Characteristics of the osmotherapy. Abbreviations: Sodium chloride (NaCl).
Abstract
Severe traumatic brain injury is a cause of disability with economic and social repercussions. Prehospital care is a fundamental part with important attention to avoid hypoxemia, hyperventilation, and hypotension with airway protection. During the primary evaluation, a clinical examination is performed focused on classifying according to their severity, neurological involvement, and extracranial lesions and providing a prognosis. Invasive or non-invasive intracranial pressure monitoring aims to direct management to maintain adequate cerebral perfusion pressure, which should be individualized in each patient, as this depends on the status of self-regulation.
Keywords
- traumatic brain injury
- cerebral perfusion pressure
- intracranial pressure
- intracranial hypertension
- trauma
1. Introduction
Traumatic brain injury (TBI) is defined as an injury to brain caused by an external force on the head that can be a blow, explosion, or penetrating injury [1]. It causes severe disability among young people with economic and social repercussions. Existing limitations to treatment in underdeveloped countries include inadequate prehospital care, limited advanced neuromonitoring techniques, or availability of intensive care [2, 3].
Globally, it is estimated that in 2016, there were 55 million patients diagnosed with TBI, the annual cost of the likely economic losses of productivity was $1.2 billion [4]. Approximately, 69 million people each year are affected by this clinical entity, it should be noted that in this study, it showed a threefold higher incidence in developing countries [5].
In the United States, around 1.7 million people are diagnosed with TBI per year, with the most affected population being between 15 and 19 years of age, as well as those over 65 years of age, with a 2:1 ratio with a predominance of males [6]. The mortality associated with a closed traumatic injury grows exponentially when it is associated with a brain injury, which is why prehospital rapid response emergency teams play an important role [7].
Prehospital care is a fundamental part of reducing complications in patients with TBI, there are three important pillars in prehospital care, which are avoiding hypoxemia (maintaining a saturation greater than 94%), protecting the airway by evaluating the need for endotracheal intubation, and avoiding hyperventilation and hypotension [7, 8].
TBIs accounted for approximately 2.5 million emergency department visits in the United States, of which 87% (2,213,826) were treated and discharged from the emergency room; The other 11% (283,630) were admitted to hospitalization and were discharged in the following days, and approximately 2% (52,844) died in the hospital [9]. On the other hand, this pathology has significant effects on the quality of life of those people who suffer from this disease, it is estimated that in the United States, there are 3.2 to 5.3 million people living with a disability related to TBI [10].
There is evidence that patients with moderate to severe TBI who were discharged from rehabilitation were more than twice as likely to die after 3 to 5 years compared to people in the general population who were the same age, sex, and race [11].
2. Brain physiology
2.1 Brain metabolism
The human brain weighs approximately 1350 grams, which represents 2% of the total weight of an adult [12]. It takes up more energy than any other organ. Glucose is the main source of brain energy; It does not freely diffuse the blood-brain barrier. It enters the brain by active transport via glucose transporter (GLUT) 1, it is distributed to the central nervous system by several transporters (GLUT 1, GLUT 3, GLUT 5). These transporters undergo regulation with a tendency to rise under certain conditions, such as hypoxia [13].
The generation of brain energy under conditions of oxygen and glucose at normal levels is carried out by means of glycolysis by oxidative phosphorylation of creatine phosphokinase and adenylyl cyclase. The process allows the formation of 3 molecules of adenosine triphosphate (ATP) for each nicotinamide adenine dinucleotide (NADH) and a maximum of 38 molecules of ATP is obtained for each molecule of glucose. In the absence of oxygen in astrocytes, anaerobic glucose metabolism is performed to form lactate and ATP is generated to capture glutamate. Lactate is taken up by neurons and transformed into pyruvate that initiates the citric acid cycle, in this process, hydrogen ions are released which are associated with acidosis and more brain damage. In this way, ATP generation is lower per glucose molecule, generating only 2 ATP molecules per glucose molecule, which is not enough for brain demands [13].
On the other hand, during a prolonged fast, the brain uses ketone bodies which are broken down into acetyl coenzyme A to be oxidized in the citric acid cycle, so in extreme conditions, the brain can produce low amounts of energy from gluconeogenesis using glycerol, glutamine, and glycine as substrates. With low oxygen levels, ATP generation then drops and phosphocreatine deposits are occupied until anaerobic metabolism is exhausted, resulting in the cessation of electrical activity in the brain. Maintaining normal blood glucose levels is essential for normal brain function. The brain is particularly sensitive to hypoglycemia because there are no glycogen stores [14].
2.2 Cerebral circulation
The cerebral irrigation is given by the internal carotid arteries, which branch and give rise to the anterior circulation, the vertebral arteries give rise to the basilar artery and its branches give rise to the posterior circulation [15].
The brain occupies approximately 15% of cardiac output (750 ml/min) and maintains a cerebral blood flow of 50 ml/100 g/minute. In the resting state, the brain consumes oxygen at an approximate rate of 3.5 ml/100 g/minute, which represents 20% of the body’s total consumption [16].
Cerebral perfusion pressure (CPP) is defined as the difference between mean arterial pressure (MAP) and intracranial pressure (ICP). Under normal conditions in adults, it ranges between 60 and 150 mmHg and the ICP around 10 mmHg [16].
2.3 Monro-Kellie theory
The skull, after the closure of the sutures and fontanelles, becomes an inextensible configuration and, therefore, maintains a constant volume regardless of the contents. Under normal conditions, this content can be separated into three components (Monro-Kellie’s theory): brain 80%, cerebrospinal fluid (CSF) 10%, and blood 10%, respectively. When there is an increase in the volume of any of the three components, the pressure exerted by that component on the other two also increases. The CSF is the main compensatory system [15].
2.4 Partial pressure of carbon dioxide (PaCO2) and its implication in cerebral blood flow
The acid-base state and its regulation have been shown to have a direct effect on cerebral blood flow (CBF), causing a series of changes that have implications for health, for years, it has been an important area of study in which CBF, PaCO2, bicarbonate (HCO-3), and pH are integrated.
The cerebral circulation varies with the levels of PaCO2, in normal situations, the CBF increases from 2 to 4% of mmHg with an increase of PaCO2, making P toCO2 the most potent physiological vasodilator [17].
The response seen in CBF to changes from PaCO2 occurs in seconds and completes equilibrium within 1 to 2 minutes due to rapid diffusion across the blood-brain barrier (BBB) to perivascular fluid and cerebral vascular smooth muscle cells.
Changes in CBF secondary to PaCO2 levels are not sustained for prolonged periods. The adaptation point in the pH of CBF is observed at 6 hours with subsequent decrease in secondary changes to PaCO2 [12, 18].
3. Clinical classifications for TBI
The primary evaluation of a patient with TBI consists of a clinical examination focused on classifying according to its severity, neurological, and even structural involvement, as well as providing prognosis.
The Glasgow Coma Scale (GCS) (score from 3 to 15 pts) and the FOUR scale (score from 0 to 16 pts) are among the most commonly used scales to assess and identify the patient’s state of consciousness and guide management [19, 20].
It is recommended to establish a single scale for the progressive evaluation of the patient at 6, 12, 24, and 72 hours of the patient and to avoid marked differences in the patient’s approach and prognosis [21, 22, 23].
3.1 Tomographic classification
Computed tomography (CT) classifications of TBI lesions are used to establish prognosis, estimate risks of intracranial hypertension (IH), and thus establish probable surgical treatment. The most commonly used classifications for these types of scenarios are Marshall and Rotterdam. Other classifications, such as Helsinki and Stockholm, have demonstrated good sensitivity and specificity in some studies for predicting decline, but it still does not exceed the previous ones mentioned in use [24].
3.1.1 Marshall’s tomographic classification
Standard method for the evaluation of CT in traumatic brain injury involves evaluating the mesencephalic cisterns, midline, presence or absence of hemorrhage and whether or not they were evacuated, as well as cerebral edema, generating a percentage risk of IH and mortality or poor clinical outcomes. Mortality and the risk of IH increase with each grade of the scale, up to 55 and 63% respectively in grade III and 100% in grade IV. In addition to these degrees, two more are described that refer to whether these hemorrhagic masses were evacuated or not; in the case of the evacuated mass, the mortality has been described between 33 and 52% and in the non-evacuated, the mortality reaches up to 80% [25, 26].
3.1.2 Rotterdam tomographic scale
A scale that values epidural hematoma and subarachnoid hemorrhage, demonstrating better performance than the Marshall classification for the 6-month prognosis, with similar results in terms of mortality. But the fact of individualizing the lesions gives you a better picture of exploration and quantification of risk. In scores 1 and 2, the mortality is 0%, in score 3, the mortality is up to 6%, in score 4, the mortality is 35%, in score 5, the mortality percentage is 54%, and in score 6, the mortality reaches 60% [25, 26, 27].
4. Neuromonitoring in the emergency room
Neuromonitoring encompasses direct and indirect techniques that help to provide early evidence of neuraxial damage and focus on reducing secondary injury by optimizing the balance between oxygen consumption and demand [28, 29]. Invasive and non-invasive surrogate PCI monitoring techniques and measurements will be addressed as a treatment guide in the emergency department.
4.1 Neurological monitoring strategies in the emergency room
4.2 Non-invasive intracranial pressure monitoring
4.2.1 Transcranial Doppler (TCD)
In the initial evaluation of patients with severe TBI, TCD measurements may help guide treatment and decrease mortality [34].
TCD is a non-invasive ultrasound that uses a low-frequency transducer (<2 MHz) that detects the intracranial circulation through an acoustic bony window, is based on emissions and flow receptions, the angle and position of the insonation must be adjusted to provide the best Doppler signal [35, 36].
The acoustic windows used are transorbital, temporal, submandibular, and foramen magnum. In clinical practice, the middle cerebral artery (MCA) is the most insonable due to its easy access through the temporal window above the zygomatic arch, it has a sensitivity of 38–91%, specificity of 94–100% prediction of vasospasm [37]. The TCD measures flow rates, spectral wave analysis, and various indices that vary according to the insonation window and the vessel analyzed.
The pulsatibility index (PI) is calculated as:
The normal value of PI is between 0.6 and 1.16, a PI less than 0.6 is associated with vasospasm, hyperemia, or high-grade stenosis; a PI between 1.2 and 1.6 is associated with moderate IH or microangiopathy, a PI greater than 1.7–3 with severe IH, and values greater than 3 are associated with severe IH and cerebral asystole [37]. The PCI can be estimated based on the PI with the following equation [38]:
The Resistance Index (RI) which is calculated as:
It increases in the early stages of IH and in old age [16]. Anything per <0.75 is considered normal [39].
Lindegaard Index (LI)
It is a value that normalizes the flow velocity of the AMC with respect to internal carotid arteries (ICA), it serves to differentiate hyperemia from true vasospasm. A normal LI is considered <3, mild vasospasm 3.0–4.5, moderate 4.5–6.0, and severe >6.0 [39]. It is calculated as:
4.2.2 Optic nerve sheath diameter (ONSD)
The optic nerve is an extension of the central nervous system, lined by meninges and cerebrospinal fluid (Figure 1). The optic nerve sheath (ONS) is in continuity with the meninges, specifically the dura mater. It is the intraorbital segment of the optic nerve and is separated from it by cerebrospinal fluid and the arachnoid trabeculae [1, 4]. The elevation of the PCI causes the CSF to be distributed through the dura mater toward the ONS, increasing its diameter [40].
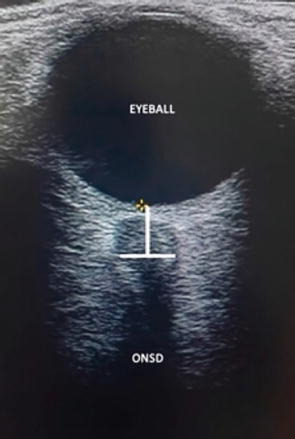
Figure 1.
ONSD measurement. Optic nerve sheath diameter (ONSD).
Several studies have validated the linear relationship between the measurement of ONS by ultrasound with invasive methods and PCI, with detection of ICP elevation in ranges of 4.8–5.6 mm with an overall sensitivity and specificity of 95 and 92%, respectively [41].
Serial measurement of the ONSD and the use of the following validated formula for inference of PCI with the ONSD [42] are recommended:
This method should not be used in patients with facial trauma, Graves’ disease, and sarcoidosis [31].
ONSD measurement is a validated non-invasive method that is easy and quick to use. Performing it together with DCT in patients with severe TBI upon admission to the emergency room can be taken as a guide for initiation of treatment and neuroprotective measures [43].
4.2.3 Other methods
Near-infrared spectroscopy (NIRS) has an uncertain sensitivity for recognizing increased PCI, yet it is considered a complementary non-invasive method in neurological monitoring, limited by its non-specificity and restricted availability [44]. Another method, such as quantitative optical pupillometry, may be useful to guide response to osmotic treatment or as a monitoring of PCI, but its use in emergency departments has not been reported [45].
4.2.4 Continuous electroencephalogram (cEEG)
cEEG provides neuronal information in response to changes in brain structure or function. It is a continuous registry, fast and easy to use, however, with a high cost. It is used for the detection of non-convulsive status epilepticus and cerebral ischemia (CBF < 25–35 mL/100 g/min, alpha and beta waves are attenuated, and if CBF <17–18 mL/100 g/min, slow delta and zeta waves are increased) [46].
4.3 Invasive intracranial pressure monitoring in the emergency room
4.3.1 Jugular bulb saturation (SjvO2)
It is a minimally invasive method of neuromonitoring. Currently, an ultrasound-guided fiber optic catheter is placed, both sides can be catheterized, however, right placement is more common [47].
SjvO2 measures the relationship between brain metabolic requirements and blood flow, being a direct determination of brain oxygen consumption (CMRO2) [48, 49].
It has a normal value of 55 to 75%, a low SjvO2 is associated with vasospasm, ischemia, or metabolic cause (fever, chills, agitation). Elevated SjvO2 is associated with hyperemia, hyperoxia, arteriovenous shunt, or extensive cerebral infarction. Although it is part of the monitoring in TBI guidelines, it is rarely used in the emergency department [49].
4.3.2 External ventricular drainage
External ventricular drainage (EVD) is globally considered the gold standard for PCI monitoring. It is used as continuous monitoring of PCI and treatment of IH for various pathologies (acute hydrocephalus, hematomas, hemorrhages, severe TBI, tumor, infection, or cerebral edema) [50]. It is associated with complications such as obstruction, improper placement, infections, and bleeding [51].
The components of the ICP wave are:
P1 is the arterial component (percussive wave). It originates from the pulsation of the choroid plexus, it has constant amplitude.
P2 represents intracranial compliance (tidal wave).
P3 is associated with the closure of the aortic valve (dicrotic wave).
P2 and P3 are produced from the retrograde venous beat of the jugular veins over cortical veins, between which the dicrote cleft of the pulse occurs. The change in the P2 wave is indicative of IH and consequently loss of brain self-regulation (Figure 2) [52].
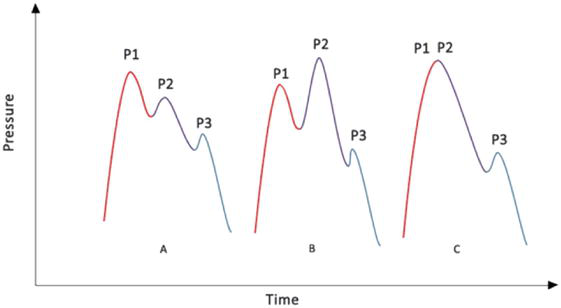
Figure 2.
Morphology of ICP waves. (A) Normal ICP curve. (B) Decreased cerebral compliance with increased P2. (C) Decreased compliance with critical increase in ICP. Intracranial pressure (ICP).
5. Treatment of severe TBI
The presence of other extracranial lesions should be considered in the management of patients with severe TBI. Post-traumatic bleeding is one of the most common causes of death in patients with TBI associated with other injuries, and is a preventable cause of death [53, 54]. Therefore, priority should be given to controlling bleeding in case of hemodynamic instability, considering medical and surgical management [54].
It is recommended that during bleeding control, a hemoglobin (Hb) greater than 7 g/dL and, in some cases, higher levels be maintained, for example, in patients with low myocardial reserve or previous heart disease and older adults. If surgical management is warranted, a platelet count greater than 50,000/mm3 and an INR of less than 1.5 should be maintained. A partial pressure oxygen (PaO2) level of 60 to 100 mmHg and a PaCO2 of 35 to 40 mmHg are maintained to ensure adequate oxygen transport [54, 55].
In hemodynamic control, a MAP greater than 80 mmHg or a systolic blood pressure (SBP) greater than 100 mmHg is sought, in case these pressure levels make it difficult to control bleeding, lower levels could be considered for short periods [55]. In addition, during the management of bleeding, consider massive transfusion protocols, as well as the use of thromboelastography (TEG) and rotational thromboelastometry (ROTEM) to guide the decision to use blood products [56].
After hemorrhage control, the severity of the brain injury should be assessed by means of pupillary evaluation, the level of consciousness with the GCS, and the performance of an imaging study [57, 58].
When performing the tomographic study, the presence of potentially surgical life-threatening intracranial lesions should be sought, and in such a case, an emergency evaluation by the neurosurgeon should be requested, who will regulate surgical management [59].
Within the management of PCI, the factors that are related to worse outcomes are values greater than 20 to 25 mmHg and the duration of the outcomes [58, 60]. The goal of management is to maintain an adequate PPC, which must be individualized in each patient, since this depends on the status of self-regulation [61], therapy should not only focus on maintaining a specific value in all patients. It is suggested in most guidelines and studies that maintaining a PPC value between 60 and 70 mmHg has better outcomes and fewer neurological sequelae [62]. PPC values above 100 mmHg are related to vasogenic edema and values below 50 mmHg are related to cerebral ischemia [61, 63].
Among the measures that can reduce PCI in order to improve PPC are osmotherapy (20% mannitol or hypertonic saline), external interventricular drainage, and hyperventilation [4, 6]. Patients with IH such as respiratory depression, bradycardia, hypertension, or signs of cerebral herniation (mydriasis or anisocoria) require immediate medical treatment with one of these options. Prophylactic use in the absence of data on increased PCI with osmotherapy over conventional therapy has not shown improvement in outcomes [62].
Mannitol and hypertonic saline (Table 1) are similar in efficacy in the control of IH [64, 65]. The decision of which osmotic therapy to use should be based on consideration of its adverse effects and the clinical scenario, as in the case of mannitol that generates osmotic diuresis and hypovolemia, which would have to be made in conjunction with volume expansion to avoid undesirable hemodynamic effects. Hypertonic saline could be considered a better option in cases of hypovolemia due to the volume expansion it generates, however, both therapies warrant monitoring of serum sodium levels and water balances [65, 66].
Bolus dose | Mechanism of action | Disadvantages | |
---|---|---|---|
Mannitol 20% | 0.25 to 1 g/kg |
|
|
Hypertonic solutions: 3% NaCl 5% NaCl 7.5% NaCl 10% NaCl 14.6% NaCl 23.4% NaCl | 250 mL or 2.5–5 mL/kg 100 mL 2–4 mL/kg or 100 mL 75 mL 24–48 mL 30 mL (0.686–2.0 mL/kg) |
|
|
Table 1.
The use of hyperventilation (PaCO2 25 to 30 mmHg) is effective for the management of IH, it is considered a transient therapy in which a definitive surgical management is carried out and in cases of imminent herniation. Prolonged use of hyperventilation has deleterious effects, as well as the risk of cerebral ischemia, due to its effects on cerebral vasculature [66, 67].
The use of antiepileptic drugs prophylactically for post-traumatic seizures is not routinely recommended [68]; their use is considered only in patients with risk factors, such as skull fracture, acute subdural hematoma, initial loss of consciousness, amnesia for more than 24 hours, and age greater than 65 years [68, 69].
Other therapies for the management of TBI can be mentioned, however, there is no evidence of benefit, such as the use of high doses of glucocorticoids or the use of albumin during fluid resuscitation; In both cases, an increase in mortality was observed when compared to conventional therapy [70, 71].
Within ventilatory management, the indication for orointubation and use of mechanical ventilation depends on a combination of factors ranging from the level of consciousness (GCS less than 8 pts), loss of airway protection reflexes, severe agitation, combative state, and significant elevation of PCI [72]. Regarding the scheduling of mechanical ventilation in patients with or without IH, the parameters may be similar to those of patients without TBI, maintaining a protective ventilation strategy (tidal volume 6 to 8 mL per predicted weight, PEEP 5 cmH2O, plateau pressure less than 30 cmH2O, and a respiratory rate of 16 to 22 breaths/minute) [73].
Ventilatory management can be staggered in the case of acute respiratory failure syndrome (ARDS), with an initial protective schedule with subsequent adjustments to avoid severe hypoxemia; The tidal volume will be adjusted to maintain a conduction pressure of less than 14 cmH2O, as well as the respiratory rate for controlled hypocapnia (PaCO2 of 32 to 35 mmHG) [74].
In case of worsening of ARDS, advanced strategies such as prone position and neuromuscular blockade should be considered in conjunction with osmotherapy with optimal MAP to ensure adequate PPC [74, 75].
Every patient with severe TBI should be given measures to maintain adequate homeostasis (keep the brain happy) [62]. Multiple mnemonics have been described to ensure measures that improve and ensure cerebral oxygenation in patients with TBI.
The mnemonics of THE MANTLE (Table 2) are used at the bedside of patients with severe TBI [76]. In the following paragraphs, we will describe what is suggested to evaluate in this mnemonic.
THE MANTLE mnemonics | ||
Temperature | 36–37.5°C | Hemoglobin |
7–9 gr/dl | Electrolytes your acid-basic status | Na + 140–150 mEq/L pH: 7.35–7.45 p50 = 26–28 mmHg |
Metabolism | Sedation and analgesia SvjO2 > 55% | Arterial blood pressure |
SABP >100–110 mmHg | Nutrition and glucose | Glycemia 110–180 mg/dL |
Target of oxygen | PaO2 80–120 mmHg SaO2 > 95% | Lung protective ventilation |
Vt 6–10 ml/kg PBW RR to achieve PaCO2 35–45 mmHg PP < 14 DP < 13 cm H2O | Edema and ICP control | ICP < 22 mmHg CPP 55–70 mmHg ONSD <5.8 mm, PI <1.2 Serial CT scan |
Table 2.
The MANTLE mnemonics.
Abbreviations: Cerebral perfusion pressure (CPP); systolic arterial blood pressure (SABP); tidal volume (Vt); respiratory rate (RR); Plateau pressure (PP); driving pressure (DP); intracranial pressure (ICP); predicted body weight (PBW); oxygen pressure at half arterial oxygen pressure (p50); optic nerve sheath diameter (ONSD); pulsatility index (PI); Computed Tomography (CT).
It is considered essential to avoid hyperthermia in these patients, this generates an increase in brain metabolism, and therefore risk of cerebral hypoxia; A temperature target of 36 to 37°C is sought [76, 77].
Maintaining adequate oxygen transport is important. A Hb between 7 and 9 g/dL is suggested for adequate oxygen transport and to avoid hypoxia [76, 78].
Serum electrolyte values and acid-base status are important for brain homeostasis; serum sodium levels of 135 to 145 mEq/L and avoiding hypotonic solutions are important to maintain an adequate osmolar gradient; in the base acid, a pH of 7.35 to 7.45 is suggested to avoid modification of the Hb dissociation curve and alteration of oxygen delivery to tissues and organs [76].
Adequate sedation, pain control, agitation, and treatment of seizures are part of bedside assessment to prevent an increase in basal metabolic rate [76, 79].
As already mentioned, the avoidance of hypotension is of utmost importance, since it is related to worse outcomes, a BPS of 100 to 110 mmHg, maintaining a normal volume, a normal peripheral perfusion, a diuresis of at least 30 ml/hr., and a central venous pressure of 6 to 10 cmH2O are suggested [76, 80].
Glucose control is crucial for proper brain metabolism. A range of 110 to 180 mg/dL is suggested, since values outside this range are related to metabolic crises and generation of neurotoxic cascades that alter homeostasis [76, 81].
In terms of oxygenation, we have a PaO2 target of 80 to 110 mmHg and a SaO2 greater than 94%, avoiding hyperoxia as well as hypoxia [76]. In case of mechanical ventilation, pulmonary protection parameters should be maintained, as mentioned above [75, 76].
Finally, cerebral edema and PCI control should be given. In case of neurological monitoring, maintain a PCI of less than 22 mmHg, SjvO2 between 50 and 75%, and non-invasively, an ONSD <5.8 mm, a PI <1.2, and a brain computed tomography scan without signs of cerebral edema [76].
Within the management of patients with severe TBI, stepwise management is sought [82]. We recommend the algorithm in Figure 3, which is based on the recommendations previously discussed, always remembering the individualization of the patient.
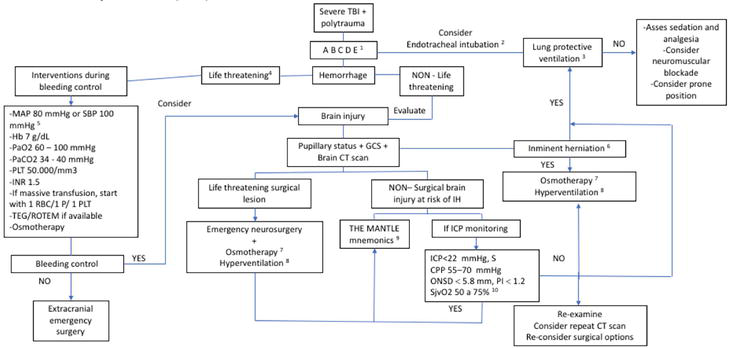
Figure 3.
Consensus algorithm. (1) primary assessment, (2) patient with impaired state of consciousness (GCS <8), (3) VT 6 to 8 ml, PEEP 5 cmH2O, PPLAT 30 cmH2O, RR of 16 to 22 breaths/minute, (4) bleeding with hemodynamic instability data, (5) in case of difficulty in controlling bleeding, consider lower values, (6) mydriasis or anisocoria, (7) mannitol or hypertonic saline, (8) maintain a PaCO2 of 25 to 30 mmHg, (9) follow mnemonics to maintain a happy brain, (10) consider maintaining adequate pain control, sedation, temperature, and agitation. Abbreviations: Tidal volume (VT), positive end-expiratory pressure (PEEP), plateau pressure (PPLAT), respiratory rate (RR), computed tomography (CT), Glasgow coma scale (GCS), mean arterial pressure (MAP), systolic blood pressure (SBP), hemoglobin (Hb), arterial partial pressure of oxygen (PaO2), arterial partial pressure of carbon dioxide (PaCO2), red blood cell (RBC), plasma (P), platelet (PLT), international normalized ratio (INR), thromboelastography (TEG), rotational thromboelastometry (ROTEM), intracranial pressure (ICP), cerebral perfusion pressure (CPP), intracranial hypertension (IH), optic nerve sheath diameter (ONSD), pulsatility index (PI), jugular bulb saturation (SjvO2).
6. Conclusion
The identification and appropriate approach of a patient with severe TBI, whether prehospital or Intra-hospital, requires a broad knowledge of brain physiology, in order to direct an appropriate neurological monitoring, whether invasive or non-invasive, and thus obtain information that allows us to direct a treatment in a staggered manner or controlling the ICP levels to guarantee the best CPP, taking into account variations in brain self-regulation. Regardless of the form in which the treatment is directed, the cornerstone is individualization.
References
- 1.
Hiskens M, Mengistus T, et al. Systematic review of the diagnostic and clinical utility of salivary micro RNAs in traumatic brain injury (TBI). International Journal of Molecular Sciences. 2022; 23 :13160. DOI: 10.3390/ijms232113160 - 2.
Rubiano AM, Griswold DP, Jibaja M, Rabinstein AA, Agustin GA. Management of severe traumatic brain injury in regions with limited resources. Brain Injury. 2021; 35 (11):1317-1325. DOI: 10.1080/02699052.2021.1972149 - 3.
Stein KY, Froese L, Gomez A, et al. Intracranial pressure monitoring and treatment thresholds in acute neural injury: A narrative review of the historical achievements, current state, and future perspectives. Neurotrauma Reports. 2023; 4 (1):478-494. DOI: 10.1089/neur.2023.0031 - 4.
Rolle ML, Garba DL, et al. The impact of traumatic brain injury on economic productivity in the Caribbean. Brain and Spine. 2023; 3 :101755. ISSN 2772-5294 - 5.
Joannides A, Korhonen TK, Clark D, et al. An international, prospective observational study on traumatic brain injury epidemiology study protocol: GEO-TBI: Incidence. NIHR Open Research. 2023; 3 :34. DOI: 10.3310/nihropenres.13377.1 - 6.
Georges A, Das M, J. Traumatic brain injury. In: StatPearls [Internet]. Treasure Island (FL): StatPearls Publishing; 2023 - 7.
Lulla A, Lumba-Brown A, et al. Prehospital guidelines for the management of traumatic brain injury – 3rd edition. Prehospital Emergency Care. 2023; 27 (5):507-538. DOI: 10.1080/10903127.2023.2187905 - 8.
Sun, Hai S, Navdeep S, et al. Impact of prehospital transportation on survival in skiers and snowboarders with traumatic brain injury. World Neurology. 2017; 104 :909-918.e8. DOI: 10.1016/j.wneu.2017.05.108 - 9.
Capizzi AN, Woo J, Verduzco-Gutierrez M. Traumatic brain injury. Medical Clinics of North America. 2020; 104 (2):213-238. DOI: 10.1016/j.mcna.2019.11.001 - 10.
Selassie AW et al. Incidence of long-term disability following traumatic brain injury hospitalization, United States. Journal of Head Trauma Rehabilitation. 2003; 23 (2):123-131. DOI: 10.1097/01.HTR.0000314531.30401.39 - 11.
Harrison-Felix C et al. Mortality after surviving traumatic brain injury risks based on age groups. Journal of Head Trauma Rehabilitation. 2012; 27 (6):E45-E56. DOI: 10.1097/HTR.0b013e31827340ba - 12.
Vavilala MS, Lee LA, Lam AM. Cerebral blood flow and vascular physiology. Anesthesiology Clinics of North America. 2002; 20 (2):247-264. DOI: 10.1016/s0889-8537(01)00012-8 - 13.
Golding EM, Robertson CS, Bryan RM. The consequences of traumatic brain injury on cerebral blood flow and autoregulation: A review. Clinical and Experimental Hypertension. 1999; 21 (4):299-332. DOI: 10.3109/10641969909068668 - 14.
Taylor C, Hirsch N. Applied cerebral physiology. Anaesthesia & Intensive Care Medicine. 2007; 8 (10):407-412. DOI: 10.1016/j.mpaic.2007.08.010 - 15.
Stratmann G. Neurotoxicity of anesthetic drugs in the developing brain. Anesthesia & Analgesia. 2011; 113 (5):1170-1179. DOI: 10.1213/ane.0b013e318232066c - 16.
Avitsian R, Schubert A. Anesthetic considerations for intraoperative management of cerebrovascular disease in neuro-vascular surgical procedures. Anesthesiology Clinics. 2007; 25 :441-463. DOI: 10.1016/j.anclin.2007.06.002 - 17.
Caldwell HG, Carr JMJR, Minhas JS, Swenson ER, Ainslie PN. Acid-base balance and cerebrovascular regulation. The Journal of Physiology. 2021; 599 (24):5337-5359. DOI: 10.1113/jp281517 - 18.
Robinson C. Moderate and severe traumatic brain injury. Continuum: Lifelong Learning in Neurology. 2021; 27 (5):1278-1300. DOI: 10.1212/con.0000000000001036 - 19.
Mehta R, Trainee G, Chinthapalli K, Neurologist C. Glasgow coma scale explained. BMJ. 2019; 365 :l1296. DOI: 10.1136/bmj.l1296 - 20.
Barea-Mendoza JA, Llompart-Pou JA, Pérez-Bárcena J, Quintana-Díaz M, Serviá-Goixart L, Guerrero-López F, et al. External validation of the glasgow coma scale with pupillary assessment in patients with severe traumatic brain injury. Emergencies. 2023; 31 (1):34-45 - 21.
McNett M, Amato S, Philippbar SA. A comparative study of Glasgow coma scale and full outline of unresponsiveness scores for predicting long-term outcome after brain injury. Journal of Neuroscience Nursing. 2016; 48 (4):207-214. DOI: 10.1097/jnn.0000000000000225 - 22.
McNett M, Amato S, Gianakis A, Grimm D, Philippbar SA, Belle J, et al. The four score and GCS as predictors of outcome after traumatic brain injury. Neurocritical Care. 2014; 21 (1):52-57. DOI: 10.1007/s12028-013-9947-6 - 23.
Ghelichkhani P, Esmaeili M, Hosseini M, Seylani K. Glasgow coma scale and FOUR score in predicting the mortality of trauma patients; a diagnostic accuracy study. Emergency. 2018; 6 (1):1-7 - 24.
Thelin EP, Nelson DW, Vehviläinen J, Nyström H, Kivisaari R, Siironen J, et al. Evaluation of novel computerized tomography scoring systems in human traumatic brain injury: An observational, multicenter study. PLoS Medicine. 2017; 14 (8):e1002368. DOI: 10.1371/journal.pmed.1002368 - 25.
Ricardez-Cazares LG, González-Fernández MA, Dehesa-López E, Peraza-Garay F. Rotterdam vs. Marshall; comparison of outcome predictors in traumatic brain injury using computed tomography. Revmeduas. 2021; 11 (2):87-94. DOI: 10.28960/revmeduas.2007-8013.v11.n2.002 - 26.
Asim M, El-Menyar A, Parchani A, Nabir S, Ahmed MN, Ahmed Z, et al. Rotterdam and Marshall scores for prediction of in-hospital mortality in patients with traumatic brain injury: An observational study. Brain Injury. 2021; 35 (7):803-811. DOI: 10.1080/02699052.2021.1927181 - 27.
Bm RRP, Siironen J, Skrifvars MB, Hernesniemi J, Kivisaari R. Predicting the outcome in traumatic brain injury development of a new computed tomography classification system (Helsinki CT score). Neurosurgery. 2014; 75 (6):632-647 - 28.
Llompart-Pou JA, Barea-Mendoza JA, Sánchez-Casado M, González-Robledo J, Mayor-García DM, et al. Neuromonitoring in severe traumatic brain injury. Data from the Spanish ICU trauma registry (RETRAUCI). Neurosurgery. 2020; 31 (1):1-6. DOI: 10.1016/j.neucir.2019.05.005 - 29.
Chesnut RM, Temkin N, Carney N, et al. Global neurotrauma research group. A trial of intracranial-pressure monitoring in traumatic brain injury. The New England Journal of Medicine. 2012; 367 :2471e81. DOI: 10.1056/NEJMoa1207363 - 30.
Teasdale G, Maas A, Lecky F, Manley G, Stocchetti N, Murray G. The glasgow coma scale at 40 years: Standing the test of time. The Lancet Neurology. 2014; 13 (8):844-854. DOI: 10.1016/S1474-4422(14)70120-6 - 31.
Nyam TE, Ao KH, Hung SY, et al. Four score predicts early outcome in patients after traumatic brain injury. Neurocritical Care. 2017; 26 :225-231. DOI: 10.1007/s12028-016-0326-y - 32.
Godoy DA, Rabinstein AA. How to manage traumatic brain injury without invasive monitoring? Current Opinion in Critical Care. 2022; 28 (2):111-122. DOI: 10.1097/MCC.0000000000000914 - 33.
Bathala L, Mehndiratta MM, Sharma VK. Transcranial doppler: Technique and common findings (part 1). Annals of Indian Academy of Neurology. 2013; 16 (2):174-179. DOI: 10.4103/0972-2327.112460 - 34.
Bouzat P, Oddo M, Payen J-F. Transcranial Doppler after traumatic brain injury. Current Opinion in Critical Care. 2014; 20 (2):153-160. DOI: 10.1097/mcc.0000000000000071 - 35.
Rasulo FA, Bertuetti R. Transcranial Doppler and optic nerve sonography. Journal of Cardiothoracic and Vascular Anesthesia. 2019; 33 :S38-S52. DOI: 10.1053/j.jvca.2019.03.040 - 36.
Ract C, Le Moigno S, Bruder N, Vigué B. Transcranial Doppler ultrasound goal-directed therapy for the early management of severe traumatic brain injury. Intensive Care Medicine. 2007; 33 (4):645-651. DOI: 10.1007/s00134-007-0558-6 - 37.
Kostin A. Transcranial Doppler in neurological intensive care. Revista Mexicana de Anestesiologia. 2015; 38 (Suppl. 3):427-429 - 38.
Hansen HC, Helmke K. Validation of the optic nerve sheath response to changing cerebrospinal fluid pressure: Ultrasound findings during intrathecal infusion tests. Journal of Neurosurgery. 1997; 87 :34-40. DOI: 10.3171/jns.1997.87.1.0034 - 39.
Ohle R, McIsaac S, Woo M, Perry J. Sonography of the optic nerve sheath diameter for detection of raised intracranial pressure compared to computed tomography. Journal of Ultrasound in Medicine. 2015; 34 (7):1285-1294. DOI: 10.7863/ultra.34.7.1285 - 40.
Nag DS, Sahu S, Swain A, Kant S. Intracranial pressure monitoring: Gold standard and recent innovations. World Journal of Clinical Cases. 2019; 7 (13):1535-1553. DOI: 10.12998/wjcc.v7.i13.1535 - 41.
Ramírez-García S, Carranza -Castro PH, Gutiérrez-Salinas J, García-Ortiz L, Hernández-Rodríguez S. Application of near-infrared spectroscopy in medicine. Medicina Interna de México. 2012; 28 (4):365-370 - 42.
Ghosh A, Elwell C, Smith M. Review article: Cerebral near-infrared spectroscopy in adults: A work in progress. Anesthesia and Analgesia. 2012; 115 :1373-1383. DOI: 10.1213/ANE.0b013e31826dd6a6 - 43.
Robba C, Santori G, Czosnyka M, Corradi F, Bragazzi N, Padayachy L, et al. Optic nerve sheath diameter measured sonographically as non-invasive estimator of intracranial pressure: A systematic review and meta-analysis. Intensive Care Medicine. 2018; 44 (8):1284-1294. DOI: 10.1007/s00134-018-5305-7 - 44.
Blandino OA, Higuera JL. Usefulness of quantitative pupillometry in the intensive care unit. Medicina Intensiva. 2022; 46 (5):273-276. DOI: 10.1016/j.medine.2021.12.011 - 45.
Rajagopalan S, Sarwal A. Neuromonitoring in critically ill patients. Critical Care Medicine. 2023; 51 (4):525-542. DOI: 10.1097/CCM.0000000000005809 - 46.
Gibbs EL, Lennox W, Nims LS, Gibbs FA. Arterial and ce-rebral venous blood: Arterial-venous differences in man. The Journal of Biological Chemistry. 1942; 144 :325-332 - 47.
Chieregato A. Normal jugular bulb oxygen saturation. Journal of Neurology, Neurosurgery, and Psychiatry. 1 Jun 2003; 74 (6):784-786. DO: 10.1136/jnnp.74.6.784(74)49(5):583-6 - 48.
Aten Q , Killeffer J, Seaver C, Reier L. Causes, complications, and costs associated with external ventricular drainage catheter obstruction. World Neurosurgery. 2020; 134 :501-506. DOI: 10.1016/j.wneu.2019.10.105 - 49.
Nawabi NL, Stopa BM, Lassarén P, Bain PA, Mekary RA, et al. External ventricular drains and risk of freehand placement: A systematic review and meta-analysis. Clinical Neurology and Neurosurgery. 2023; 231 :107852. DOI: 10.1016/j.wneu.2019.10.105 - 50.
Rodríguez-Boto G, Rivero-Garvía M, Gutiérrez-González R, Márquez-Rivas J. Basic concepts about brain pathophysiology and intracranial pressure monitoring. Neurología. 2015; 30 (1):16-22. DOI: 10.1016/j.nrl.2012.09.002 - 51.
Teixeira PG, Inaba K, Hadjizacharia P, Brown C, Salim A, Rhee P, et al. Preventable or potentially preventable mortality at a mature trauma center. The Journal of Trauma. 2007; 63 (6):133846. DOI: 10.1097/TA.0b013e31815078ae - 52.
Callcut RA, Kornblith LZ, Conroy AS, Robles AJ, Meizoso JP, Namias N, et al. The why and how our trauma patients die: A prospective Multicenter Western trauma association study. Journal of Trauma and Acute Care Surgery. 2019; 86 (5):864-870. DOI: 10.1097/TA.0000000000002205 - 53.
Picetti et al. WSES consensus conference guidelines: Monitoring and management of severe adult traumatic brain injury patients with polytrauma in the first 24 hours. World Journal of Emergency Surgery. 2019; 14 :53. DOI: 10.1186/s13017-019-0270-1 - 54.
Spahn DR, Bouillon B, Cerny V, Duranteau J, Filipescu D, Hunt BJ, et al. The European guideline on management of major bleeding and coagulopathy following trauma. Critical Care. 2017; 23 (1):98. DOI: 10.1186/s13054-019-2347-3 - 55.
Carney N, Totten AM, O'Reilly C, Ullman JS, Hawryluk GW, Bell MJ, et al. Guidelines for the management of severe traumatic brain injury. Neurosurgery. 2017; 80 (1):6-15. DOI: 10.1227/NEU.0000000000001432 - 56.
Kornblith LZ, Moore HB, Cohen MJ. Trauma-induced coagulopathy: The past, present, and future. Journal of Thrombosis and Haemostasis. 2019; 17 (6):852-862. DOI: 10.1111/jth.14450 - 57.
Subcommittee A. Advanced trauma life support (ATLS®). Journal of Trauma and Acute Care Surgery. 2013; 74 (5):1363-1366. DOI: 10.1097/ta.0b013e31828b82f5 - 58.
Badri S, Chen J, Barber J, Temkin NR, Dikmen SS, Chesnut RM, et al. Mortality and long term functional outcome associated with intracranial pressure after traumatic brain injury. Intensive Care Medicine. 2012; 38 (11):1800-1809. DOI: 10.1007/s00134-012-2655-4 - 59.
Bullock MR, Chesnut R, Ghajar J, Gordon D, Hartl R, Newell DW, et al. Guidelines for the surgical management of traumatic brain injury. Neurosurgery. 2006; 58 (3 Suppl.):S1-S62. DOI: 10.1227/01.NEU.0000210365.36914.E3 - 60.
Vik A, Nag T, Fredriksli OA, Skandsen T, Moen KG, Schirmer-Mikalsen K, et al. Relationship of “dose” of intracranial hypertension to outcome in severe traumatic brain injury. Journal of Neurosurgery. 2008; 109 (4):678-684. DOI: 10.3171/JNS/2008/109/10/0678 - 61.
Balestreri M, Czosnyka M, Hutchinson P, Steiner LA, Hiler M, Smielewski P, et al. Impact of intracranial pressure and cerebral perfusion pressure on severe disability and mortality after head injury. Neurocritical Care. 2006; 4 (1):8-13. DOI: 10.1385/NCC:4:1:008 - 62.
Bulger EM, May S, Brasel KJ, Schreiber M, Kerby JD, Tisherman SA, et al. Outof-hospital hypertonic resuscitation following severe traumatic brain injury: A randomized controlled trial. Journal of the American Medical Association. 2010; 304 (13):1455-1464. DOI: 10.1001/jama.2010.1405 - 63.
Geeraerts T, Velly L, Abdennour L, Asehnoune K, Audibert G, et al. Management of severe traumatic brain injury (first 24 hours). Anaesthesia, Critical Care & Pain Medicine. 2018; 37 :171-186. DOI: 10.1016/j.accpm.2017.12.001 - 64.
Kamel H, Navi BB, Nakagawa K, Hemphill JC 3rd, Ko NU. Hypertonic saline versus mannitol for the treatment of elevated intracranial pressure: A metaanalysis of randomized clinical trials. Critical Care Medicine. 2011; 39 (3):554-559. DOI: 10.1097/CCM.0b013e318206b9be - 65.
Rickard AC, Smith JE, Newell P, Bailey A, Kehoe A, Mann C. Salt or sugar for your injured brain? A meta-analysis of randomised controlled trials of mannitol versus hypertonic sodium solutions to manage raised intracranial pressure in traumatic brain injury. Emergency Medicine Journal. 2014; 31 (8):679-683. DOI: 10.1136/emermed-2013-202679 - 66.
Fortune JB, Feustel PJ, Graca L, Hasselbarth J, Kuehler DH. Effect of hyperventilation, mannitol, and ventriculostomy drainage on cerebral blood flow after head injury. The Journal of Trauma. 1995; 39 (6):1091-1097. DOI: 10.1097/00005373-199512000-00014 - 67.
Coles JP, Minhas PS, Fryer TD, Smielewski P, Aigbirihio F, Donovan T, et al. Effect of hyperventilation on cerebral blood flow in traumatic head injury: Clinical relevance and monitoring correlates. Critical Care Medicine. 2002; 30 (9):1950-1959. DOI: 10.1097/00003246-200209000-00002 - 68.
Ramakrishnan V, Dahlin R, Hariri O, Quadri SA, Farr S, Miulli D, et al. Antiepileptic prophylaxis in traumatic brain injury: A retrospective analysis of patients undergoing craniotomy versus decompressive craniectomy. Surgical Neurology International. 2015; 6 :8. DOI: 10.4103/2152-7806.149613 - 69.
Bhullar IS, Johnson D, Paul JP, Kerwin AJ, Tepas JJ 3rd, Frykberg ER. More harm than good: Antiseizure prophylaxis after traumatic brain injury does not decrease seizure rates but may inhibit functional recovery. Journal of Trauma and Acute Care Surgery. 2014; 76 (1):54-60. DOI: 10.1097/TA.0b013e3182aafd15 - 70.
Roberts I, Yates D, Sandercock P, Farrell B, Wasserberg J, Lomas G, et al. Effect of intravenous corticosteroids on death within 14 days in 10,008 adults with clinically signifificant head injury (MRC CRASH trial): Randomised placebo-controlled trial. Lancet. 2004; 364 (9442):1321-1328. DOI: 10.1016/S0140-6736(04)17188-2 - 71.
Reinhart K, Perner A, Sprung CL, Jaeschke R, Schortgen F, Johan Groeneveld AB, et al. Consensus statement of the ESICM task force on colloid volume therapy in critically ill patients. Intensive Care Medicine. 2012; 38 (3):368-383. DOI: 10.1007/s00134-012-2472-9 - 72.
Stevens RD, Lazaridis C, Chalela JA. The role of mechanical ventilation in acute brain injury. Neurologic Clinics. 2008; 26 :543-563. DOI: 10.1016/j.ncl.2008.03.014 - 73.
Della Torre V, Badenes R, Corradi F, Racca F, Lavinio A, Matta B, et al. Acute respiratory distress syndrome in traumatic brain injury: How do we manage it? Journal of Thoracic Disease. 2017; 9 (12):5368-5381. DOI: 10.21037/jtd.2017.11.03 - 74.
Robba C, Camporota L, Citerio G. Acute respiratory distress syndrome complicating traumatic brain injury. Can opposite strategies converge? Intensive Care Medicine. 2023; 49 :583-586. DOI: 10.1007/s00134-023-07043-6 - 75.
Robba C, Poole D, McNett M, Asehnoune K, Bösel J, et al. Mechanical ventilation in patients with acute brain injury: Recommendations of the European Society of Intensive Care Medicine consensus. Intensive Care Medicine. 2020; 46 :2397-2410. DOI: 10.1007/s00134-020-06283-0 - 76.
Godoy DA, MurilloCabezas F, Suarez JI, Badenes R, Pelosi P, Robba C. “The Mantle” bundle for minimizing cerebral hypoxia in severe traumatic brain injury. Critical Care. 2023; 27 :13. DOI: 10.1186/s13054-022-04242-3 - 77.
Badjatia N. Hyperthermia and fever control in brain injury. Critical Care Medicine. 2009; 37 (Suppl):S250-S257. DOI: 10.1097/CCM.0b013e3181aa5e8d - 78.
Lelubre C, Bouzat P, et al. Anemia management after acute brain injury. Critical Care. 2016; 20 :152. DOI: 10.1186/s13054-016-1321-6 - 79.
Taran S, Pelosi P, Robba C. Optimizing oxygen delivery to the injured brain. Current Opinion in Critical Care. 2022; 28 :145-156. DOI: 10.1097/MCC.0000000000000913 - 80.
Godoy DA, Lubillo S, Rabinstein AA. Pathophysiology and manage ment of intracranial hypertension and tissular brain hypoxia after severe traumatic brain injury: An integrative approach. Neurosurgery Clinics of North America. 2018; 29 :195-212. DOI: 10.1016/j.nec.2017.12.001 - 81.
Godoy DA, Behrouz R, Di Napoli M. Glucose control in acute brain injury: Does it matter? Current Opinion in Critical Care. 2016; 22 :120-127. DOI: 10.1097/MCC.0000000000000292 - 82.
Stocchetti N, Maas AI. Traumatic intracranial hypertension. The New England Journal of Medicine. 2014; 370 (22):2121-2130. DOI: 10.1056/nejmra1208708