg administered via intraperitoneal injection, reduction in tumour size, HK2 and Ki67 protein expression and p-Akt and p-mTOR protein levels observed | [51] | |||
Suppression of tumour growth, apoptosis and autophagy induction | Quercetin 60 mg/kg administered orally, reduction in tumour weight and volume observed, as well as apoptotic markers (increased Bax, cleaved caspase-3, reduced Bcl-2 protein expression) | [52] | ||
Suppression of tumour growth and progression, apoptosis induction | Quercetin encapsulation (PLGA-loaded gold-quercetin nanoparticles) 30, 40 and 50 mg/kg via intraperitoneal injection causes inhibition of AP-2β/hTERT, p50/NF-κB/COX-2, Akt/ERK1/2 pathways, reduced tumour weight and volume and increased apoptotic activity observed | [55] | ||
Inhibition of tumour growth | Quercetin combined with dasatinib 50 mg/kg of quercetin with 5 mg/kg of dasatinib administered orally halted tumour progression | [56] |
Table 2
Basic characteristics of
4.3 Colorectal cancer
Quercetin has garnered significant interest in its ability to prevent and combat colorectal cancer [57]. Numerous research have examined these effects both in laboratory settings (
Evaluations have been conducted on the role of quercetin in apoptosis, hyperproliferation and inflammation, as well as its mechanism in 1,2-dimethylhydrazine (DMH)-induced carcinogenicity and tumour multiplicity [65]. Rats were orally given quercetin at doses of 25 or 50 mg/kg body weight and subcutaneously injected with 20 mg/kg body weight of DMH for 15 weeks. Afterwards, the rats were euthanized. The DMH generates reactive oxygen species (specifically superoxide) through the process of membrane lipid peroxidation, leading to a disruption in redox equilibrium. DMH also reduces the amount of antioxidants in tissues. The DMH-induced intestinal carcinogenicity led to an increase in proliferative and inflammatory variables due to a low Bax/Bcl-2 ratio and dysregulation of apoptosis. Pretreatment with quercetin mitigated the deleterious effects of DMH, such as maintaining the activity of detoxifying enzymes and decreasing proliferation as well as early indicators (such as mucin depletion and goblet cell disintegration) in the colonic tissue. Quercetin controlled the expression of β-catenin and adenomatous polyposis coli (APC), decreasing in the occurrence and number of tumours. The histology findings confirm the beneficial effect of quercetin in mitigating the pathogenic changes generated by DMH [65].
A prior investigation similarly assessed the impact of daily intake of microencapsulated probiotics either alone or in conjunction with microencapsulated quercetin to prevent colorectal cancer. The researchers utilized ApcMin/+ mice which exhibit spontaneous intestinal adenomas and cancer. The researchers evaluated changes in tissue structure, intestinal bleeding, fat accumulation, respiratory quotient, body weight and energy usage. In addition, gene expression related to the Wnt signalling pathway was assessed [66]. ApcMin/+ mice were given Bifidobacterium bifidum (Bf) and Lactobacillus gasseri (Lg) probiotic strains, with a concentration of 10 [57] colony-forming units (CFU)/100 g of food or a combination of both probiotic strains together with microencapsulated quercetin at a dosage of 15 mg/100 g of food, for 73 days. Subsequently, energy metabolism, alterations in organ and body weight, histology of colon tissue, composition of intestinal microbiota and gene expression related to the Wnt signalling system were also assessed. The data showed that the microencapsulated supplement, consisting of probiotics and quercetin, effectively inhibited the advancement of colorectal cancer in ApcMin/+ mice [66].
Furthermore, an investigation conducted by Liu and Zhi in 2021 employed a rat model to create constipation, a recognized risk factor for colorectal issues, by providing loperamide. Subsequently, the impact of quercetin on constipation induced by loperamide was examined. The data indicated that administration of quercetin at doses of 25 and 50 mg/kg in rats resulted in an elevation in intestinal transit rate, as well as an increase in the concentration of short-chain fatty acids and levels of gastrin, motilin and substance P. Besides, quercetin enhanced the motility of the intestines and decreased the levels of somatostatin. The levels of aquaporin 3 (AQP3), transient receptor potential vanilloid 1 (TRPV1), glial cell line-derived neurotrophic factor (GDNF), enteric nerve-related factors, nitric oxide (NO) synthase, stem cell factor (SCF) and its receptor c-Kit were measured using Western blotting and reverse transcription-quantitative polymerase chain reaction (RT-qPCR). Quercetin administration was discovered to decrease loperamide-induced constipation by enhancing the levels of interstitial cells of Cajal indicators, such as stem cell factor, its receptor c-Kit and AQP3. Ultimately, their findings demonstrated that quercetin exhibited a safeguarding impact against loperamide-induced constipation, hence potentially mitigating the likelihood of developing colorectal cancer [67].
The antiangiogenic and anticancer effects of quercetin and luteolin on colon cancer cells (HT29) were assessed by Erdoğan et al. in comparison with the conventional chemotherapy drug 5-FU and a combination of 5-FU and luteolin or quercetin. An MTT (3-[4,5-dimethylthiazol-2-yl]-2,5-diphenyl tetrazolium bromide) assay, fluorescence microscopy, human VEGF ELISA (enzyme-linked immunosorbent assay), Western blotting and qRT-PCR were employed. Their method of evaluating the effects was Western blotting on the following genes: mTOR, Akt protein, PTEN, p53, Bax, Bcl-2 and P38. The MTT assay assessed cell viability, and ELISA was utilized to ascertain how the treatment affected angiogenesis. Fluorescence microscopy was employed to identify HT-29 cell apoptosis. Eight times as much apoptosis was induced in cells treated with quercetin and 5-FU as in cells treated with luteolin and 5-FU, and 10 times more in cells treated with the combination. The VEGF level exhibited a notable decrease in cells subjected to a combination treatment of quercetin or luteolin along with 5-FU. The researchers discovered that quercetin and luteolin can govern programmed cell death (apoptosis) in HT-29 cells. Additionally, it was observed that combination therapy decreased the expression of anti-apoptotic proteins, such as Bcl-2, mTOR and Akt genes, as compared to the control group. The groups treated with 5-FU and quercetin exhibited a more rapid rise in the expression of P53, P38, MPK and PTEN genes compared to those treated with 5-FU and luteolin. To summarize, the combined use of luteolin and quercetin can enhance the anticancer properties of 5-FU and mitigate its harmful side effects in colorectal cancer [68]. Figure 4 shows signalling transduction pathways in colon commonly modulated by quercetin.
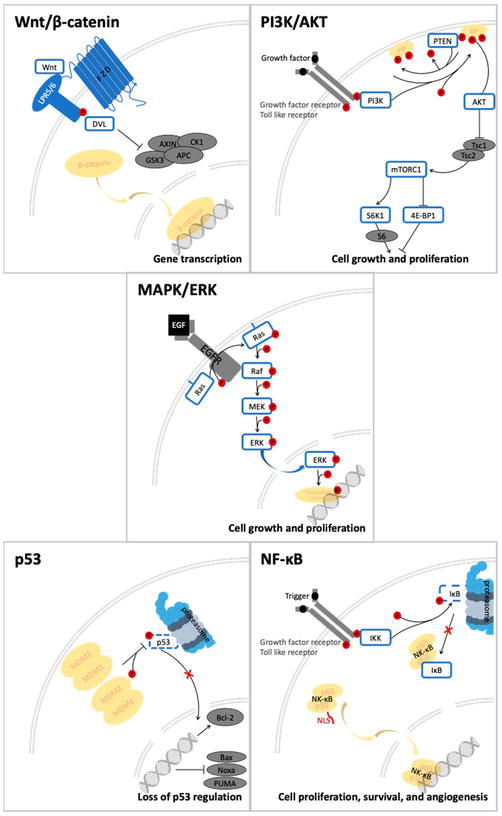
Figure 4
Signalling transduction pathway in colorectal cancer that being modulated by therapeutic approaches of quercetin. Source: Adaptation by Ref. [
Al-Ghamdi et al. examined the mechanism and impact of a 200 mg dosage of quercetin and a 150 mg dose of EGCG (epigallocatechin gallate) at different ratios on the stimulation of programmed cell death and suppression of growth in the human colorectal cell line HCT-116 [70]. Quercetin suppressed cell proliferation, halted the cell cycle, induced annexin V and decreased clonogenicity. The lowest dose of the investigated medicines suppressed colony development. Moreover, a substantial rise in annexin V was observed at 150 mg of quercetin and 100 mg of EGCG. The combination therapy resulted in the cessation of cell division at the G1 phase. Ultimately, the combination of EGCG and quercetin holds promise as a potent combination of chemotherapy in the future. However, further investigations are necessary to determine the appropriate dosage and potential adverse reactions [70]. Table 3 lists some studies on the therapeutic effect of quercetin in colorectal cancer.
Gastrointestinal cancer | Preclinical model ( | Anticancer mode | Molecular mechanism | Reference |
---|---|---|---|---|
Colorectal cancer | Anti-apoptotic, anti-hyperproliferation | Quercetin reduced tumour occurrence and number by controlling β-catenin and APC expression. Histology confirmed quercetin’s beneficial effect in reducing DMH-induced pathogenic changes. | [65] | |
— | Probiotics and quercetin-containing microencapsulated supplementation successfully prevented colorectal cancer in ApcMin/+ mice from progressing via alteration of Wnt signalling | [66] | ||
— | Results showed that quercetin had a protective effect against constipation brought on by loperamide, which may lessen the risk of colorectal cancer by increasing intestinal transit rate and motility and reducing somatostatin levels | [67] | ||
Anti-angiogenic and anti-apoptotic | Treatment with 5-FU and quercetin increased expression levels of P53, P38, MPK and PTEN genes | [68] | ||
Anti-proliferation, inhibition on cell cycle and suppression of growth | Halted cell division at G1 phase | [69] | ||
Apoptosis, anti-angiogenesis and anti-proliferation | Induced apoptosis, anti-angiogenesis and anti-proliferation via EGFR, Akt, Cdk1, cyclin B and VEGF alteration | [63] | ||
Apoptosis and antioxidant | Apoptosis induction via TNF-α and TNF-R1 alteration | [71] | ||
Apoptosis | Apoptosis induction via KRAS, JNK and caspase-3 alteration | [72] | ||
Lipid peroxidation | Inhibition of lipid peroxidation via Nrf-2 and Prx-6 protein | [73] |
Table 3
Summary on the latest studies on the therapeutic effect of quercetin in colorectal cancer.
4.4 Pancreatic cancer
The plant-derived medication presents a compelling option for treating cancer. Quercetin has been demonstrated that quercetin modulates several dysregulated signalling pathways, including those linked to autophagy and apoptosis, in order to achieve its anticancer effects through a variety of methods. In particular, quercetin inhibits multiple signalling pathways, including NF-κB, P53, Wnt/β-catenin, MAPK, janus kinase/signal transducer and activator of transcription (JAK/STAT) and the Hedgehog pathway, to achieve its anticancer actions. Numerous intracellular signalling molecules, including tumour necrosis factor-α (TNF-α), Bax, Bcl-2, caspases and VEGF, are interfered by quercetin.
Numerous cancer types, including breast, prostate, ovarian, lung, colon, hepatocellular, lymphoma and pancreatic cancer, have been researched in relation to quercetin’s anticancer properties. Nevertheless, most current research on quercetin’s anticancer effects focus on cancer in laboratory animals.
According to a recent study on the impact of quercetin on pancreatic cancer, quercetin treatment altered the expression of 105 miRNAs, including the lethal-7 (let-7), miR-194, miR-103, miR-29, miR-125 and miR-106 families. These miRNAs are essential for inhibiting cell death and promoting invasion, proliferation and metastasis. Among these, let-7c is one of the most significant miRNAs. This miRNA controls Notch inhibitor Numbl, a downstream target of let-7c, following transcription. By intensifying its hostility towards Notch, the Numbl stops the spread of cancer [74].
Quercetin is one of the natural plant extracts that have various important biological antitumour properties. Besides, quercetin has been shown to exert its anticancer properties by targeting noncoding RNAs (ncRNAs) that include long non-coding RNA (lncRNA) and microRNA (miRNA), which have been linked to the development of cancer. It inhibits the proliferation of cancer cells, encourages cell death and increases susceptibility to chemotherapy drugs by controlling the production of ncRNAs, which in turn influences the expression of genes and proteins involved in the signalling cascade. These provide insight into the molecular relationship between quercetin and non-coding RNAs, which may be useful in using quercetin as a therapeutic adjuvant. When combined, quercetin is a naturally occurring substance that may have an anticancer impact when used as a cancer adjuvant [9].
Quercetin specifically causes death in pancreatic cancer cells to have an anticancer impact. Still, new research also suggests that quercetin modifies many signal transduction pathways to slow down the growth of cancer. Quercetin may prevent EMT, invasion and metastasis by suppressing the expression of the N-cadherin, MMP-9 and STAT-3 signalling pathways. Through its inhibitory influence on Receptor for Advanced Glycation Endproducts (RAGE) expression, quercetin increases the chemosensitivity of pancreatic cancer cells to gemcitabine (GEM). Meanwhile, it is a desirable agent for the treatment of cancer due to its broad accessibility, effectiveness and low toxicity when compared to other investigated substances. Recently, quercetin has been made available and used as a potentially effective medication to treat a variety of malignancies, either by itself or in conjunction with other chemotherapeutic drugs [75].
Guo et al. employed various methods such as a nude mice tumour formation test, migration, proliferation and invasion as well as real-time cell analysis, to assess the effects of quercetin on pancreatic ductal adenocarcinoma (PDA). The development and spread of tumours as well as the
Hassan et al. assessed the effectiveness of quercetin in conjunction with well-known medications such as gemcitabine (GEM) and doxorubicin (DOX) against human pancreatic cancer cells and hepatic cancer cells, respectively. The investigation revealed that quercetin combined anticancer medications produced superior outcomes than single-drug therapy. Up to 60% of the cells in two-dimensional (2D) and three-dimensional (3D) cultures were dead, with single-drug treatment only exerting ≤20% apoptosis [78]. A more thorough analysis showed that quercetin downregulated hypoxia-inducible factor (HIF)-1α and raised p53 regulator levels, which led to p53-mediated apoptosis. The impact of quercetin on hypoxia and drug efflux pump function was also assessed by investigating the functions of HIF-1α and multidrug transporters (such as multidrug resistance (MDR)) activity [78]. The investigation revealed that quercetin could reduce multidrug resistance mutation 1 (MDR-1) efflux activity, exerting similar inhibitory effects as verapamil, the positive control used in the study. Hence, quercetin might be used with GEM or DOX against multidrug-resistant malignancies in the pancreas and liver, respectively [78].
In another investigation, Liu et al. assessed quercetin’s effects and mode of action on pancreatic cancer cells resistant to GEM. Two cell lines from hepatocellular and pancreatic cancers were used in this investigation. Liver cancer cell lines used were HepG2 and Huh-7, whereas BxPC-3 and PANC-1 were the pancreatic cancer cell lines. Quercetin was reported to have a cytotoxic effect on HepG2 and PANC-1 (GEM-resistant) using a proliferation test and a pro-apoptotic effect on HepG2 and PANC-1, according to a flow cytometry analysis. Western blotting results showed that quercetin caused apoptosis by upregulating p53 protein and downregulating cyclin-D1. Additionally, it resulted in S phase cell cycle arrest. Ultimately, the findings demonstrated that quercetin can be used with established anticancer medications to treat GEM-resistant liver and pancreatic cancer [79].
Hoca et al. investigated the effects of quercetin and resveratrol on the epithelial-mesenchymal transition (EMT) in CD133-positive and CD133-negative pancreatic cancer cells [80]. After separating the CD133+ cancer stem cells from PANC-1 cells using the MiniMACS technique, quercetin and resveratrol were added in varying amounts to the three cell variants: CD133+, CD133− and PANC-1. The investigation employed MTT test and immunocytochemistry with antibodies against vimentin, TNF-α, actin alpha 2 (ACTA-2), N-cadherin and interleukin-1β (IL-1β). The intensity of N-cadherin, ACTA-2 and IL-1β staining was lower in the quercetin-treated cells compared to CD133 + cells treated with resveratrol. Hence, quercetin is effective in significantly reducing N-cadherin expression, preventing EMT which leads to metastasis [80].
In another investigation by Serri et al., gemcitabine and quercetin combination showed synergistic activity, particularly in inhibiting the migration of cancer cells. The study employs hyaluronic acid-coated nanoparticles (PPHA NPs) carrying GEM and quercetin [81]. The nanoparticle carriers were able to improve drug delivery, thereby improving cytotoxicity and cellular uptake; it was also mentioned that the PPHA NPs improved the anti-inflammatory properties of quercetin, observed by a reduction in interleukin (IL) levels in pancreatic ductal adenocarcinoma cell lines Mia-PaCa-2 and PANC-1 [81].
A few research on quercetin’s potential therapeutic benefits for pancreatic cancer are included in Table 4.
Gastrointestinal cancer | Preclinical model ( | Anticancer mode | Molecular mechanism | Reference |
---|---|---|---|---|
Pancreatic cancer | Inhibition of metastasis | EMT suppression by reducing TGF- | [76] | |
Synergism between gemcitabine and quercetin | Reduced IL-6 and IL-8 expressions and enhanced cytotoxicity against Mia-PaCa-2 and PANC-1 cell lines | [81] | ||
Inhibition of metastasis | Reduced immunoreactivities, such as ACTA-2, IL-1 | [82] | ||
Halting tumour progression | Improved effects of BET inhibitors at suppressing tumour development and reduced hnRNPA1 | [83] | ||
Apoptosis induction, autophagy, chemosensitizing effect | Quercetin showed a RAGE silencing like effect that attenuates RAGE expression to accelerate apoptosis, autophagy and chemosensitivity of MIA Paca-2GEMR cells | [84] |
Table 4
Anticancer effects of quercetin against pancreatic cancer.
4.5 Oesophageal cancer
Yue Liu et al. evaluated the effects of quercetin on the angiogenesis and migration of oesophageal cancer cells, in addition to the underlying mechanism [85]. In their study, human oesophageal cancer cells (Eca109) received 5 or 10 μg/ml of quercetin. A scratch wound healing assay evaluated cell migration and invasion was examined using a transwell assay, and a colony formation assay was conducted. Human umbilical vein vascular endothelial cells (CRL-1730) were inoculated in Eca109 conditioned medium, and the effects of quercetin were measured by tube formation and wound healing assays. Western blotting measured MMP-2, MMP-9 and VEGF-A protein expression levels. Quercetin (10 μg/ml) decreased colony formation in Eca109 cells; however, no difference was observed between the control group and the 5 μg/ml quercetin group. The group treated with 10 μg/ml quercetin showed reduced cell migration and invasion, while 5 μg/ml only suppressed invasion. Tube formation ability and endothelial cell migration were inhibited in cells incubated in Eca109 conditioned medium. The group treated with 10 μg/ml quercetin showed reduced MMP-2, MMP-9 and VEGF-A expression levels [85].
Interesting study by Li et al. involving Yishen Qutong Granules (YSQTG) in traditional Chinese medicine showed that quercetin (the main component of YSQTQ) could inhibit proliferation, invasion and clonal formation of oesophageal carcinoma cell lines [86]. Compared with the control group, quercetin at different concentrations of 50, 100, 150 and 200 μmol/L inhibited the proliferation of KYSE30 and KYSE150 cells at 24, 48 and 72 hours. Further study on the effects of quercetin on the formation of cell clonal ability showed that the number of clones formed in KYSE30 cells in the control and 50 and 100 μmol/L quercetin groups was 346 ± 7, 203 ± 10 and 132 ± 5, respectively, while the number of clones formed in KYSE150 cells in the control and 50 and 100 μmol/L quercetin groups was 470 ± 13, 364 ± 5 and 225 ± 15, respectively, the difference was statistically significant (
According to a study by Wang et al., quercetin treatment significantly slowed down the proliferation of oesophageal cancer cells by increasing the rate of apoptosis shown in the flow cytometry detection test (
Gastrointestinal cancer | Preclinical model ( | Anticancer mode | Molecular mechanism | Reference |
---|---|---|---|---|
Oesophageal cancer | Inhibition of invasion and angiogenesis | Reduction levels of MMP-2, MMP-9 and VEGF-A | [85] | |
Inhibition of proliferation, invasion and clonal formation | affect the metabolic pathway, MAPK signalling pathway and nuclear factor-kappa B (NF-κB) signalling pathway | [86] | ||
Induction of apoptosis | Inducing miR-1-3p level and suppressing TAGLN2 level | [87] |
Table 5
Molecular mechanisms of quercetin on oesophageal cancer.
5. Conclusion and future perspectives
The use of quercetin in anticancer management has been proved to have a plethora of applications. Based on the above-mentioned evidences, quercetin makes a promising anticancer agent by targeting cancer cell proliferation, metastasis, angiogenesis and apoptosis directly on cancers of the GI tract. Furthermore, quercetin also prevents cancer progression by exerting chemopreventive effects via anti-oxidative properties. Various
Acknowledgments
This work was supported by the Deanship of Scientific Research, Vice Presidency of Graduate Studies and Scientific Research, King Faisal University, Saudi Arabia (Grant No. 3767) and Sunway University research grants (project no. GRTIN-IGS(02)-CVVR-13-2023.
References
- 1.
Ferlay J et al. Estimating the global cancer incidence and mortality in 2018: GLOBOCAN sources and methods. International Journal of Cancer. 2019; 144 (8):1941-1953. DOI: 10.1002/IJC.31937 - 2.
Bray F, Ferlay J, Soerjomataram I, Siegel RL, Torre LA, Jemal A. Global cancer statistics 2018: GLOBOCAN estimates of incidence and mortality worldwide for 36 cancers in 185 countries. CA: A Cancer Journal for Clinicians. 2018; 68 (6):394-424. DOI: 10.3322/CAAC.21492 - 3.
Sung H et al. Global cancer statistics 2020: GLOBOCAN estimates of incidence and mortality worldwide for 36 cancers in 185 countries. CA: A Cancer Journal for Clinicians. 2021; 71 (3):209-249. DOI: 10.3322/CAAC.21660 - 4.
Baskar R, Lee KA, Yeo R, Yeoh KW. Cancer and radiation therapy: Current advances and future directions. International Journal of Medical Sciences. 2012; 9 (3):193-199. DOI: 10.7150/IJMS.3635 - 5.
Hossain MS et al. Colorectal cancer: A review of carcinogenesis, global epidemiology, current challenges, risk factors, preventive and treatment strategies. Cancers (Basel). 2022; 14 (7):1732. DOI: 10.3390/CANCERS14071732 - 6.
Ekor M. The growing use of herbal medicines: Issues relating to adverse reactions and challenges in monitoring safety. Frontiers in Pharmacology. 2014; 4 :177. DOI: 10.3389/FPHAR.2013.00177 - 7.
Anand David AV, Arulmoli R, Parasuraman S. Overviews of biological importance of quercetin: A bioactive flavonoid. Pharmacognosy Reviews. 2016; 10 (20):84-89. DOI: 10.4103/0973-7847.194044 - 8.
Salehi B et al. Therapeutic potential of quercetin: New insights and perspectives for human health. ACS Omega. 2020; 5 (20):11849-11872. DOI: 10.1021/ACSOMEGA.0C01818 - 9.
Asgharian P et al. Potential mechanisms of quercetin in cancer prevention: Focus on cellular and molecular targets. Cancer Cell International. 2022; 22 (1):257. DOI: 10.1186/S12935-022-02677-W - 10.
Niedzwiecki A, Roomi MW, Kalinovsky T, Rath M. Anticancer efficacy of polyphenols and their combinations. Nutrients. 2016; 8 (9):552. DOI: 10.3390/NU8090552 - 11.
Boots AW, Haenen GRMM, Bast A. Health effects of quercetin: From antioxidant to nutraceutical. European Journal of Pharmacology. 2008; 585 (2–3):325-337. DOI: 10.1016/J.EJPHAR.2008.03.008 - 12.
Sharma P, Hu-Lieskovan S, Wargo JA, Ribas A. Primary, adaptive, and acquired resistance to cancer immunotherapy. Cell. 2017; 168 (4):707-723. DOI: 10.1016/J.CELL.2017.01.017 - 13.
Yang D, Wang T, Long M, Li P. Quercetin: Its main pharmacological activity and potential application in clinical medicine. Oxidative Medicine and Cellular Longevity. 2020; 2020 :8825387. DOI: 10.1155/2020/8825387 - 14.
Wang W et al. The biological activities, chemical stability, metabolism and delivery systems of quercetin: A review. Trends in Food Science and Technology. 2016; 56 :21-38. DOI: 10.1016/J.TIFS.2016.07.004 - 15.
Kapoor MP, Moriwaki M, Timm D, Satomoto K, Minegawa K. Genotoxicity and mutagenicity evaluation of isoquercitrin-γ-cyclodextrin molecular inclusion complex using Ames test and a combined micronucleus and comet assay in rats. The Journal of Toxicological Sciences. 2022; 47 (6):221-235. DOI: 10.2131/JTS.47.221 - 16.
Liang X, Xia Z, Yan J, Wang Y, Xue S, Zhang X. Quercetin inhibits human sperm functions by reducing sperm [Ca2+]i and tyrosine phosphorylation. Pakistan Journal of Pharmaceutical Sciences. 2016; 29 (6 Suppl):2391-2396 - 17.
Ożarowski M et al. Pharmacological effect of quercetin in hypertension and its potential application in pregnancy-induced hypertension: Review of in vitro, in vivo, and clinical studies. Evidence-Based Complementary and Alternative Medicine. 2018; 2018 :7421489. DOI: 10.1155/2018/7421489 - 18.
Di Pierro F et al. Potential clinical benefits of quercetin in the early stage of COVID-19: Results of a second, pilot, randomized, controlled and open-label clinical trial. International Journal of General Medicine. 2021; 14 :2807-2816. DOI: 10.2147/IJGM.S318949 - 19.
Han MK, Barreto TA, Martinez FJ, Comstock AT, Sajjan US. Randomised clinical trial to determine the safety of quercetin supplementation in patients with chronic obstructive pulmonary disease. BMJ Open Respiratory Research. 2020; 7 (1):e000392. DOI: 10.1136/BMJRESP-2018-000392 - 20.
El-Saber Batiha G et al. The pharmacological activity, biochemical properties, and pharmacokinetics of the major natural polyphenolic flavonoid: Quercetin. Food. 2020; 9 (3):374. DOI: 10.3390/FOODS9030374 - 21.
Almeida AF et al. Bioavailability of quercetin in humans with a focus on interindividual variation. Comprehensive Reviews in Food Science and Food Safety. 2018; 17 (3):714-731. DOI: 10.1111/1541-4337.12342 - 22.
Septembre-Malaterre A et al. Focus on the high therapeutic potentials of quercetin and its derivatives. Phytomedicine Plus : International Journal of Phytotherapy and Phytopharmacology. 2022; 2 (1):100220. DOI: 10.1016/J.PHYPLU.2022.100220 - 23.
Dabeek WM, Marra MV. Dietary Quercetin and Kaempferol: Bioavailability and potential cardiovascular-related bioactivity in humans. Nutrients. 2019; 11 (10):2288. DOI: 10.3390/NU11102288 - 24.
Mansour FR, Abdallah IA, Bedair A, Hamed M. Analytical methods for the determination of quercetin and quercetin glycosides in pharmaceuticals and biological samples. Critical Reviews in Analytical Chemistry. 2023:1-26. DOI: 10.1080/10408347.2023.2269421 - 25.
Lesjak M et al. Antioxidant and anti-inflammatory activities of quercetin and its derivatives. Journal of Functional Foods. 2018; 40 :68-75. DOI: 10.1016/J.JFF.2017.10.047 - 26.
Ezzati M, Yousefi B, Velaei K, Safa A. A review on anti-cancer properties of Quercetin in breast cancer. Life Sciences. 2020; 248 :117463. DOI: 10.1016/J.LFS.2020.117463 - 27.
Arnold M et al. Global burden of 5 major types of gastrointestinal cancer. Gastroenterology. 2020; 159 (1):335-349.e15. DOI: 10.1053/J.GASTRO.2020.02.068 - 28.
Plummer M, Franceschi S, Vignat J, Forman D, De Martel C. Global burden of gastric cancer attributable to Helicobacter pylori . International Journal of Cancer. 2015;136 (2):487-490. DOI: 10.1002/IJC.28999 - 29.
Kamangar F et al. Opposing risks of gastric cardia and noncardia gastric adenocarcinomas associated with Helicobacter pylori seropositivity. Journal of the National Cancer Institute. 2006;98 (20):1445-1452. DOI: 10.1093/JNCI/DJJ393 - 30.
Khan SA, Thomas HC, Davidson BR, Taylor-Robinson SD. Cholangiocarcinoma. Lancet. 2005; 366 (9493):1303-1314. DOI: 10.1016/S0140-6736(05)67530-7 - 31.
Siegel RL et al. Colorectal cancer statistics, 2020. CA: A Cancer Journal for Clinicians. 2020; 70 (3):145-164. DOI: 10.3322/CAAC.21601 - 32.
McCormack VA et al. Informing etiologic research priorities for squamous cell esophageal cancer in Africa: A review of setting-specific exposures to known and putative risk factors. International Journal of Cancer. 2017; 140 (2):259-271. DOI: 10.1002/IJC.30292 - 33.
Mali SB. Cancer treatment: Role of natural products. Time to have a serious rethink. Oral Oncology Reports. 2023; 6 :100040. DOI: 10.1016/J.OOR.2023.100040 - 34.
Reyes-Farias M, Carrasco-Pozo C. The anti-cancer effect of quercetin: Molecular implications in cancer metabolism. International Journal of Molecular Sciences. 2019; 20 (13):3177. DOI: 10.3390/IJMS20133177 - 35.
Neuwirthová J, Gál B, Smilek P, Urbánková P. Potential of the flavonoid quercetin to prevent and treat cancer—Current status of research. Klinická Onkologie. 2018; 31 (3):184-190. DOI: 10.14735/AMKO2018184 - 36.
Zeng Y, Shen Z, Gu W, Wu M. Bioinformatics analysis to identify action targets in NCI-N87 gastric cancer cells exposed to quercetin. Pharmaceutical Biology. 2018; 56 (1):393-398. DOI: 10.1080/13880209.2018.1493610 - 37.
Lei CS, Hou YC, Pai MH, Lin MT, Yeh SL. Effects of quercetin combined with anticancer drugs on metastasis-associated factors of gastric cancer cells: In vitro and in vivo studies. The Journal of Nutritional Biochemistry. 2018; 51 :105-113. DOI: 10.1016/J.JNUTBIO.2017.09.011 - 38.
Li H, Chen C. Quercetin has antimetastatic effects on gastric cancer cells via the interruption of uPA/uPAR function by modulating NF-κb, PKC-δ, ERK1/2, and AMPKα. Integrative Cancer Therapies. 2018; 17 (2):511-523. DOI: 10.1177/1534735417696702 - 39.
Shang HS et al. Quercetin induced cell apoptosis and altered gene expression in AGS human gastric cancer cells. Environmental Toxicology. 2018; 33 (11):1168-1181. DOI: 10.1002/TOX.22623 - 40.
Hemati M, Haghiralsadat F, Jafary F, Moosavizadeh S, Moradi A. Targeting cell cycle protein in gastric cancer with CDC20siRNA and anticancer drugs (doxorubicin and quercetin) co-loaded cationic PEGylated nanoniosomes. International Journal of Nanomedicine. 2019; 14 :6575-6585. DOI: 10.2147/IJN.S211844 - 41.
Balogh J et al. Hepatocellular carcinoma: A review. Journal of Hepatocellular Carcinoma. 2016; 3 :41-53. DOI: 10.2147/JHC.S61146 - 42.
Méndez-Blanco C, Fondevila F, García-Palomo A, González-Gallego J, Mauriz JL. Sorafenib resistance in hepatocarcinoma: Role of hypoxia-inducible factors. Experimental & Molecular Medicine. 2018; 50 (10):1-9. DOI: 10.1038/S12276-018-0159-1 - 43.
Fondevila F, Méndez-Blanco C, Fernández-Palanca P, González-Gallego J, Mauriz JL. Anti-tumoral activity of single and combined regorafenib treatments in preclinical models of liver and gastrointestinal cancers. Experimental & Molecular Medicine. 2019; 51 (9):1-15. DOI: 10.1038/S12276-019-0308-1 - 44.
Fernández-Palanca P, Fondevila F, Méndez-Blanco C, Tuñón MJ, González-Gallego J, Mauriz JL. Antitumor effects of quercetin in hepatocarcinoma in vitro and in vivo models: A systematic review. Nutrients. 2019; 11 (12):2875. DOI: 10.3390/NU11122875 - 45.
Yamada N, Matsushima-Nishiwaki R, Kozawa O. Quercetin suppresses the migration of hepatocellular carcinoma cells stimulated by hepatocyte growth factor or transforming growth factor-α: Attenuation of AKT signaling pathway. Archives of Biochemistry and Biophysics. 2020; 682 :108296. DOI: 10.1016/J.ABB.2020.108296 - 46.
Ding Y, Chen X, Wang B, Yu B, Ge J, Shi X. Quercetin suppresses the chymotrypsin-like activity of proteasome via inhibition of MEK1/ERK1/2 signaling pathway in hepatocellular carcinoma HepG2 cells. Canadian Journal of Physiology and Pharmacology. 2018; 96 (5):521-526. DOI: 10.1139/CJPP-2017-0655 - 47.
Hisaka T et al. Quercetin suppresses proliferation of liver cancer cell lines in vitro. Anticancer Research. 2020; 40 (8):4695-4700. DOI: 10.21873/ANTICANRES.14469 - 48.
Abdu S, Juaid N, Amin A, Moulay M, Miled N. Effects of sorafenib and quercetin alone or in combination in treating hepatocellular carcinoma: In vitro and in vivo approaches. Molecules. 2022; 27 (22).DOI: 10.3390/MOLECULES27228082 - 49.
Zou H, Zheng YF, Ge W, Wang SB, Mou XZ. Synergistic anti-tumour effects of quercetin and oncolytic adenovirus expressing TRAIL in human hepatocellular carcinoma. Scientific Reports. 2018; 8 (1):2182. DOI: 10.1038/S41598-018-20213-7 - 50.
Guo X et al. The construction of preclinical evidence for the treatment of liver fibrosis with quercetin: A systematic review and meta-analysis. Phytotherapy Research. 2022; 36 (10):3774-3791. DOI: 10.1002/PTR.7569 - 51.
Wu H et al. Quercetin inhibits the proliferation of glycolysis-addicted HCC cells by reducing hexokinase 2 and Akt-mTOR pathway. Molecules. 2019; 24 (10):1993. DOI: 10.3390/MOLECULES24101993 - 52.
Ji Y et al. Quercetin inhibits growth of hepatocellular carcinoma by apoptosis induction in part via autophagy stimulation in mice. The Journal of Nutritional Biochemistry. 2019; 69 :108-119. DOI: 10.1016/J.JNUTBIO.2019.03.018 - 53.
Chen Z et al. Reversal effect of quercetin on multidrug resistance via FZD7/β-catenin pathway in hepatocellular carcinoma cells. Phytomedicine. 2018; 43 :37-45. DOI: 10.1016/J.PHYMED.2018.03.040 - 54.
Abdelmoneem MA et al. Dual-targeted lactoferrin shell-oily core nanocapsules for synergistic targeted/herbal therapy of hepatocellular carcinoma. ACS Applied Materials & Interfaces. 2019; 11 (30):26731-26744. DOI: 10.1021/ACSAMI.9B10164 - 55.
Ren KW et al. Quercetin nanoparticles display antitumor activity via proliferation inhibition and apoptosis induction in liver cancer cells. International Journal of Oncology. 2017; 50 (4):1299-1311. DOI: 10.3892/IJO.2017.3886 - 56.
Kovacovicova K et al. Senolytic Cocktail Dasatinib+Quercetin (D+Q) does not enhance the efficacy of senescence-inducing chemotherapy in liver cancer. Frontiers in Oncology. 2018; 8 :459. DOI: 10.3389/FONC.2018.00459 - 57.
Bhatiya M, Pathak S, Jothimani G, Duttaroy AK, Banerjee A. A comprehensive study on the anti-cancer effects of quercetin and its epigenetic modifications in arresting progression of colon cancer cell proliferation. Archivum Immunologiae et Therapiae Experimentalis (Warsz). 2023; 71 (1):6. DOI: 10.1007/S00005-023-00669-W - 58.
Zhou W et al. Validation of quercetin in the treatment of colon cancer with diabetes via network pharmacology, molecular dynamics simulations, and in vitro experiments. Molecular Diversity. 2023. DOI: 10.1007/S11030-023-10725-4 - 59.
Inala MSR, Pamidimukkala K. Amalgamation of quercetin with anastrozole and capecitabine: A novel combination to treat breast and colon cancers—An in vitro study. Journal of Cancer Research and Therapeutics. 2023; 19 (Supplement):93-105. DOI: 10.4103/JCRT.JCRT_599_20 - 60.
Lawson MK. Improvement of therapeutic value of quercetin with chitosan nanoparticle delivery systems and potential applications. International Journal of Molecular Sciences. 2023; 24 (4):3293. DOI: 10.3390/IJMS24043293 - 61.
Tezerji S, abdolazimi H, Fallah A, Talaei B. The effect of resveratrol and quercetin intervention on azoxymethane-induced colon cancer in Rats model. Clinical Nutrition Open Science. 2022; 45 :91-102. DOI: 10.1016/J.NUTOS.2022.01.008 - 62.
Czapla J et al. The complex composition of trans-resveratrol, quercetin, vitamin E and selenium inhibits the growth of colorectal carcinoma. Anticancer Research. 2022; 42 (10):4763-4772. DOI: 10.21873/ANTICANRES.15981 - 63.
Rashedi J et al. Anti-tumor effect of quercetin loaded chitosan nanoparticles on induced colon cancer in Wistar rats. Advanced Pharmaceutical Bulletin. 2019; 9 (3):409-415. DOI: 10.15171/APB.2019.048 - 64.
Darband SG et al. Quercetin attenuated oxidative DNA damage through NRF2 signaling pathway in rats with DMH induced colon carcinogenesis. Life Sciences. 2020; 253 :117584. DOI: 10.1016/J.LFS.2020.117584 - 65.
Shree A, Islam J, Sultana S. Quercetin ameliorates reactive oxygen species generation, inflammation, mucus depletion, goblet disintegration, and tumor multiplicity in colon cancer: Probable role of adenomatous polyposis coli, β-catenin. Phytotherapy Research. 2021; 35 (4):2171-2184. DOI: 10.1002/PTR.6969 - 66.
Benito I et al. Microencapsulated Bifidobacterium bifidum andLactobacillus gasseri in combination with quercetin inhibit colorectal cancer development in ApcMin/+ mice. International Journal of Molecular Sciences. 2021;22 (9):4906. DOI: 10.3390/IJMS22094906 - 67.
Liu W, Zhi A. The potential of Quercetin to protect against loperamide-induced constipation in rats. Food Science & Nutrition. 2021; 9 (6):3297-3307. DOI: 10.1002/FSN3.2296 - 68.
Erdoğan MK, Ağca CA, Aşkın H. Quercetin and Luteolin improve the anticancer effects of 5-fluorouracil in human colorectal adenocarcinoma in vitro model: A mechanistic insight. Nutrition and Cancer. 2022; 74 (2):660-676. DOI: 10.1080/01635581.2021.1900301 - 69.
Neamtu AA et al. A comprehensive view on the quercetin impact on colorectal cancer. Molecules. 2022; 27 (6):1873. DOI: 10.3390/MOLECULES27061873 - 70.
Al-Ghamdi MA, Al-Enazy A, Huwait EA, Albukhari A, Harakeh S, Moselhy SS. Enhancement of Annexin V in response to combination of epigallocatechin gallate and quercetin as a potent arrest the cell cycle of colorectal cancer. Brazilian Journal of Biology. 2021; 83 :e248746. DOI: 10.1590/1519-6984.248746 - 71.
Sezer ED, Oktay LM, Karadadaş E, Memmedov H, Selvi Gunel N, Sözmen E. Assessing anticancer potential of blueberry flavonoids, quercetin, kaempferol, and gentisic acid, through oxidative stress and apoptosis parameters on HCT-116 cells. Journal of Medicinal Food. 2019; 22 (11):1118-1126. DOI: 10.1089/JMF.2019.0098 - 72.
Yang Y et al. Quercetin preferentially induces apoptosis in KRAS-mutant colorectal cancer cells via JNK signaling pathways. Cell Biology International. 2019; 43 (2):117-124. DOI: 10.1002/CBIN.11055 - 73.
Morales AM, Mukai R, Murota K, Terao J. Inhibitory effect of catecholic colonic metabolites of rutin on fatty acid hydroperoxide and hemoglobin dependent lipid peroxidation in Caco-2 cells. Journal of Clinical Biochemistry and Nutrition. 2018; 63 (3):175-180. DOI: 10.3164/JCBN.18-38 - 74.
Nwaeburu CC et al. Up-regulation of microRNA let-7c by quercetin inhibits pancreatic cancer progression by activation of Numbl. Oncotarget. 2016; 7 (36):58367-58380. DOI: 10.18632/ONCOTARGET.11122 - 75.
Asgharian P et al. Quercetin impact in pancreatic cancer: An overview on its therapeutic effects. Oxidative Medicine and Cellular Longevity. 2021; 2021 :4393266. DOI: 10.1155/2021/4393266 - 76.
Guo Y et al. Quercetin suppresses pancreatic ductal adenocarcinoma progression via inhibition of SHH and TGF-β/Smad signaling pathways. Cell Biology and Toxicology. 2021; 37 (3):479-496. DOI: 10.1007/S10565-020-09562-0 - 77.
Mirazimi SMA et al. Application of quercetin in the treatment of gastrointestinal cancers. Frontiers in Pharmacology. 2022; 13 :860209. DOI: 10.3389/FPHAR.2022.860209 - 78.
Hassan S et al. Quercetin potentializes the respective cytotoxic activity of gemcitabine or doxorubicin on 3D culture of AsPC-1 or HepG2 cells, through the inhibition of HIF-1α and MDR1. PLoS ONE. 2020; 15 (10):e0240676. DOI: 10.1371/JOURNAL.PONE.0240676 - 79.
Liu ZJ et al. Quercetin induces apoptosis and enhances gemcitabine therapeutic efficacy against gemcitabine-resistant cancer cells. Anti-Cancer Drugs. 2020; 31 (7):684-692. DOI: 10.1097/CAD.0000000000000933 - 80.
Hoca M, Becer E, Kabadayı H, Yücecan S, Vatansever HS. The effect of resveratrol and quercetin on epithelial-mesenchymal transition in pancreatic cancer stem cell. Nutrition and Cancer. 2020; 72 (7):1231-1242. DOI: 10.1080/01635581.2019.1670853 - 81.
Serri C et al. Combination therapy for the treatment of pancreatic cancer through hyaluronic acid-decorated nanoparticles loaded with quercetin and gemcitabine: A preliminary in vitro study. Journal of Cellular Physiology. 2019; 234 (4):4959-4969. DOI: 10.1002/JCP.27297 - 82.
El-kott AF, Shati AA, Ali Al-kahtani M, Alharbi SA. The apoptotic effect of resveratrol in ovarian cancer cells is associated with downregulation of galectin-3 and stimulating miR-424-3p transcription. Journal of Food Biochemistry. 2019; 43 (12):e13072. DOI: 10.1111/JFBC.13072 - 83.
Pham TND et al. Quercetin enhances the anti-tumor effects of BET inhibitors by suppressing hnRNPA1. International Journal of Molecular Sciences. 2019; 20 (17):4293. DOI: 10.3390/IJMS20174293 - 84.
Lan CY, Chen SY, Kuo CW, Lu CC, Yen GC. Quercetin facilitates cell death and chemosensitivity through RAGE/PI3K/AKT/mTOR axis in human pancreatic cancer cells. Journal of Food and Drug Analysis. 2019; 27 (4):887-896. DOI: 10.1016/J.JFDA.2019.07.001 - 85.
Liu Y, Li CL, Xu QQ, Cheng D, Di Liu K, Sun ZQ. Quercetin inhibits invasion and angiogenesis of esophageal cancer cells. Pathology, Research and Practice. 2021; 222 :153455. DOI: 10.1016/J.PRP.2021.153455 - 86.
Li J et al. Network pharmacology and in vitro experimental verification on intervention of quercetin, present in Chinese medicine Yishen Qutong granules, on esophageal cancer. Chinese Journal of Integrative Medicine. 2023; 29 (3):233-243. DOI: 10.1007/S11655-022-3677-6 - 87.
Wang Y, Chen X, Li J, Xia C. Quercetin antagonizes esophagus cancer by modulating miR-1-3p/TAGLN2 pathway-dependent growth and metastasis. Nutrition and Cancer. 2022; 74 (5):1872-1881. DOI: 10.1080/01635581.2021.1972125