Abstract
Chronic, non-healing wounds represent a major clinical challenge with substantial economic burden. Impaired wound healing stems from excessive inflammation, infection, ischemia, and oxidative stress. Molecular hydrogen has recently emerged as a therapeutic medical gas with antioxidant, anti-inflammatory, and cytoprotective properties through selective free radical scavenging, activating antioxidant enzymes, reducing pro-inflammatory cytokines, and potentially modulating cell signaling. Arly preclinical evidence shows hydrogen therapy may promote wound healing through accelerated closure, decreased inflammation, and mitigated tissue injury. Initial small-scale human pilots and case reports demonstrate feasibility and apparent safety of administering hydrogen via inhalation, ingestion, topical application, or baths. However, rigorous clinical trials validating therapeutic efficacy are lacking. Further research should optimize delivery, dose, timing, and tissue bioavailability to translate promising preclinical findings into effective clinical hydrogen treatments for wound care. Definitive large-scale studies are critical next steps.
Keywords
- molecular hydrogen
- wound healing
- oxidative stress
- antioxidant therapy
- inflammation
- hydrogen gas
- hydrogen water
- topical delivery
- medical gases
1. Introduction
Wound healing is a complex physiological process that involves coordinated cellular, molecular, and biochemical events leading to tissue repair and restoration of function after injury. This dynamic process can be categorized into four overlapping phases: hemostasis, inflammation, proliferation, and tissue remodeling. While ideal wound healing results in restored tissue integrity without scar formation, this orchestrated process can be impaired by both intrinsic and extrinsic factors, leading to chronic non-healing wounds [1].
Upon tissue injury, the first response is hemostasis, which stops bleeding and limits blood loss. Vascular constriction induced by localized reflexes and circulating catecholamines achieves initial hemostatic control. This is further reinforced by platelet aggregation and fibrin clot formation, with cross-linked fibrin serving as a temporary matrix [2]. The hemostatic plug also contains various growth factors and cytokines released by degranulating platelets that help initiate the inflammatory phase.
Inflammation begins shortly after injury with the extravasation and chemotaxis of immune cells, particularly neutrophils followed by macrophages, to the wound site. Neutrophils phagocytose and destroy bacteria and debris, while macrophages debride dead tissue and continue phagocytosis in the later stages of inflammation [3]. Macrophages also release a variety of growth factors and cytokines that promote fibroblast proliferation, extracellular matrix synthesis, and angiogenesis. Key pro-inflammatory cytokines released include tumor necrosis factor α (TNF-α), interleukins 1 and 6 (IL-1 and IL-6), and transforming growth factor β (TGF-β) [1].
After approximately 48–72 h, the proliferation phase commences which involves several processes to restore tissue integrity. A provisional extracellular matrix formed during inflammation is enhanced as fibroblasts proliferate and deposit collagen, fibronectin, glycosaminoglycans and proteoglycans [2]. Angiogenesis stimulated by factors like vascular endothelial growth factor (VEGF) supplies the healing tissue with nutrients and oxygen. Keratinocytes also proliferate and migrate across the wound bed to re-epithelialize the surface. The final phase of wound healing is remodeling, which can last for months to years. Matrix metalloproteinases (MMPs) degrade excess collagen to remodel scar tissue and increase tensile strength [4]. Myofibroblasts contract wound edges together while collagen fibers become organized along tension lines. Remodeling gradually returns the injured tissue to a pre-wound architecture and functional state, although the resulting scar tissue only regains approximately 80% of its original strength [5].
While this phased wound healing process allows the efficient repair of injured tissue. Advanced age is associated with delayed healing, likely due to reduced growth factor production and angiogenesis [1]. Wounds in the elderly also exhibit diminished collagen accumulation and remodeling [6]. Obesity can also impair healing through chronic inflammation and vascular dysfunction. Diabetes strongly inhibits healing due to hyperglycemia, reduced growth factors, deficient angiogenesis and collagen accumulation, immune dysfunction, and susceptibility to infection (Figure 1) [7].

Figure 1.
Phases of wound healing as depicted in the given figure. Hemostasis occurs immediately after injury to halt bleeding. Inflammation follows to clean the wound by recruiting immune cells. Proliferation phase involves processes like re-epitheliallization (1), fibroblast proliferation (2), angiogenesis (3), and matrix deposition (4) to cover the wound site. Remodeling then strengthens the wound over months/years via collagen remodeling and apoptosis. This coordinated process effectively closes the wound and restores the epithelial barrier.
Chronic non-healing wounds are also characterized by prolonged inflammation and elevated pro-inflammatory cytokines like TNF-α and IL-1β [8]. This prevents transition to the proliferative phase. Immune dysfunction due to illness, stress or medications like steroids also impedes normal healing. Ionizing radiation generates reactive oxygen species that damage cells and lead to poorly vascularized tissue with abnormal extracellular matrix [9]. Wound infections need to be controlled to allow progression beyond the inflammatory stage. Compromised circulation in conditions like peripheral arterial disease or venous insufficiency results in ischemia and hypoxia that impair healing [1]. Finally, nutritional deficits including protein, vitamins C and A, and zinc can delay healing by inhibiting cell proliferation and collagen synthesis (Figure 2) [10].

Figure 2.
Barriers to wound healing: unraveling the complex factors that inhibit the regenerative journey.
Inflammation is a critical part of the normal wound healing response. During the inflammatory phase, various cytokines, chemokines, and growth factors are released to recruit immune cells like neutrophils and macrophages to the wound [11]. These inflammatory cells defend against infection, debride damaged tissue, and release additional signals to activate tissue repair processes like angiogenesis and fibroplasia. However, uncontrolled inflammation driven by elevated pro-inflammatory cytokines like TNF-α and IL-1 can also impair healing. Chronic wounds often exhibit prolonged inflammation that prevents transition to the proliferative phase [12]. Therefore, inflammation is necessary for healing but its careful regulation is equally important. Reactive oxygen species (ROS) are generated as a consequence of aerobic metabolism and inflammatory cell activation during wound healing. At low concentrations, ROS facilitate defense against microbes and act as signaling molecules to promote cell proliferation and migration [13]. However, excessive ROS damage lipids, proteins, nucleic acids and extracellular matrix components while depleting endogenous antioxidant defenses. This oxidative stress stalls healing by causing cell senescence, apoptosis, and necrosis [14]. Systemic conditions like diabetes and the local wound environment can both contribute to disrupted redox balance. Antioxidants that scavenge ROS and boost endogenous antioxidant capacity may help correct oxidative stress and facilitate wound healing. Topical antioxidants including curcumin, honey, and alpha-tocopherol have shown promise in animal models but clinical evidence in humans remains limited [15]. Other antioxidant therapies like ebselen and zinc have demonstrated efficacy in diabetic wound models but also need further clinical evaluation [16]. A key challenge is effective and sustained antioxidant delivery to heal chronic wounds in patients.
2. Molecular hydrogen
Molecular hydrogen (H2) has recently emerged as a physiological inert gas with antioxidant, anti-inflammatory, and cytoprotective effects in animal models [17]. As the smallest molecule, hydrogen can readily diffuse and accumulate in subcellular compartments to reduce localized oxidative stress. Administering hydrogen in either inhaled gas, dissolved saline or water, or topical formulations may have therapeutic potential for difficult to heal wounds by correcting the underlying redox imbalance. Early preclinical studies have shown beneficial effects of hydrogen in rodent models of skin flap ischemia, burns, radiation injury, and diabetic wounds. However, rigorous clinical investigations are still needed to translate these promising findings with molecular hydrogen to human wound healing.
2.1 Mechanisms of molecular hydrogen
Molecular hydrogen (H2) is a gas consisting of two attached hydrogen atoms. This stable diatomic hydrogen molecule has the lowest molecular weight of 2.02 g/mol and is physiologically inert [18]. As the lightest gas, hydrogen can diffuse rapidly across cell membranes and penetrate tissues. It is slightly soluble in water, with a maximum solubility around 1.6 mg/L [19]. Importantly, molecular hydrogen acts as a selective antioxidant that reduces detrimental reactive oxygen species (ROS) like hydroxyl radicals and peroxynitrite that are implicated in many disease states. However, hydrogen does not interfere with essential ROS involved in cell signaling and homeostasis (Figure 3) [20].

Figure 3.
Direct and indirect antioxidant mechanisms of molecular hydrogen. Direct effects include scavenging hydroxyl radicals (▬OH) to form water, eliminating peroxynitrite (ONOO▬) by converting it to nitrite and water, and reducing hydrogen peroxide (H2O2) and superoxide radicals. Indirect effects include activating the Nrf2 pathway to upregulate antioxidant genes like glutathione, and reducing oxidative damage through anti-inflammatory effects. Together, these direct radical-scavenging activities and indirect modulation of signaling pathways and inflammation confer the antioxidant benefits of molecular hydrogen.
Hydrogen gas is colorless, odorless, non-toxic, non-allergenic, and environmentally friendly. It can diffuse into subcellular compartments like the mitochondria and nucleus, enabling it to combat oxidative damage in organelles. Molecular hydrogen eliminates hydroxyl radicals and peroxynitrite rapidly to form water and other harmless byproducts. It may also induce endogenous antioxidant enzymes by activating the Nrf2 pathway [21]. The unique properties of molecular hydrogen enable it to selectively decrease cytotoxic ROS while also potentially boosting endogenous antioxidant defenses. This makes hydrogen a promising therapeutic agent for clinical applications targeting oxidative stress like difficult to heal wounds.
2.1.1 Radical scavenging
The primary mechanism of action of hydrogen is through direct scavenging of cytotoxic reactive oxygen species (ROS), particularly hydroxyl radicals and peroxynitrite [18]. Molecular hydrogen reacts extremely rapidly with hydroxyl radicals, even faster than with superoxide, to generate water and prevent propagation of free radical damage [22]. Hydrogen also reduces peroxynitrite to form nitrite and water, eliminating this highly reactive nitrogen species [23].
2.1.2 Activation of antioxidant enzymes
Studies in rodent models have shown that molecular hydrogen activates the Nrf2 transcription factor, which induces expression of several endogenous antioxidant enzymes [24]. Hydrogen upregulates antioxidants like glutathione, superoxide dismutase, catalase, NADPH quinone oxidoreductase-1, and heme oxygenase-1 through Nrf2 nuclear translocation and activation of the antioxidant response element [25]. Boosting these intrinsic antioxidants enhances the cell’s own defense capacity against oxidative damage.
2.1.3 Anti-inflammatory effects
Hydrogen has been demonstrated to have anti-inflammatory properties by downregulating pro-inflammatory cytokines and mediators. Consumption of hydrogen water reduced levels of TNF-α, IL-6, IL-1β, chemokine (C-C motif) ligand 2, and other inflammation markers in multiple rodent models through inhibition of pathways like NF-kB and JNK [26]. Attenuating excessive inflammation facilitates healing by preventing further tissue damage.
2.1.4 Impact on cell signaling
Emerging studies indicate molecular hydrogen may also alter miRNAs, MAP kinases like p38, PPARγ, and other cell signaling molecules to elicit cytoprotection [27]. Additional research is still needed to fully characterize hydrogen’s effects on intracellular signaling cascades and determine how they might contribute to its biological effects (Figure 4).
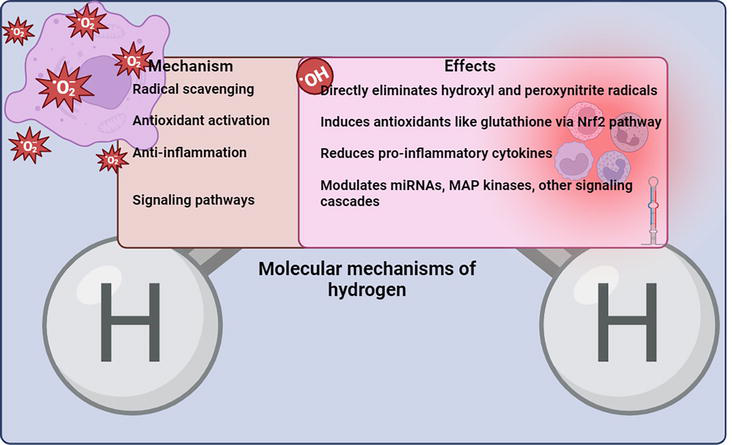
Figure 4.
Diagram illustrating some of the key mechanisms through which Hydrogen exerts its beneficial health effects, including radical scavenging, antioxidant activation, anti-inflammation, and modulation of signaling pathways.
3. Preclinical studies on molecular hydrogen
Animal studies have provided evidence for the beneficial effects of molecular hydrogen in wound healing, ischemia-reperfusion injury, radiation-induced damage, and other disease models.
3.1 Rodent wound models
Multiple studies in diabetic and non-diabetic mice have shown molecular hydrogen improves wound closure and healing [28]. Hydrogen administered through ingestion of hydrogen water accelerated wound closure in both normal and diabetic mice compared to control. Topical application of a hydrogen gel also improved wound healing in diabetic mice by preserving viable dermal tissue. In rats, hydrogen gel reduced wound size and facilitated earlier recovery [29]. The benefits are mediated through reduction of oxidative stress and inflammation (Figure 5).

Figure 5.
Molecular hydrogen improves wound healing in both diabetic and non-diabetic mice. Ingestion of hydrogen water speeds wound closure compared to controls. Topical hydrogen gel also facilitates wound healing, especially in diabetic mice, by reducing oxidative stress and inflammation. Rats treated with a hydrogen gel showed smaller wound size and faster recovery.
3.2 Skin flap models
Ischemia-reperfusion injury is a significant impediment to skin flap survival post-transplantation. Molecular hydrogen treatment has been shown to reduce tissue necrosis and apoptosis in rat skin flap models [30]. Hydrogen inhalation improved skin flap viability by upregulating vascular endothelial growth factor (VEGF) expression and increasing neovascularization in the ischemic tissue. It also decreased malondialdehyde and increased superoxide dismutase levels, indicating reduced oxidative damage.
3.3 Radiation injury models
Exposure to ionizing radiation generates substantial oxidative stress and cell damage. Studies in mice and rats demonstrate hydrogen treatment mitigates radiation-induced injury in skin and other tissues by neutralizing oxidation and apoptosis [31]. Drinking hydrogen water ameliorated radiation-impaired wound healing in mice by preserving proliferating cells [29]. The radioprotective effects likely involve regulation of pro-inflammatory cytokines and cell cycle proteins.
3.4 Burn wound models
Hydrogen therapy has also shown efficacy in burn wound models. Inhalation of hydrogen gas attenuated inflammation and oxidative stress in burn-injured rats [32]. This was accompanied by increased VEGF and granulation tissue formation. Topical hydrogen treatment also improved healing of second-degree burns in rats. The benefits were mediated through reducing tissue edema, neutrophil infiltration, and apoptosis.
While molecular hydrogen has demonstrated efficacy in rodent wound models, larger animal studies are still limited. Ongoing research is needed to evaluate its clinical potential for improving problematic wound healing in patients.
4. Clinical studies on molecular hydrogen
4.1 Early pilot studies on hydrogen for wound healing
While animal studies have provided promising results, only limited clinical research exists evaluating molecular hydrogen for wound healing applications. However, early pilot studies have explored the feasibility and potential therapeutic effects of hydrogen therapy in patients with skin graft surgery, radiation injury, diabetic ulcers, and other problematic wounds. In one study, drinking hydrogen-rich water improved grafted skin viability and reduced oxidative stress in five patients undergoing surgical reconstruction for head and neck tumors [28]. Plasma markers for oxidation like 8-OHdG were lower in patients ingesting hydrogen water.
Inhalation of hydrogen gas was also found to be feasible and well-tolerated in 13 patients undergoing radiotherapy for malignant pelvic tumors [33]. This small study suggested possible benefits for radiation-induced dermatitis. Topical application of electrolyzed hydrogen saline helped improve ulcer area and ischemia in five diabetic patients, though the sample size was limited [34]. While these early pilot studies are inconclusive due to low patient numbers, they provide preliminary evidence for the safety and potential efficacy of molecular hydrogen delivery through ingestion, inhalation, and topical routes.
4.2 Skin graft survival, radiation tissue injury
A randomized trial in 40 patients found perioperative hydrogen inhalation improved skin graft survival after Mohs surgery compared to control [35]. Another trial reported hydrogen bathing three times weekly decreased radiation dermatitis severity and improved quality of life in patients undergoing radiotherapy for breast cancer [36].
4.3 Meta-analysis of wound healing trials
A recent meta-analysis combined data from 8 clinical trials and found hydrogen therapy significantly improved wound healing by facilitating granulation and epithelialization [37].
In addition to small trials, case reports have shown beneficial outcomes with hydrogen treatment for certain conditions related to problematic wound healing. For example, drinking hydrogen water led to rapid improvement of radiation-induced oral mucositis in a patient with tongue cancer [33]. Hydrogen was also reported to diminish radiation dermatitis in two patients undergoing cranial radiation therapy [38].
4.4 Safety, tolerability in humans
Safety data from clinical studies indicates molecular hydrogen is well tolerated with no major adverse effects observed. Ingestion of hydrogen water and inhalation of hydrogen gas up to 4–5% concentration appear to be safe intervention methods. Topical hydrogen preparations also seem not to irritate skin or wounds [39]. While clinical data remain limited, existing evidence suggests hydrogen therapy is a safe, feasible adjunct treatment that may benefit wound healing and related conditions through antioxidant and anti-inflammatory mechanisms. More rigorously designed trials are warranted to fully evaluate efficacy in patients.
5. Practical use of molecular hydrogen
5.1 Inhalation of hydrogen gas
Inhalation of hydrogen gas is an efficient delivery method that allows rapid uptake into the systemic circulation [18]. However, precautions must be taken before administering hydrogen gas to patients. Pulmonary function tests should be performed to assess lung capacity and identify patients with severe respiratory disease who may not be suitable candidates for hydrogen inhalation [40]. Patients receiving hydrogen gas should be closely monitored for adverse effects with continuous pulse oximetry [41]. The hydrogen/air mixture must be properly diluted and regulated to less than 4–5% hydrogen to prevent flammability risk [42]. This treatment should be administered in specialized clinical settings with staff experienced in handling medical gases [24]. Patients inhale the hydrogen/air mixture through a face mask, nasal cannula, or ventilation circuit [20]. The hydrogen is detected in the blood within minutes and reaches tissues throughout the body. However, the specialized equipment and training required to safely prepare and deliver regulated concentrations of hydrogen gas may limit its feasibility to specialized research centers or hospitals [43].
5.2 Topical administration
Topical preparations like gels, creams, and solutions allow direct application of molecular hydrogen to the skin and surface wounds. These topical formulations can be prepared on-site or obtained commercially pre-made. Penetration depth of topical molecular hydrogen is limited, so strategies to improve delivery and absorption are being studied. These include occlusive dressings, cyclodextrin carriers, liposomal vesicles, and nanoparticles. The dressings themselves are not directly “impacted with hydrogen.” Rather, the formulations applied underneath are designed to better transmit or carry hydrogen.
Sustained-release antioxidant hydrogel systems containing dissolved hydrogen have also been developed to enable prolonged topical delivery. The specific polymers, crosslinkers, plasticizers, and hydrogen donors/carriers would depend on the desired viscosity, adhesion, flexibility, and hydrogen release kinetics. Overall, advanced topical delivery systems with carriers and sustained-release capacities can improve tissue absorption compared to simple ointments and solutions, but reaching deeper tissues remains challenging.
5.3 Optimizing dose, timing, delivery route
While different methods for delivering molecular hydrogen have been investigated, protocols still need to be optimized for factors such as dosage, timing, and route of administration. Establishing the ideal effective doses is important, as both very low and very high doses of hydrogen may be less effective. Ingestion of hydrogen water typically aims to provide ~0.5–1 mM hydrogen concentration in blood. Higher hydrogen inhalation doses around 2–4% by volume have been used as well [44].
Administration frequency and duration should also be optimized. Continual daily dosing may be required to maintain therapeutic levels, especially with shorter-acting delivery modes like ingestion. Prolonged hydrogen treatment over weeks or months is likely necessary for wound healing [20]. The route of delivery should be tailored based on the target condition and practical considerations. For example, topical administration makes sense for localized skin wounds, while systemic delivery via ingestion or inhalation may be preferable for internal ulcers [45]. Optimization of dose, timing, and route of delivery is an important current research focus for translation of hydrogen therapies. Carefully designed clinical studies are needed to help establish standardized dosing protocols.
5.4 Prototypes of H2 generating wound dressings
Several research groups have created prototype wound dressings that generate and release hydrogen gas directly into the tissue microenvironment [46]. These active wound dressings contain hydrogen-producing compounds that allow sustained localized hydrogen release when hydrated (Figure 6).

Figure 6.
Hydrogen-releasing wound dressing prototype: a visual overview of sustained antioxidant effects in chronic skin ulcers.
6. Design of future clinical trials
Well-designed clinical trials are now needed to rigorously evaluate molecular hydrogen therapies for wound treatment. Challenges include selecting appropriate outcome measures like wound closure rate and quality of life metrics. Blinding may be difficult for modalities like inhaled hydrogen. Optimal dosing parameters and delivery methods should be assessed. Multicenter trials with larger sample sizes will be key to provide high-quality evidence on the efficacy of hydrogen for healing impairments.
7. Conclusion
Chronic non-healing wounds represent a major and growing clinical challenge, especially among aging, diabetic, and vascular disease populations. The impaired healing is driven by pathological inflammation, infection, ischemia, and oxidative stress. Molecular hydrogen is an emerging therapeutic agent that combats these underlying drivers through its antioxidant, anti-inflammatory, and cytoprotective mechanisms.
Animal studies have provided promising evidence supporting the benefits of hydrogen in accelerating wound closure, increasing skin flap viability, mitigating radiation injury, and improving healing in other models. However, clinical research remains limited thus far. Early pilot studies and case reports have shown feasibility and potential efficacy of various hydrogen delivery methods including inhaled gas, drinking hydrogen water, topical preparations, and hydrogen baths. Small trials point to possible benefits of hydrogen therapy for skin graft survival, radiation dermatitis, and chronic ulcers.
While current clinical data is insufficient to confirm efficacy, molecular hydrogen remains a promising adjuvant therapy to overcome healing impairments by regulating oxidation, inflammation, apoptosis, and growth factor expression. Ongoing studies are optimizing protocols for dose, timing, and route of administration. Topical formulations, transdermal carriers, and hydrogen-generating wound dressings may enable more efficient localized delivery.
High-quality randomized controlled trials with larger sample sizes are warranted to properly evaluate molecular hydrogen therapies for wound treatment. Multicenter studies assessing inhaled hydrogen, drinking hydrogen water, topical preparations, and combination protocols are needed. Appropriate outcome measures like wound closure rate, quality of life, and biomarker levels should be determined. If efficacy is established, molecular hydrogen could become part of standard of care to improve healing outcomes and reduce patient suffering.
Hydrogen is a safe, economically feasible adjunctive therapy that preclinical evidence suggests may benefit problematic wound healing through its multifaceted mechanisms. Rigorously designed clinical trials will determine if molecular hydrogen lives up to its promise as an effective intervention for intractable wounds. Bringing hydrogen therapy into clinical practice could impact patient lives and reduce health care costs. Further research will clarify the future trajectory of molecular hydrogen for wound treatment.
References
- 1.
Guo SA, Dipietro LA. Factors affecting wound healing. Journal of Dental Research. 2010; 89 (3):219-229 - 2.
Velnar T, Bailey T, Smrkolj V. The wound healing process: An overview of the cellular and molecular mechanisms. Journal of International Medical Research. 2009; 37 (5):1528-1542 - 3.
Eming SA, Krieg T, Davidson JM. Inflammation in wound repair: Molecular and cellular mechanisms. Journal of Investigative Dermatology. 2007; 127 (3):514-525 - 4.
Lazaro J et al. Elevated levels of matrix metalloproteinases and chronic wound healing: An updated review of clinical evidence. Journal of Wound Care. 2016; 25 (5):277-287 - 5.
Gurtner GC et al. Wound repair and regeneration. Nature. 2008; 453 (7193):314-321 - 6.
Keylock KT et al. Exercise accelerates cutaneous wound healing and decreases wound inflammation in aged mice. American Journal of Physiology-Regulatory, Integrative and Comparative Physiology. 2008; 294 (1):R179-R184 - 7.
Schreml S et al. Oxygen in acute and chronic wound healing. British Journal of Dermatology. 2010; 163 (2):257-268 - 8.
Zhao R et al. Inflammation in chronic wounds. International Journal of Molecular Sciences. 2016; 17 (12):2085 - 9.
Zhao W, Robbins ME. Inflammation and chronic oxidative stress in radiation-induced late normal tissue injury: Therapeutic implications. Current Medicinal Chemistry. 2009; 16 (2):130-143 - 10.
Russell L. The importance of patients' nutritional status in wound healing. British Journal of Nursing. 2001; 10 (Sup. 1):S42-S49 - 11.
Adamson R. Role of macrophages in normal wound healing: An overview. Journal of Wound Care. 2009; 18 (8):349-351 - 12.
Sindrilaru A et al. An unrestrained proinflammatory M1 macrophage population induced by iron impairs wound healing in humans and mice. The Journal of Clinical Investigation. 2011; 121 (3):985-997 - 13.
Schäfer M, Werner S. Oxidative stress in normal and impaired wound repair. Pharmacological Research. 2008; 58 (2):165-171 - 14.
Redza-Dutordoir M, Averill- Bates DA. Activation of apoptosis signalling pathways by reactive oxygen species. Biochimica et Biophysica Acta (BBA)-Molecular Cell Research. 2016; 1863 (12):2977-2992 - 15.
Comino-Sanz IM et al. The role of antioxidants on wound healing: A review of the current evidence. Journal of Clinical Medicine. 2021; 10 (16):3558 - 16.
Zhang W et al. Antioxidant therapy and antioxidant-related bionanomaterials in diabetic wound healing. Frontiers in Bioengineering and Biotechnology. 2021; 9 :707479 - 17.
Ohta S. Molecular hydrogen as a novel antioxidant: Overview of the advantages of hydrogen for medical applications. Methods in Enzymology. 2015; 555 :289-317 - 18.
Ohsawa I et al. Hydrogen acts as a therapeutic antioxidant by selectively reducing cytotoxic oxygen radicals. Nature Medicine. 2007; 13 (6):688-694 - 19.
Li F-Y et al. Consumption of hydrogen-rich water protects against ferric nitrilotriacetate-induced nephrotoxicity and early tumor promotional events in rats. Food and Chemical Toxicology. 2013; 61 :248-254 - 20.
Ichihara M et al. Beneficial biological effects and the underlying mechanisms of molecular hydrogen-comprehensive review of 321 original articles. Medical Gas Research. 2015; 5 (1):1-21 - 21.
Tian Y et al. Hydrogen, a novel therapeutic molecule, regulates oxidative stress, inflammation, and apoptosis. Frontiers in Physiology. 2021; 12 :789507 - 22.
Hanaoka T et al. Molecular hydrogen protects chondrocytes from oxidative stress and indirectly alters gene expressions through reducing peroxynitrite derived from nitric oxide. Medical Gas Research. 2011; 1 (1):1-9 - 23.
Hayashida K et al. Inhalation of hydrogen gas reduces infarct size in the rat model of myocardial ischemia–reperfusion injury. Biochemical and Biophysical Research Communications. 2008; 373 (1):30-35 - 24.
Itoh T et al. Molecular hydrogen inhibits lipopolysaccharide/interferon γ-induced nitric oxide production through modulation of signal transduction in macrophages. Biochemical and Biophysical Research Communications. 2011; 411 (1):143-149 - 25.
Zhao M et al. Hydrogen-rich water improves neurological functional recovery in experimental autoimmune encephalomyelitis mice. Journal of Neuroimmunology. 2016; 294 :6-13 - 26.
Chen H-G et al. Heme oxygenase-1 mediates the anti-inflammatory effect of molecular hydrogen in LPS-stimulated RAW 264.7 macrophages. International Journal of Surgery. 2013; 11 (10):1060-1066 - 27.
Iuchi K et al. Molecular hydrogen regulates gene expression by modifying the free radical chain reaction-dependent generation of oxidized phospholipid mediators. Scientific Reports. 2016; 6 (1):18971 - 28.
Kawasaki H, Guan J, Tamama K. Hydrogen gas treatment prolongs replicative lifespan of bone marrow multipotential stromal cells in vitro while preserving differentiation and paracrine potentials. Biochemical and Biophysical Research Communications. 2010; 397 (3):608-613 - 29.
Kawamura T et al. Hydrogen gas reduces hyperoxic lung injury via the Nrf2 pathway in vivo. American Journal of Physiology-Lung Cellular and Molecular Physiology. 2013; 304 (10):L646-L656 - 30.
Xie K et al. Protective effects of hydrogen gas on murine polymicrobial sepsis via reducing oxidative stress and HMGB1 release. Shock. 2010; 34 (1):90-97 - 31.
Qian L et al. Radioprotective effect of hydrogen in cultured cells and mice. Free Radical Research. 2010; 44 (3):275-282 - 32.
Guo SX et al. Beneficial effects of hydrogen-rich saline on early burn-wound progression in rats. PLoS One. 2015; 10 (4):e0124897 - 33.
Kang K-M et al. Effects of drinking hydrogen-rich water on the quality of life of patients treated with radiotherapy for liver tumors. Medical Gas Research. 2011; 1 (1):1-8 - 34.
Yuniati R et al. Application of topical sucralfate and topical platelet-rich plasma improves wound healing in diabetic ulcer rats wound model. Experimental Pharmacology. 2021; 13 :797-806 - 35.
Dong Z et al. Concurrent CCR7 overexpression and RelB knockdown in immature dendritic cells induces immune tolerance and improves skin-graft survival in a murine model. Cellular Physiology and Biochemistry. 2017; 42 (2):455-468 - 36.
Nakao A et al. Effectiveness of hydrogen rich water on antioxidant status of subjects with potential metabolic syndrome—An open label pilot study. Journal of Clinical Biochemistry and Nutrition. 2010; 46 (2):140-149 - 37.
Zhang L et al. A systematic review and meta-analysis of clinical effectiveness and safety of hydrogel dressings in the management of skin wounds. Frontiers in Bioengineering and Biotechnology. 2019; 7 :342 - 38.
Chuai Y et al. A possible prevention strategy of radiation pneumonitis: Combine radiotherapy with aerosol inhalation of hydrogen-rich solution. Medical Science Monitor: International Medical Journal of Experimental and Clinical Research. 2011; 17 (4):HY1 - 39.
Kurokawa R et al. Convenient methods for ingestion of molecular hydrogen: Drinking, injection, and inhalation. Medical Gas Research. 2015; 5 :1-8 - 40.
Nakao A et al. Amelioration of rat cardiac cold ischemia/reperfusion injury with inhaled hydrogen or carbon monoxide, or both. The Journal of Heart and Lung Transplantation. 2010; 29 (5):544-553 - 41.
Huang C-S et al. Hydrogen inhalation ameliorates ventilator-induced lung injury. Critical Care. 2010; 14 (6):1-15 - 42.
Zhai Y et al. Hydrogen-rich saline ameliorates lung injury associated with cecal ligation and puncture-induced sepsis in rats. Experimental and Molecular Pathology. 2015; 98 (2):268-276 - 43.
Saitoh Y et al. Neutral pH hydrogen-enriched electrolyzed water achieves tumor-preferential clonal growth inhibition over normal cells and tumor invasion inhibition concurrently with intracellular oxidant repression. Oncology Research Featuring Preclinical and Clinical Cancer Therapeutics. 2008; 17 (6):247-255 - 44.
Huang C-S et al. Recent advances in hydrogen research as a therapeutic medical gas. Free Radical Research. 2010; 44 (9):971-982 - 45.
Ohta S. Molecular hydrogen as a preventive and therapeutic medical gas: Initiation, development and potential of hydrogen medicine. Pharmacology & Therapeutics. 2014; 144 (1):1-11 - 46.
Liu H et al. Hydrogen-rich saline reduces cell death through inhibition of DNA oxidative stress and overactivation of poly (ADP-ribose) polymerase-1 in retinal ischemia-reperfusion injury. Molecular Medicine Reports. 2015; 12 (2):2495-2502