Currently used therapeutic agents, their molecular action, and adverse effects.
Abstract
Natural flavonoid quercetin is widely distributed in fruits and vegetables. Quercetin may be therapeutically useful in the treatment and prevention of a variety of diseases, including cancer, diabetes, and cardiovascular disease, according to mounting research. The aim of the chapter is to provide information on the antidiabetic properties of quercetin. According to studies, quercetin regulates whole-body glucose homeostasis through interacting with a variety of molecular targets in the liver, pancreas, skeletal muscle, adipose tissue, and small intestine. The pleiotropic mechanisms of action of quercetin include the reduction of intestinal glucose absorption, insulin secretory, and insulin-sensitizing actions, as well as enhanced glucose utilization in peripheral tissues.
Keywords
- quercetin
- flavonoids
- diabetes
- gluconeogenesis
- glucose absorption
- glucose uptake
- insulin resistance
- insulin secretion
- AMPK
1. Introduction
The prevalence of diabetes mellitus (DM), which has been steadily rising over time, is a serious issue that affects the entire world. Worldwide, especially in low- to middle-income nations, the prevalence of DM has been rising. In the previous 30 years, from 108 million in 1980 to 422 million in 2014, the number of persons with DM has nearly quadrupled, according to a global report from the World Health Organization (WHO). Over 1.5 million deaths in 2012 were specifically related to DM, and 2.2 million deaths in 2012 were caused by hyperglycemia. DM is expected to rank as the sixth leading cause of mortality worldwide by the year 2030 [1]. DM has also been causing severe organ failure over the years rapidly becoming one of the noncommunicable diseases, causing a rapid increase in mortality rates [2]. The two main types of diabetes are insulin-dependent diabetes mellitus (IDDM, type 1) and noninsulin-dependent diabetes mellitus (NIDDM, type 2), as shown in Figure 1. Diabetes mellitus type 2 are caused by a complex metabolic disorder in the endocrine system, which is characterized by hyperglycemia and obstruction in the metabolism of protein, carbohydrates, and lipid as a result of either impairment or destruction of insulin action and insulin-secreting pancreatic cells in target tissues. The insulin-secreting cells in type 11 diabetes, an autoimmune condition, were targeted and destroyed by regional inflammatory mediators [3, 4]. The most encountered form of diabetes is NIDDM as it accounts for more than 80% of the total case of DM hyperglycemia brought on by impaired insulin production or insulin resistance characterizes diabetes as an endocrine illness. Diabetes is primarily divided into type I and type II, as depicted in Figure 1. Type I diabetes, commonly known as juvenile diabetes due to its predominance in youngsters, is brought on by flaws in the pancreatic insulin secretion process. As depicted in Figure 1, type II diabetes (T2DM) is characterized by insulin resistance, in which insulin binds to its receptor in the cell membrane without activating the signaling cascade necessary for glucose metabolism. It leads to pancreatic
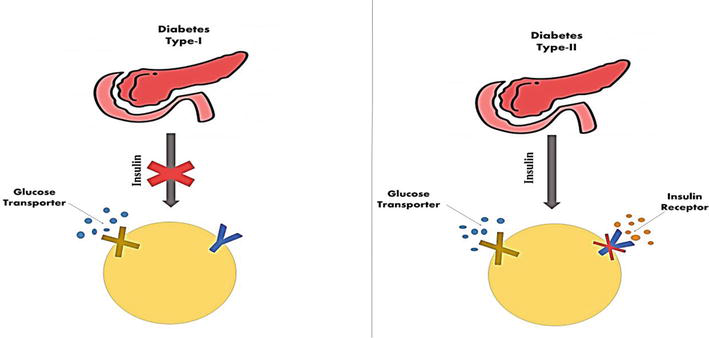
Figure 1.
Types of diabetes mellitus.
Therapeutic agent | Molecular action | Adverse effects |
---|---|---|
Metformin (Biguanides) | AMPK pathway | Felling seek, gastrointestinal disturbances, nausea, vomiting. |
Insulin | Insulin receptors in muscle, Liver, adipose tissue | Hypoglycemia, blurred vision, fast heartbeat |
Thiazolidinediones | PPAR-γ, GLUT receptors | Weight gain, edema, hepatotoxicity |
Exenatide | Stimulate insulin secretion, suppress glucagon release | Nausea, vomiting, diarrhea |
Nateglinide Repaglinide | Stimulate insulin secretion | Hypoglycemia, weight gain, ear congestion |
Glimepiride, Glipizide, Glyburide | Stimulate insulin secretion | Hypoglycemia, skin rashes, itching |
Sulphonyl ureas (Glibenclamide) | SU receptors in beta cell | Hypoglycemia, weight gain, skin reactions |
Table 1.
Flavonoid | Mechanism of Action |
---|---|
Quercetin | Reduce oxidative stress, inhibit alpha-glucosidase, stimulates glucose uptake in muscle cells |
Kaempferol | Protect beta cells and inhibit alpha amylase |
Myricetin | Promote glycogen synthesis and inhibit alpha amylase |
Apigenin | Protect beta cells |
Luteolin | Inhibit alpha amylase and glucosidase |
Genistein | Stimulate insulin secretion, and inhibit alpha amylase |
Epicatechin | Protect beta cells |
Hesperetin | Inhibit alpha glucosidase, stimulate glucose uptake |
Rutin | stimulate glucose uptake Inhibit alpha glucosidase and reduce oxidative stress |
Table 2.
Bioflavonoids and their mechanism of action.
The acceleration of population aging, as well as behavioral and socioeconomic shifts, have made metabolic illnesses a significant public health issue Therefore, the search for safe and efficient treatment medications is urgent. Physiotherapy has been valued in diverse traditional cultures, and it is believed that natural products are more economical and safer than chemical products. In the prevention or/and treatment of diseases, natural substances derived from fruits, vegetables, or medicinal plants are frequently used. Flavonoids are a superfamily of phytochemicals that have been taken from natural plants and are thought to offer therapeutic promise for metabolic disorders [10]. Quercetin is a crucial flavonoid component that is present in a variety of edible and therapeutic plants. Due to its excellent potential for the treatment of metabolic illnesses, quercetin has attracted a lot of interest recently [11]. Numerous pharmacological effects include hepatoprotective, anti-inflammatory, anticancer, hypoglycemic, hypolipidemic, and cardiovascular protection. Additionally, it has been shown in several clinical trials to be advantageous for type 2 diabetes, hyperlipidemia, and NAFLD [12].
2. Natural source of quercetin
Quercetin is widely distributed in the roots, leaf, and fruit of many medicinal plants, including
Food source | Quercetin in mg/100 g | Food source | Quercetin in mg/100 g |
---|---|---|---|
Spinach | 27.2 | Radicchio | 32.0 |
Black tea | 2.0 | Fennel leaves | 49.0 |
Red grapes | 1.38 | Kale | 23.0 |
Capers | 233 | Cherries | 2.7 |
Onion | 22.0 | Red apples | 4.7 |
Radish leaves | 70.0 | Green tea | 2.7 |
Oregano | 42.0 | Red apples | 4.7 |
Dill | 79.0 | Green tea | 2.7 |
Chili pepper | 32.6 | Cilantro | 53.0 |
Lemon | 2.29 | Coriander | 5.00 |
Tomato | 2.7 | Radish | 70.37 |
Coen poppy | 26.30 | Okra | 24.00 |
Pear | 0.59 | Plum | 2.34 |
Fig | 0.87 | Strawberry | 1.02 |
Blueberry | 9.92 | Blackberry | 2.70 |
Table 3.
Food rich in quercetin.
3. Physicochemical properties of quercetin
The melting point of quercetin is 313–314°C, and it is a bright yellow needle-like crystal. It has the chemical formula C15H10O7 with a molecular mass of 302.23. Quercetin is also known as 3,3′,4′,5,7-pentahydroxyflavone or 3,3′,4′,5,7-pentahydroxy-2-phenylchromen-4-one. The flavonol subclass of flavonoids includes quercetin, which has two aromatic rings (A and B) connected by a three-carbon pyrone ring (C). Each of the following locations has a hydroxyl group: 3, 3′, 4′, 5, and 7 as shown in Figure 2. Quercetin is soluble in ethanol, methanol, and ethyl acetate, slightly soluble in petroleum ether, benzene, ether, and chloroform, and nearly insoluble in water [14]. It is regarded as having a strong antioxidant effect since its molecular arrangement is acknowledged as the optimum one for scavenging free radicals and binding transition metal ions.
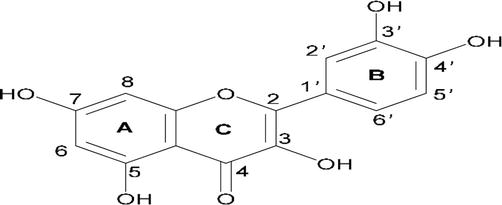
Figure 2.
Quercetin’s chemical structure, with (a, B) and (C) standing in for the benzene and pyrone rings, respectively.
4. Bioavailability of quercetin
Fruits and vegetables contain glycoconjugates of quercetin. The quantity and kind of sugar moiety affect the solubility and absorption of glycoconjugates. Quercetin’s,
5. Mechanism of action of quercetin against diabetes
5.1 Effects of quercetin on glycemia
Quercetin is a promising drug target for treating diabetes. Several mechanisms, including improved insulin sensitivity, promoted glycogen synthesis, and reduced insulin resistance, have been put up to explain quercetin antihyperglycemic effects. It enhances glucose metabolism and insulin production, which all work together to improve the insulin sensitization effect and also quercetin inhibits the enzymes glucosidase and amylase. In addition, quercetin maintained the mass and functionality of β-cells, hence enhancing the serum insulin effect, and lowered blood glucose in streptozotocin (STZ)-induced diabetes mice. Quercetin, on the other hand, improved the β-cells’ ability to secrete insulin and prevented diabetes by reducing oxidative stress in alloxan-diabetic animal models [10].
Quercetin stimulates the GLUT4 expression and endogenous GLUT4 translocation by increasing estrogen receptor, subsequently enhancing phosphorylation of both phosphatidylinositol-3-kinase/Akt (PI3K/Akt) and AMP-activated protein kinase/Akt (AMPK/Akt) signal pathways, increasing the glucose uptake in skeletal muscle cells. Que. acts similarly to rosiglitazone-like drugs such as glycogen phosphorylase (GP) and peroxisome proliferator-activated receptor γ(PPARγ) agonist on insulin-receptor signaling and glucose transport to increase the utilization of glucose [16]. Quercetin might considerably raise plasma insulin levels and lower blood glucose levels by preserving the bulk and activity of cells and enhancing serum insulin action. Additionally, the establishment of the diabetic state in alloxan-diabetic animal models is based on the death of cells brought on by oxidative stress. It has a variety of ways to harm beta cells. However, by lowering oxidative stress in diabetic patients, the quercetin can lessen islet cell failure, improve cell insulin secretion, and further prevent DM.
5.2 Mechanisms of quercetin’s hypoglycemic effects
5.2.1 Enhancement of glucose uptake in tissue
In people, quercetin can affect a variety of organs, including the liver, pancreas, small intestine, and muscles. Skeletal muscles that are responsive to insulin are where more than 80% of glucose is absorbed by humans. Therefore, a problem with skeletal muscle glucose absorption might affect the body’s overall glucose balance and eventually cause type 2 diabetes. In skeletal muscles, quercetin increases adenosine monophosphate kinase (AMPK), which, in turn, stimulates GLUT4 receptors [17]. Through GLUT4, which facilitates glucose entry into cells, glucose is processed, which controls the glucose level. Additionally, quercetin stimulates AMPK activity in hepatocytes and blocks glucose 6-phosphatase. The main signaling molecules that control cellular GLUT4 expression are AMPK and CaMKII. Exercise is also an effective GLUT4 expression activator, which enhances insulin action and muscle glycogen synthesis (Figure 3). On the other hand, quercetin administration decreases intestine sodium-dependent glucose absorption and GLUT2 expression [18]. Quercetin functions similarly to rosiglitazone as a PPAR gamma agonist in that it influences signal transduction and improves the utilization of glucose through affecting glucose transport and insulin-receptor signaling [10].
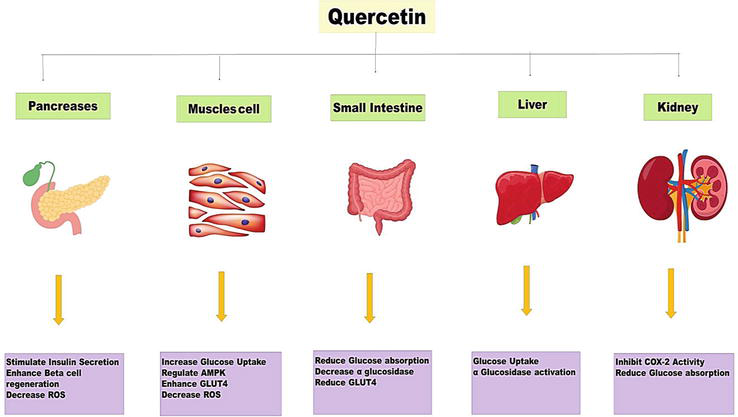
Figure 3.
Mechanism of quercetin as antidiabetic agent.
5.2.2 Release of insulin from β-cells
Pancreatic beta cells secrete insulin, which regulates blood glucose levels when blood sugar levels rise. Reduced viability and functionality of pancreatic cells are frequently linked to diabetic problems. Quercetin improves glucose metabolism and insulin secretion, has a superior hypoglycemic impact, and has an insulin-sensitizing effect by encouraging pancreatic-cell proliferation [19]. In INS-1E (insulin-secreting rat insulinoma) cells, quercetin may help to increase glucose-stimulated insulin secretion and insulin expression. According to a study, quercetin can prevent the malfunctioning of pancreatic cells brought on by cholesterol, preserving insulin production triggered by glucose and glycemic management [19]. By specifically activating extracellular signal-regulated kinase 1/2 (ERK1/2), quercetin might greatly improve the insulin secretion of INS-1 pancreatic cells. Moreover, by triggering the intracellular Ca2+ signaling system, quercetin dramatically increased the amount of insulin secreted. Additionally, during an oral glucose tolerance test in diabetic rats, quercetin considerably raised the insulin sensitivity index at 30 minutes while significantly lowering the levels of plasma cholesterol, fasting plasma insulin, and postprandial glucose [20]. Regarding this, the beneficial effects of quercetin on islet cells can be divided into three categories, namely an increase in insulin secretion, protection of -cells, and promotion of islet-cell proliferation.
5.2.3 Insulin resistance
Insulin resistance (IR) is the term used to describe the decline in insulin’s effectiveness in promoting glucose uptake and utilization, which ultimately results in hyperglycemia. High levels of fatty acids and glucose promote an excessive buildup of ROS, which can damage cells and lead to insulin resistance in peripheral metabolic tissues [10]. In other studies, it was discovered that long-term hyperglycemia decreased the binding of the insulin receptor and the substrate (IRS-1), increased the expression of inducible nitric oxide synthase while decreasing endothelial nitric oxide synthase, and ultimately caused insulin resistance in diabetic rats. Quercetin’s effects on glycemic control postulated a number of pathways to account for the advantageous effects of this polyphenol. Inducible nitric oxide synthase expression was raised as a result of the anti-oxidative protective activity on the pancreatic islets of endothelial nitric oxide synthase, which, in turn, caused insulin resistance in diabetic rats. These advantageous effects of quercetin may be attained by decreasing the buildup of cyclic adenosine phosphoric acid (cAMP) and the input of free fatty acids, activating protein kinase A (PKA), maintaining cyclic nucleotide-dependent phosphodiesterase 3B (PDE3B), and increasing two acyl glycerol (DAG) [21].
5.2.4 Alpha-glucosidase inhibition to decrease glucose absorption
One of the therapeutic methods is to suppress the carbohydrate hydrolyzing enzymes, amylases and glucosidase, in the human digestive system in order to delay the absorption of glucose [22]. Consequently, finding glucosidase inhibitors in plants has become a very important endeavor. The intestinal GLUT2 enzyme is severely inhibited by quercetin, which lowers the absorption of glucose [23]. According to numerous reports, quercetin inhibits yeast glucosidases more potently than acarbose. Inhibiting amylase and glucosidases may, therefore, be useful in lowering blood glucose levels after consuming a diet high in mixed carbohydrates. To find out the quercetin and its glycoside derivatives’ inhibitory effects on rat intestine glucosidases in an
6. Effects of quercetin on diabetic complications
6.1 Effect on diabetic liver disorders
The term “nonalcoholic fatty liver disease” (NAFLD) refers to a clinic pathological syndrome with excessive intracellular fat deposition that is linked to metabolic illnesses such as hyperlipidemia, insulin resistance, and type 2 diabetes [25]. A growing body of research indicates a clear connection between diabetes and the emergence of liver problems. Diabetes has been linked to an increase in CYP2E1 in the liver, and the suppression of the enzyme primarily protects the liver from oxidative damage. It was discovered that STZ-induced diabetes in rats resulted in hyperglycemia, body weight loss, altered hepatocyte ultrastructure, elevated protein levels, and enhanced CYP2E1 activity in the liver [26]. In STZ-induced diabetic rats, the impact of quercetin on liver apoptosis was examined. The findings demonstrated that 15 mg/kg (IP) quercetin had a strong protective effect against liver cell damage by lowering the number of apoptotic cells; hence, quercetin can be a useful drug for the treatment of diabetes in rats. On experimentally developed diabetic rats, quercetin produced hepatoprotective effects
6.2 Effect on diabetic retinopathy
The involved mechanisms in diabetes-induced diabetic retinopathy mainly include oxidative stress, inflammation, neurodegeneration, and damaged retinal vasculature. Diabetes-related alterations to the retina were significantly prevented by quercetin therapy [28]. Notably, the protective effect of quercetin was significantly reduced by concomitant HO-1 inhibition. These findings suggest that HO-1 is essential for quercetin’s ability to protect the nervous system in DR. Quercetin inhibited high glucose-induced cell proliferation by reducing vascular endothelial growth factor (VEGF) production, according to an in vitro experiment using human retinal endothelial cells. Quercetin also had a neuroprotective effect against diabetic retinopathy [29]. Inhibition of the HMGB1/TLR4/NF-B/NLRP3 inflammation/IL-1/IL-18 axis, suppression of VEGF and sICAM-1 secretion, and encouragement of BDNF secretion
6.3 Effect on diabetic nephropathy
One of the main microvascular consequences of diabetes, diabetic nephropathy (DN), is marked by high incidence, low diagnosis rate, lengthy duration, expensive treatment, and high rates of disability and fatality. End-stage renal failure may result from mesangial enlargement and thickening of basement membranes, which cause pathological alterations such as glomerulosclerosis, interstitial fibrosis, and tubular atrophy [31]. Numerous factors, including abnormalities in lipid synthesis, oxidative stress, changes in renal hemodynamics, and signaling pathways for polyol and mitogen-activated protein kinase, all have a role in the outcome and progression of DN. Through the inhibition of protein kinase C (PKC) activity, downregulation of TGF-1 expression, reduction of extracellular matrix production, and delay of renal hypertrophy, quercetin may help the kidney shape that has been affected by hyperglycemia. In addition, quercetin can slow the onset of diabetic nephropathy, prevent degenerative alterations to the kidneys, and enhance glycolipid metabolism in type 2 diabetic rats [32]. Increased insulin resistance and high blood glucose levels have been associated to the release of pro-inflammatory mediators such as IL-1, IL-6, IL-8, IL-4, TNF-, and histamine in brown adipose tissue (Figure 3). These mediators are inhibited by quercetin, which also reduces oxidative stress. Quercetin decreases blood glucose reabsorption by lowering DPP-IV and cyclooxygenase-2 (COX-2) activities in the kidneys (Figure 3). Quercetin prevented renal development in diabetic rats with kidney disease by stimulating AMPK phosphorylation and decreasing sterol regulatory element binding protein (SREBP)-1c in the kidney [33]. This decreased the tissue dyslipidemia caused by lipid deposits. Quercetin might reduce the oxidative damage brought on by diabetes in the kidney tissues. Additionally, several investigations revealed that diabetic rats with kidney dysfunction had high lipid accumulation. In experimental type 1 diabetic rats, quercetin prevented renal progression by phosphorylating the 5′ adenosine monophosphate (AMP)-activated protein kinase (AMPK) and suppressing sterol regulatory element binding protein (SREBP)-1c in the kidney [32]. This reduced the tissue dyslipidemia condition. As a result, quercetin may 1 day be used as a clinical therapy intervention to stop DM’s neurodegenerative consequences.
6.4 Effect on diabetic reproductive disorders
Male diabetic patients frequently experience clinical issues with diabetes-induced reproductive dysfunction, which negatively impacts their sexual lives and ability to conceive. Within five to ten years of the disease’s beginning, the reproductive function declined in the majority of diabetic patients. The positive effects of quercetin on diabetes-induced reproductive dysfunction processes, such as sexual dysfunction, testicular dysfunction, or infertility, have been demonstrated in animal trials, but the underlying mechanisms are still unknown [33]. In adult male STZ-induced diabetic SD rats, a recent study examined the effects of quercetin on male sexual behavior and sperm quantity. According to additional research, treating damaged rat testes with an appropriate dosage of quercetin led to a considerable rise in the testis and epididymis weights, SOD activity, and levels of sexual hormones. On the impaired reproductive system of diabetics, quercetin has protective properties [34, 35]. Part of the protective impact of quercetin on sexual behavior, testicular cell damage, and spermatogenic damage brought on by diabetes can be attributed to anti-oxidation, cell proliferation, and anti-apoptosis.
6.5 Effect on diabetic neurodegenerative and neuroprotective disease
According to prior studies, chronic hyperglycemia, oxidative stress, and cholinergic dysfunction are three simple ways to cause diabetic neurodegenerative diseases, which primarily include cognitive and memory deficits, Alzheimer’s disease (AD), stroke, and Parkinson’s disease [10]. These diseases are a result of changes in the central nervous system. Numerous research on animals has demonstrated the therapeutic effects of antidiabetics, antioxidants, and acetylcholine esterase (AChE) inhibitors on diabetic neurodegenerative disorders, particularly memory loss and cognitive dysfunction. In many
Quercetin supplementation may raise BDNF levels, which may help with cognitive function and memory, both of which can be affected by diabetes-related diseases, such diabetic neuropathy. Furthermore, a western blot study revealed that quercetin enhanced the levels of brain-derived neurotrophic factor (BDNF), nerve growth factor (NGF), postsynaptic density 93 (PSD93), and postsynaptic density 95 (PSD95) in the brain of db/db mice. The pro-inflammatory cytokines IL-1 and IL-18, as well as NLRP3, ASC, and cleaved Caspase-1, were all expressed less, while SIRT1 was expressed more in response to quercetin. It also enhanced the protein expression of SIRT1 and lowered the expression of NLRP3 inflammation-related proteins. The present findings suggest that the SIRT1/NLRP3 pathway may be an important mechanism underlying quercetin’s neuroprotective action against DE [39].
6.6 Quercetin and other diabetic complication
One of the most challenging types of pain to manage is diabetic neuropathic pain, a significant micro-vascular consequence of diabetes mellitus (DM). There are not many reports on the use of quercetin to treat diabetic neuropathic pain. The antinociceptive effects of quercetin in normal mice and animals with diabetes caused by STZ. Diabetes patients frequently experience depression, which impairs glucose control and raises the risk of diabetic complications. Clinical care for diabetics has come to be seen as increasingly dependent on the accurate diagnosis and treatment of concomitant depression [40]. Malignant diabetic cardiomyopathy has a high incidence and fatality rate. This type of cardiomyopathy causes hypertension and coronary artery disease and is linked to mitochondrion abnormalities, hyperinsulinemia, insulin resistance, and endoplasmic reticulum modifications. Chronic hyperglycemia causes oxidative stress, which, in turn, causes issues with the central nervous system and may cause neurodegenerative diseases including Parkinson’s and Alzheimer’s. Recent research has demonstrated that quercetin treatment alleviated memory impairment and decreased brain energy metabolism in rats with STZ-induced memory impairment by lowering ATP content in a dose-dependent manner [41]. The risk of macrovascular consequences, such as hypertension, cardiomyopathy, and coronary artery disorders, is increased by persistent insulin resistance and hyperglycemia. Quercetin, with or without glibenclamide, was observed to lessen cardiomyopathy-related damage in STZ-induced rats in a dose-dependent manner. Recent research has shown that quercetin enhanced cardioprotection in STZ-induced mice by boosting endothelial cell receptors and nitric oxide generation. Another investigation including type 2 diabetes women found quercetin supplements to significantly lower systolic blood pressure. Quercetin may be an effective preventative measure against cardiovascular problems in diabetics; however, more thorough investigation and research are needed [41].
7. Conclusion and future Prospect
A common natural flavonol substance is quercetin. It can be obtained from common fruits and vegetables, as well as some common medicinal plants. The pharmacological effects and molecular mechanisms of quercetin in the management of diabetes were thoroughly reviewed in this chapter. Future preclinical and clinical research on quercetin may benefit from using the material as a resource.
Quercetin has a wide range of pharmacological activities, including actions that are antihyperglycemic, according to studies. It helps reduce hyperlipidemia and hyperglycemia. Through a number of molecular mechanisms, including AMPK, which controls GLUT4 expression in adipose tissue and muscles, quercetin lowers blood glucose levels, increases glucose tolerance, and improves pancreatic beta-cell function. Quercetin has been demonstrated to enhance pancreatic-cell proliferation, insulin sensitivity, glucose metabolism, and insulin production in diabetic animal models. Quercetin has been found to be quite important in the management of diabetes because of its many advantages. Numerous investigations are being conducted to ascertain whether quercetin has the potential to become a future antidiabetic drug. These would provide a safer alternative to the present synthetic medications on the market, which have negative side effects. To learn more about the benefits of quercetin on reducing blood glucose levels and boosting insulin release in T2DM, including the molecular basis of these actions, additional research—from animal models to human trials—is necessary.
In conclusion, more research is needed to determine the precise mechanism of QE on various disorders. QE may be used to successfully prevent some of the clinical consequences of diabetes according to the findings reported in the current review. Future research will focus on QE’s involvement in the management of diabetes and its negative effects.
References
- 1.
Sok Yen F, Shu Qin C, Tan Shi Xuan S, Jia Ying P, Yi Le H, Darmarajan T, et al. Hypoglycemic effects of plant flavonoids: A review. Evidence-based Complementary and Alternative Medicine. 2021; 2021 :2057333 - 2.
Koye DN, Magliano DJ, Nelson RG, Pavkov ME. The global epidemiology of diabetes and kidney disease. Advances in Chronic Kidney Disease. 2018; 25 (2):121-132 - 3.
Samarghandian S, Azimi-Nezhad M, Samini F, Farkhondeh T. Chrysin treatment improves diabetes and its complications in liver, brain, and pancreas in streptozotocin-induced diabetic rats. Canadian Journal of Physiology and Pharmacology. 2016; 94 (4):388-393 - 4.
Kawser Hossain M, Abdal Dayem A, Han J, Kumar Saha S, Yang GM, Choi HY, et al. Recent advances in disease modeling and drug discovery for diabetes mellitus using induced pluripotent stem cells. International Journal of Molecular Sciences. 2016; 17 (2):256 - 5.
Coman C, Rugina OD, Socaciu C. Plants and natural compounds with antidiabetic action. Notulae Botanicae Horti Agrobotanici Cluj-Napoca. 2012; 40 (1):314-325 - 6.
American Diabetes Association. Diagnosis and classification of diabetes mellitus. Diabetes Care. 2014; 37 (Suppl. 1):S81-S90 - 7.
Pereira DF, Cazarolli LH, Lavado C, Mengatto V, Figueiredo MSRB, Guedes A, et al. Effects of flavonoids on α-glucosidase activity: Potential targets for glucose homeostasis. Nutrition. 2011; 27 (11-12):1161-1167 - 8.
Ansari P, Choudhury ST, Seidel V, Rahman AB, Aziz MA, Richi AE, et al. Therapeutic potential of quercetin in the management of type-2 diabetes mellitus. Life. 2022; 12 (8):1146 - 9.
Dhanya R. Quercetin for managing type 2 diabetes and its complications, an insight into multitarget therapy. Biomedicine & Pharmacotherapy. 2022; 146 :112560 - 10.
Shi GJ, Li Y, Cao QH, Wu HX, Tang XY, Gao XH, et al. In vitro and in vivo evidence that quercetin protects against diabetes and its complications: A systematic review of the literature. Biomedicine & Pharmacotherapy. 2019; 109 :1085-1099 - 11.
Maalik A, Khan FA, Mumtaz A, Mehmood A, Azhar S, Atif M, et al. Pharmacological applications of quercetin and its derivatives: A short review. Tropical Journal of Pharmaceutical Research. 2014; 13 (9):1561-1566 - 12.
Prysyazhnyuk V, Voloshyn O. Effects of comprehensive treatment with quercetin administration on biochemical blood parameters and pro-and anti-inflammatory cytokines in nonalcoholic fatty liver disease patients. The Pharma Innovation Journal. 2017; 6 :386-389 - 13.
Azeem M, Hanif M, Mahmood K, Ameer N, Chughtai FRS, Abid U. An insight into anticancer, antioxidant, antimicrobial, antidiabetic and anti-inflammatory effects of quercetin: A review. Polymer Bulletin. 2023; 80 (1):241-262 - 14.
Yi H, Peng H, Wu X, Xu X, Kuang T, Zhang J, et al. The therapeutic effects and mechanisms of quercetin on metabolic diseases: Pharmacological data and clinical evidence. Oxidative Medicine and Cellular Longevity. 2021; 2021 :6678662 - 15.
Goldberg DM, Yan J, Soleas GJ. Absorption of three wine-related polyphenols in three different matrices by healthy subjects. Clinical Biochemistry. 2003; 36 (1):79-87 - 16.
Srinivasan P, Vijayakumar S, Kothandaraman S, Palani M. Anti-diabetic activity of quercetin extracted from Phyllanthus emblica L. fruit: In silico and in vivo approaches. Journal of Pharmaceutical Analysis. 2018;8 (2):109-118 - 17.
Mohammad A, Sharma V, McNeill JH. Vanadium increases GLUT4 in diabetic rat skeletal muscle. Molecular and Cellular Biochemistry. 2002; 233 :139-143 - 18.
Eid HM, Martineau LC, Saleem A, Muhammad A, Vallerand D, Benhaddou-Andaloussi A, et al. Stimulation of AMP-activated protein kinase and enhancement of basal glucose uptake in muscle cells by quercetin and quercetin glycosides, active principles of the antidiabetic medicinal plant vaccinium vitis-idaea. Molecular Nutrition & Food Research. 2010; 54 (7):991-1003 - 19.
Li JM, Wang W, Fan CY, Wang MX, Zhang X, Hu QH, et al. Quercetin preserves β-cell mass and function in fructose-induced hyperinsulinemia through modulating pancreatic Akt/FoxO1 activation. Evidence-based Complementary and Alternative Medicine. 2013; 2013 :303902 - 20.
Adewole SO, Caxton-Martins EA, Ojewole JA. Protective effect of quercetin on the morphology of pancreatic β-cells of streptozotocin-treated diabetic rats. African Journal of Traditional, Complementary and Alternative Medicines. 2007; 4 (1):64-74 - 21.
Choi HN, Jeong SM, Huh GH, Kim JI. Quercetin ameliorates insulin sensitivity and liver steatosis partly by increasing adiponectin expression in ob/ob mice. Food Science and Biotechnology. 2015; 24 :273-279 - 22.
Jo SH, Ka EH, Lee HS. Comparison of antioxidant potential and rat intestinal α-glucosidases inhibitory activities of quercetin, rutin, and isoquercetin. International Journal of Applied Research in Natural Products. 2009; 2 :52-60 - 23.
Soltesova-Prnova M, Milackova I, Stefek M. 3′-O-(3-Chloropivaloyl) quercetin, α-glucosidase inhibitor with multi-targeted therapeutic potential in relation to diabetic complications. Chemical Papers. 2016; 70 (11):1439-1444 - 24.
Kim JH, Kang MJ, Choi HN, Jeong SM, Lee YM, Kim JI. Quercetin attenuates fasting and postprandial hyperglycemia in animal models of diabetes mellitus. Nutrition Research and Practice. 2011; 5 (2):107-111 - 25.
Agarwal N, Sharma AK, Agarwal P, Sharma MK, Awasthi N, Mishra A, et al. Evaluation of kidney and liver disorders In type 2 diabetic patients using USG. Evaluation. 2015; 21 (2):265-271.26 - 26.
Dias AS, Porawski M, Alonso M, Marroni N, Collado PS, Gonzalez-Gallego J. Quercetin decreases oxidative stress, NF-κB activation, and iNOS overexpression in liver of streptozotocin-induced diabetic rats. The Journal of Nutrition. 2005; 135 (10):2299-2304 - 27.
Sirovina D, Oršolić N, Končić MZ, Kovačević G, Benković V, Gregorović G. Quercetin vs chrysin: Effect on liver histopathology in diabetic mice. Human & Experimental Toxicology. 2013; 32 (10):1058-1066 - 28.
Elbe H, Vardi NİGAR, Esrefoglu MUKADDES, Ates B, Yologlu S, Taskapan C. Amelioration of streptozotocin-induced diabetic nephropathy by melatonin, quercetin, and resveratrol in rats. Human & Experimental Toxicology. 2015; 34 (1):100-113 - 29.
Thomas AA, Feng B, Chakrabarti S. ANRIL: A regulator of VEGF in diabetic retinopathy. Investigative Ophthalmology & Visual Science. 2017; 58 (1):470-480 - 30.
Kumar B, Gupta SK, Srinivasan BP, Nag TC, Srivastava S, Saxena R, et al. Hesperetin rescues retinal oxidative stress, neuroinflammation and apoptosis in diabetic rats. Microvascular Research. 2013; 87 :65-74 - 31.
Huang J, Huang K, Lan T, Xie X, Shen X, Liu P, et al. Curcumin ameliorates diabetic nephropathy by inhibiting the activation of the SphK1-S1P signaling pathway. Molecular and Cellular Endocrinology. 2013; 365 (2):231-240 - 32.
Sonawane RD, Vishwakarma SL, Lakshmi S, Rajani M, Padh H, Goyal RK. Amelioration of STZ-induced type 1 diabetic nephropathy by aqueous extract of Enicostemma littorale Blume and swertiamarin in rats. Molecular and Cellular Biochemistry. 2010; 340 :1-6 - 33.
Haneda M, Koya D, Kikkawa R. Cellular mechanisms in the development and progression of diabetic nephropathy: Activation of the DAG-PKC-ERK pathway. American Journal of Kidney Diseases. 2001; 38 (4):S178-S181 - 34.
Nelli GB, Anand Solomon K, Kilari EK. Antidiabetic effect of α-mangostin and its protective role in sexual dysfunction of streptozotocin induced diabetic male rats. Systems Biology in Reproductive Medicine. 2013; 59 (6):319-328 - 35.
Niwas Jangir R, Chand Jain G. Diabetes mellitus induced impairment of male reproductive functions: A review. Current Diabetes Reviews. 2014; 10 (3):147-157 - 36.
Ghosh S, Banerjee S, Sil PC. The beneficial role of curcumin on inflammation, diabetes and neurodegenerative disease: A recent update. Food and Chemical Toxicology. 2015; 83 :111-124 - 37.
Peeyush KT, Gireesh G, Jobin M, Paulose CS. Neuroprotective role of curcumin in the cerebellum of streptozotocin-induced diabetic rats. Life Sciences. 2009; 85 (19-20):704-710 - 38.
Costa LG, Garrick JM, Roquè PJ, Pellacani C. Mechanisms of neuroprotection by quercetin: Counteracting oxidative stress and more. Oxidative Medicine and Cellular Longevity. 2016; 2016 :2986796 - 39.
Hu T, Lu XY, Shi JJ, Liu XQ , Chen QB, Wang Q , et al. Quercetin protects against diabetic encephalopathy via SIRT1/NLRP3 pathway in db/db mice. Journal of Cellular and Molecular Medicine. 2020; 24 (6):3449-3459 - 40.
Kandhare AD, Raygude KS, Kumar VS, Rajmane AR, Visnagri A, Ghule AE, et al. Ameliorative effects quercetin against impaired motor nerve function, inflammatory mediators and apoptosis in neonatal streptozotocin-induced diabetic neuropathy in rats. Biomedicine & Aging Pathology. 2012; 2 (4):173-186 - 41.
Li C, Zhao X, Zheng H, Cai F. GW28-e0635 quercetin retards progression of diabetic cardiomyopathy through modulations of SIRT1 and AMP-activated protein kinase. Journal of the American College of Cardiology. 2017; 70 (16S):C63-C64