Common types of breeding schemes according to the number of fish and number of crosses.
Abstract
Fish facilities often maintain tens to hundreds of zebrafish lines, among transgenic, mutant and wild types. The replicability and reproducibility of the research based on these animals relies on its quality. However, keeping fish lines healthy and genetically stable across generations is not trivial. Housing space is often limited, so each stock is frequently restricted to a few tens of animals and inbreeding depression can rapidly install, causing health issues, gender bias and decreased reproductive performance. To minimise this, it is fundamental to define colony management strategies adequate to each fish line and each facility. This chapter discusses common difficulties and approaches to overcome them, including line-specific mating schemes and complementary actions to keep lines hardy. In addition, strategies to prevent genetic cross contaminations and record keeping methods are also addressed as part of a complete colony management program.
Keywords
- replicability
- reproducibility
- line maintenance
- inbreeding depression
- genetic variability
- databases
1. Introduction
With the rapid evolution of efficient gene-editing tools, the number of new transgenic and mutant zebrafish lines has increased steeply, contributing to the acceleration of scientific knowledge on a wide range of research fields. In parallel, it also brought challenges to the management of fish colonies since the physical space in fish facilities is often limited, each fish line has specific features to account for and maintaining genetically stable lines to guarantee the quality of scientific outputs is not an easy task. To keep up with the increasingly demanding research, it is crucial that zebrafish facilities implement custom-designed colony management programmes, regardless of the number of fish and fish lines held.
The largest share of a colony management program is line maintenance, which refers to the actions required to ensure that a line, including its genomic and phenotypic traits, is preserved across generations. Those actions include selecting broodstock and breeding schemes, defining criteria for offspring selection and determining the periodicity to launch a new generation. Mating schemes can be based on two main categories: inbreeding, which is the mating of individuals more closely related than the population average (also known as incross), and outbreeding, the mating of individuals less closely related than the population average (also known as outcross) [1].
In species such as mice and medaka, breeding plans are often based on inbreeding. Briefly, close relatives share many alleles for multiple genes, so when they are incrossed, at least some progeny will inherit the same alleles from both parents, increasing homozygosity at different loci and reducing the genetic diversity among the offspring. When a line reaches 98% homozygosity, typically after 20 consecutive generations of full sibling matting and thorough selection of the best breeders and traits of interest and against harmful phenotypes, it is defined as an ‘inbred strain’, often abbreviated to ‘strain’ [2]. At that point, the strain is considered genetically homogeneous with little or no phenotypic variance, and the individuals are practically clones of each other. Given the influence that the genome has on physiology and behaviour, the genetic stability of inbred strains is a great advantage, reducing uncontrollable variability and increasing the statistical power of experimental results and their interpretation, replicability and reproducibility [3]. Ultimately, allows a faster transition from basic to translational studies and eventually ‘real life’ applications.
As for zebrafish, there have been several attempts to create inbred strains such as the C32 [4], SJD [5], CG1/CG2 [6], IM12m and IM14m lines [7], some of which continue to be available at international resource centres. Nevertheless, consecutive filial incross in zebrafish can produce detrimental effects on fish development and reproduction that become incompatible with the continuity of the lines after just a few generations, a phenomenon known as inbreeding depression.
The absence of zebrafish inbred strains created a recognised problem for reliability of the outcomes from research based on this model. This is because fish from the same lines but maintained in different facilities are many times genetically distinct [8], making comparisons between experimental results questionable. An alternative to inbred strains could be the creation of genetically defined lines. Actually, some lines were created and extensively characterised at the genomic level at a defined generation, namely, SAT [2], NHGRI-1 [9] and T5D. However, opposite to inbred strains, the fish that compose these lines conserve a considerable degree of allelic variability that originate changes in the line genetic composition across generations due to the natural allele recombination that occur at meiosis. Thus, to become real assets, they would need to be under continuous genetic monitoring to keep the genetic map updated, which has not been the case mostly due to the high costs associated and lack of expertise on how to perform such monitoring [3].
While there is no consensus solution to the concern regarding the reliability of experimental data obtained from zebrafish, fish facilities put efforts on keeping their colonies healthy and reproductively active based on somewhat opposite strategies that hinge on allelic diversity preservation [2]. By doing so, there is also a reduction of the natural genetic divergence between fish from the same lines that are held at different facilities, which is another positive contribution to data reproducibility and replicability [10, 11]. In the next sections of this chapter, common difficulties and current approaches for the management of wild-type, transgenic and mutant colonies are described.
2. Inbreeding depression and genetic drift
The main reason why it is so difficult to generate and maintain zebrafish inbred lines is because inbreeding frequently produces deleterious phenotypes well before the 20th filial generation. The phenomenon, also known as inbreeding depression, is defined as the loss of fitness in a population submitted to increasing homozygosity [12]. Signs of inbreeding depression include gender biases (typically more males); decrease in fertility, growth and survival; more frequent and severe developmental defects (e.g., small head, underdeveloped lower jaw and poor circulation) and shorter lifespan [1, 7, 13], and ultimately, it may even lead to fish lines’ extinction. Inbreeding morphological abnormalities tend to become evident after six to eight generations [13]. Some malformations may become more pronounced and evident as fish age advances and may be associated with inbreeding, though not genetically confirmed so far. These include skeletal malformations, such as spinal curvatures in one or multiple sites (Figure 1B); caudal fin developmental deformities (Figure 1C) and craniofacial phenotypes that comprise shortening of the frontonasal area (Figure 1D), terminal infolding of the opercular apparatus (Figure 1E) and hypo- or hypertrophy of the jaw (Figure 1F) [14].
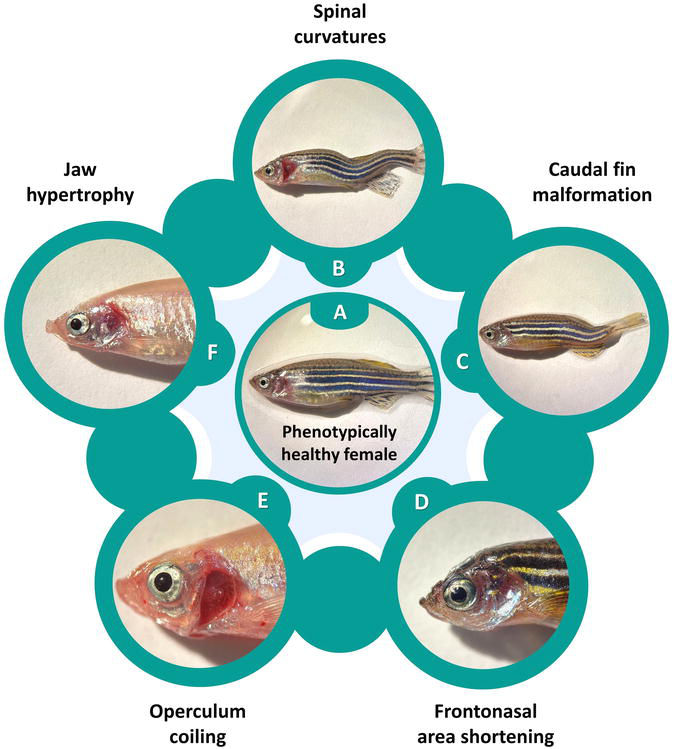
Figure 1.
Phenotypes possibly associated with inbreeding depression in adult zebrafish. A – Control, phenotypically healthy female; B – Spinal curvatures in multiple sites; C – Caudal fin developmental malformation; D – Frontonasal area shortening; E – Operculum coiling; F – Jaw hypertrophy.
The origin of inbreeding depression stands on the mating of closely related fish, such as the cross of full siblings or parents with their progeny. When that happens, deleterious recessive alleles that are present as single copies on each parent can be paired and expressed in their offspring, being the accumulated expression of harmful alleles the cause behind inbreeding depression. In addition, there are studies that indicate that inbreeding depression can also occur as a consequence of some allele combinations being superior in terms of the benefits for the animal when they are expressed in heterozygosity, so the increase in homozygosity lowers population fitness. More studies are required, but if this is the case, inbred lines should always have inferior genetic fitness due to the loss of heterozygosity, which would explain the failure in creating zebrafish inbred strains [15].
Another natural factor that negatively affects the maintenance of healthy animal stocks is genetic drift, defined as random changes in gene frequency over several generations, leading to a decline in the population’s genetic diversity and occasional irreversible loss of alleles [1]. It is considered a twin sister of inbreeding depression, since the latter accentuates the former. In fact, the primary source of genetic variation within a zebrafish line is often an extreme case of genetic drift designated as ‘founder effect’ that happens upon the establishment of a new colony from a few imported animals, or when a subset of fish is selected as spawners for the next generation. Briefly, it is the random allelic variation derived from the split of the original population into smaller groups that are not genetically representative of the first population [16]. Instead, each group holds a different subset of alleles and some get lost.
Inbreeding depression and genetic drift may affect not only the population’s fitness but also the replicability and reproducibility of experimental results. Both happen more intensely in small populations; hence, the facility dimension and size of the colony for each line have to be considered from the earliest times in the design of a colony management programme.
3. Line maintenance strategies
The term ‘colony management’ is often used as a synonym for line maintenance. In our view, the former includes the latter as well as additional topics that are addressed in this chapter. Line maintenance commonly refers to the controlled cycle that ensures the continuity of a line across as many generations as intended or possible. It includes four main stages: the maintenance of adult fish, breeding, larvae selection and rearing and finally the replacement of the parent stock by the new generation (Figure 2). The frequency of this cycle is determined by the required replacement rate, defined by either the researcher’s experimental needs or the age limit imposed by the facility (typically 15 to 18 months of age) as a preventive health measure, since older fish are more susceptible to disease and spawning efficiency is lower [17, 18].
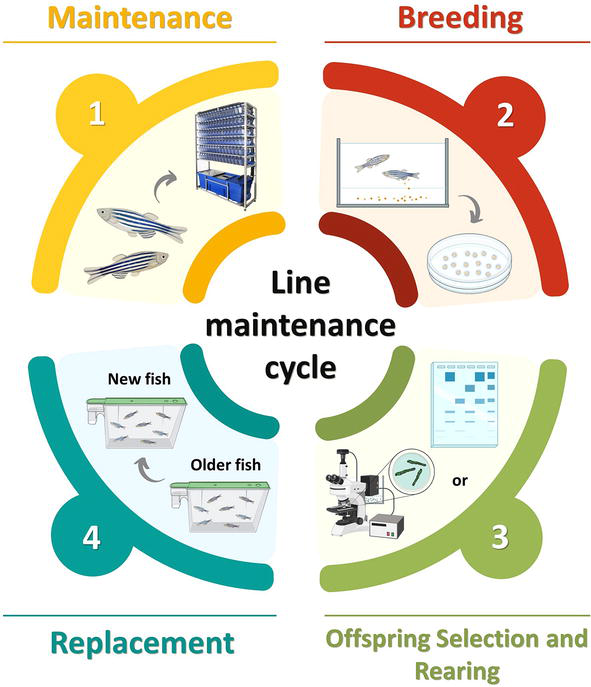
Figure 2.
Line maintenance cycle main stages. (1) adults are housed and kept in holding tanks. Some can be used for experiments as long as there is still enough broodstock for line propagation. (2) fish are bred by predefined methods at scheduled intervals and embryos collected. (3) embryos are incubated at 28°C until 5 days post fertilisation and selected before entering a nursery holding system. (4) older fish are replaced by the next generation typically at 15 to 18 months of age, since they become more susceptible to disease.
Line maintenance strategies need to be fish line-specific; there is no ‘one fits all’ formula. This includes defining the breeding scheme and the criteria for breeder and offspring selection, which can have a direct effect on the extent of inbreeding depression and genetic drift per generation. In addition, it is wise to consider the possible need for special housing and husbandry of more delicate lines, such as, for example, immunodeficient or tumour carriers. By doing so, it is possible to prevent welfare and survival issues that could increase morbidity and mortality, causing indirect negative impact on the maintenance of future generations. All these decisions should take into consideration the type of line (wild type, transgenic or mutant), intended zygosity, available housing space, pedigree record keeping and experimental needs.
3.1 Overview of zebrafish breeding in a laboratory setting
Understanding zebrafish breeding in the wild provides a good base for the development of breeding methods in captivity. Zebrafish breeding is influenced by intrinsic factors difficult to control such as mate choice, courtship and social behaviours, as well as by environmental aspects that can be adjusted in a facility, like water chemical and physical parameters, amount and type of food, vegetation and volume of water mass [19]. A number of decisions need to be made before setting a cross, depending on its purpose: the type of tank to use, number of fish, sex ratio and genetic aspects like fish pedigree and genotype of interest.
In terms of physical setup options, crosses can be set directly in the housing system, in large isolated spawning apparatuses that can be used in recirculating or static mode, or in static breeding tanks. The first two options hold larger broodstocks and provide better control over water quality stability, so they are preferred when fish are left to breed for more than one overnight. Zebrafish also seem to prefer larger volumes of water for spawning [19]. There are several models of mass spawning tanks on the market with holding capacity up to 300 L and ability to hold up to some hundreds of fish [10, 19]. On the other hand, 0.7 to 2 L breeding tanks with static water freshly collected from the housing system are the most used option. They are available in different sizes and designs, are easy to set, disassemble and clean and their small size eases handling and embryo collection.
Most breeding tanks come with an inner insert tank with perforated bottom that allows embryo passage and their accumulation on the outer tank. This is because zebrafish show no parental care behaviour, so eggs need to be protected from being eaten by the adults. In nature, zebrafish are regularly found in shallow slow-flowing waters, so some inner tanks have a tilted bottom that creates low-depth areas. Many spawning systems also include a divider insert to keep males and females separated when a controlled and synchronous spawning is required. Physical enrichment is also encouraged to create spawning sites that meet zebrafish preference. This includes gravel or gravel-like images at the bottom of the tanks, non-toxic plastic shelters and plastic plants to provide hiding sites and decrease male aggression [20]. Crosses are typically set in the afternoon or early evening and left undisturbed until the following morning; then, fish spawning is stimulated by dawn [2]. Males tend to be territorial, which interferes with female oviposition [20], the reason why a female:male ratio of 2:1 is generally recommended.
3.2 Breeding and offspring selection plans
Good planning of the crossing strategy and progeny selection method for each fish line is the best insurance against inbreeding depression and genetic drift accumulation to deleterious levels.
Ideally, the starting point should be to define, for each fish line, the level of inbreeding and genetic drift one is willing to accept over a certain number of generations. Based on that, there are specific equations to estimate the number of breeders that are required on each generation to fulfil those genetic criteria. In an ideal population, all individuals equally contribute genes to the next generation. However, most real populations are far from ideal and individual contributions to the offspring vary considerably. Consequently, factors such as type of cross, broodstock number and sex ratio, spawning success ratio, offspring viability and selection strategy need to be included in the equation when calculating the number of breeders to use in order to preserve the genetic pool of the line [1, 21, 22]. Realistically, the vast majority of the zebrafish facilities do not follow the ideal approach. The equations are complex, not widely divulgated or discussed in zebrafish meetings and require detailed information that not all facilities keep. On top of that, housing space per fish line is frequently limited and consequently broodstock number is insufficient for ideal line propagation plans. Fortunately, there are alternative strategies to preserve allele variability for several generations.
The first thing to do is to find how many females and males compose the broodstock of the line of interest. The larger the colony, the easier the maintenance of genetic variability across generations. Second, decide the crossing unit—single pair or bulk cross. And finally, establish how many mating units will contribute progeny to the next generation and how many crosses need to be set considering the expected spawning success. Different combinations of these variables is what defines the most common types of breeding schemes (Table 1). The specific breeding schemes implemented depend greatly on the fish line and colony size.
Type of cross | Single pair | Small bulk cross | Large bulk cross | Multiple paired crosses | Multiple units of small bulk crosses |
---|---|---|---|---|---|
Work effort | Low | Low | Moderate | High | High |
Number of breeders | One pair | ≤ 15 | > 40 | As many cross units as possible | As many cross units as possible |
Female:male ratio | 1:1 | ~2:1 | ~2:1 | 1:1 | ~2:1 |
Offspring selection | All progeny are full siblings | No control over the number of effective spawners | No control over the number of effective spawners | Controlled mix of progeny from all spawning units | Controlled mix of progeny from all spawning units |
Genetic diversity obtained | Very low | Low | High – Very high | High – Very high | High – Very high |
Table 1.
Single pair cross (Figure 3A) is the mating of one female with one male. The pair can produce hundreds of eggs in a single spawning event [10], yet genetic variability in the offspring is limited to the alleles present in the two parents. The method is useful for individual screening of adults based on their progeny’s phenotype or genotype. This is required during the establishment of a new fish line, to find founders with germline transmission of the feature, mutation or transgene of interest. In addition, it is helpful to identify intruders upon accidental mix of adult fish from lines that look alike at visual inspection but carry different transgenes or mutations that are expressed in larvae. In those cases, a cross is set between the candidate under screen and a fish with a known genotype (often a wild type), and the progeny is screened: if the phenotype or genotype of interest is found, then the parent under screen is positive for the feature of interest. Single pair mating is also the best choice for establishing new lines from single founders with unique expression patterns and genomic features [2]. Still, if a single pair is used to launch a new generation, there is a steep reduction in allelic diversity known as founder effect, which greatly increases the rate of genetic drift and inbreeding depression [1, 10].
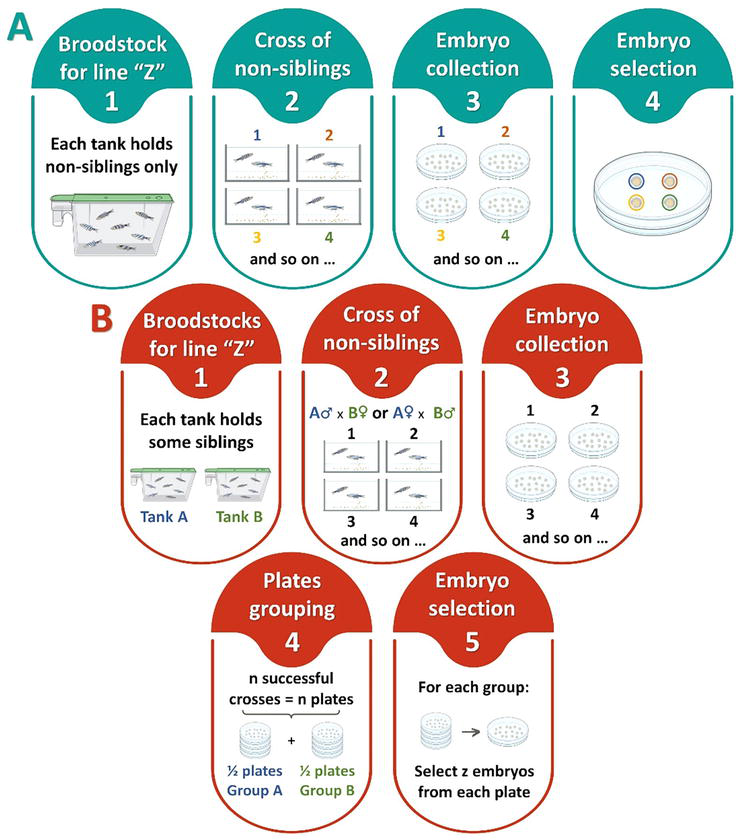
Figure 3.
Line propagation schemes based on pedigree mating and two progeny selection methods. (A) (1–2) pair-up (single pairs) or group-up (bulk crosses) fish from the same tank. (3) collect embryos from each mating unit separately, and (4) mix one embryo from each cross into one plate. Repeat to create as more non-sibling plates as needed for research. Raise the fish from each plate in separate tanks. Repeat the process every generation. Adapted from [
Another breeding and offspring selection method is pedigree mating. It is a time-consuming strategy but effective in keeping even small populations fit for multiple generations as it meets the two fundamental criteria: the entire broodstock must be genetically represented in the following generation—to preserve the line genetic variability—and the mating fish cannot be closely related—to minimise inbreeding depression [1]. Respecting these criteria is not trivial given the difficulty in keeping track of the pedigree of zebrafish individuals, since they should not be housed alone due to their shoaling nature. On top of that, there is no zebrafish equivalent to mice ear punches for individual tracing within a group because the fins regenerate in a matter of days. Yet pedigree mating overcomes such obstacles. This method is depicted on Figure 3A. It starts with the setting of several single pair crosses, each composed of one female and one male that are not closely related (not full siblings or parent–progeny). The following day, after fish spawning, the progeny from each pair is collected into separate petri dishes. Within the next few days, larvae are screened for the traits of interest and one individual from every cross is selected and pooled together into a new petri dish. Finally, the selected group is transferred from the petri dish into a holding tank and raised as the new generation of the fish line of interest [23]. If the number of fish to raise exceeds the capacity of one holding tank, additional tanks can be set up following the same mating and selection approach. The method ensures that there are no full-siblings within each tank, so when they are adults and the line needs to be refreshed with a new generation, the fish within each tank can be paired up in mating tanks, restarting the breeding and selection cycle.
When the number of breeding pairs is lower than the number of fish that need to be raised, it is not possible to select just one embryo per crossing pair as previously described, yet control over pedigree is still recommended. In this case, a variation of the former method can be applied, as follows: multiple crosses of single pairs are set using females and males from non-sibling tanks, and the embryo clutches from the different breeding pairs are collected into separate petri dishes (Figure 3B, panels 1–3). Then, the colony manager defines the number of tanks, greater than one, where the larvae will be raised, and assigns a different subset of the collected clutches to each tank (Figure 3B, panel 4). Next, a few siblings per petri dish within each subset are selected, mixed and finally transferred to the corresponding holding tank, to be raised (Figure 3B, panel 1 and 5). The number ‘z’ of larvae selected per clutch depends on how many clutches are assigned to each tank, the size of the tanks and the intended fish holding density. As a result of this selection approach, each tank will hold a unique mix of siblings from different progenitors. Therefore, to avoid sibling mating when the next generation of the fish line needs to be established, all the new breeding pairs should include a female from one tank and a male from another.
Since zebrafish are shoaling fish known to naturally spawn in large groups, if we are not facing any broodstock number limitation, large bulk spawning in tanks of 40–300 L capacity is less time-consuming. Compared to pair crossing approaches, it increases fertilisation efficiency, provides better batches of viable embryos and enhances the population’s genetic variability [19]. This approach is more frequently carried out in large-scale facilities, since it requires large broodstocks. More than 10,000 embryos can be collected using mass spawning tanks, in just a 10-minute period [10]. For small fish stocks, the strategy can be downsized and small bulk crosses set holding around 5 to 15 fish with a ~ 2:1 female:male ratio, which takes less time to set and house than multiple units of single pairs. However, group crosses have some downsides: it is not possible to know the individual contribution of each brood fish to the progeny or how many fish actually spawned. Consequently, the offspring selection is random and there is no direct control over a population’s genetic diversity. The chance of selecting some siblings is real, especially in small bulk mattings and when the number of offspring selected to propagate the line is low. To overcome that, it is possible to set multiple small bulk crosses and follow the same rationale for progeny selection as for multiple paired crosses (Figure 3). By doing so, the chance of spawning success on each mating unit is higher. In addition, if the progeny selection strategy includes the collection of more than one embryo per cross (Figure 3B), there is a possibility of selecting non-siblings from each crossing unit, further contributing for a more genetically diverse group of fish.
3.2.1 Wild-type lines
There are plenty of reasons to maintain wild-type lines with good fitness and high genetic variability. The wild-type zebrafish maintained in research facilities are the closest representations of the species in the wild. Keeping these lines robust and genetically diverse is crucial for reproducible experimental outcomes which, in turn, is key for validating scientific evidence [3]. Aside from direct experimental use, wild-type embryos are the most common base for the generation of transgenic and mutant lines upon delivery of the molecular components required for the intended genetic modification into the embryos. Additionally, once established, genetically modified lines are often maintained in heterozygosity by crossing with a wild-type line as a means of avoiding inbreeding depression [2, 23].
Strategies for breeding wild types are typically adaptations of crosses described in Section 3.2. The decision depends greatly on the size of the stocks that each facility holds, but the goal should always be to preserve as many alleles as possible across generations. Only phenotypically healthy larvae should be selected.
3.2.2 Transgenic lines
Maintenance of transgenic lines requires the preservation of the transgene or transgenes of interest while keeping genetic diversity of the remaining genome. A simple yet effective strategy is to maintain the transgenic fish in heterozygosity through outcross with a wild-type line. At each line maintenance cycle, the wild-type fish used should have little or no relation to the wild-type individuals used to set the former transgenic generation, and offspring should be carefully screened. In addition, including cross units with transgenic males and wild-type females and other units with the opposite can avoid the loss of genetic or epigenetic information transmitted to the offspring by one of the sexes alone [24, 25].
Another approach is to incross the line for several consecutive generations using mating schemes that involve multiple crossing units. The progeny will include embryos that are homozygous for the transgene and by selecting only those embryos to raise, its expression gets stronger. This is an interesting option, for example, when transgene expression is detected by dim fluorescent reporters or when it silences fast (e.g., UAS reporters) and to increase confidence in phenotypic screens because homozygous are often easier to identify. Moreover, researchers may require the propagation of lines in homozygosity for experiments. The downside of successive incrosses is that to keep fish away from inbreeding depression, the transgenic lines need to maintain a broodstock larger than the capacity of many facilities, whether that is due to space limitations, budget constraints, 3Rs policies or a combination of factors. In those cases, a good option is to intercalate incross with outcross to a robust wild-type line every other generation, or at least every 1 to 3 generations of incross, for the introduction of new alleles (Figure 4) [23].
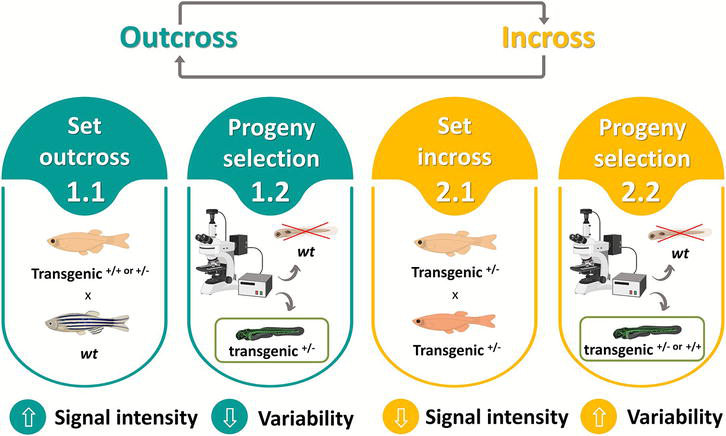
Figure 4.
Example of a line maintenance approach for transgenic zebrafish. (Outcross, 1.1) transgenic fish are outcrossed with a robust wild-type line. (1.2) the heterozygous progeny is selected, raised and maintained as broodstock or for experiments. (Incross, 2.1) when a new generation is required, the adult heterozygous transgenics are incrossed and (2.2) the best offspring for the trait of interest are raised. It may be possible to distinguish phenotypically homozygous from heterozygous fish, otherwise they can be raised together and addressed to transgene positives. Incrosses should be alternated with outcross every other generation or at least every 1 to 3 generations of incross, to keep the colony hardy.
Once offspring are obtained, it is necessary to select the individuals to raise. Screening approaches vary with the transgene and also if discrimination of zygosity matters. When the transgene has a fluorescent reporter, it is possible to preserve the genotype across generations without genotyping, by raising only the fish with the brightest fluorescence and complete expression pattern. If the number of fish that respect those criteria is lower than the required, it is worth repeating the cross until reaching the target number, instead of selecting suboptimal fish. A similar rationale can be applied to transgenes with other traceable phenotypes.
In some transgene expression systems, such as Cre-loxP, Gal4-UAS and Q system, the promoter and the reporter are inserted into individual constructs that may be delivered to separate fish [26, 27, 28]. This increases the number of possible combinations between promoter and reporter upon outcrossing the lines with the elements of interest. Yet there is no direct way to identify the fish that carry each element alone, because the expression of the reporter only happens in fish that carry both components. A practical solution is to include in the construct of each element (promoter or reporter) an expression cassette for a fluorescence marker, such as a heart or retina marker that will be expressed in all the fish that carry that element [29, 30]. Alternatively, select embryos based on good health and screen them individually upon sexual maturity. For that, mate them in single pairs with fish that carry the complementary element of the transgene expression system and analyse the progeny: if at least some of the progeny express the expected phenotype, then the parent under screen is positive for the transgene.
Single paired crosses and progeny analysis can also be used to learn parental zygosity without the need to genotype by molecular methods, as long as the transgene of interest has a detectable phenotype. The mating pair needs to be negative for the transgene of interest and similar phenotypes. On top of that, if screening for one of the elements of a two-component transgene expression system, the mating partner also needs to be homozygous for the element complementary to that under screen. Then, if all the offspring exhibit the phenotype of interest, the parent under screen is homozygous for the transgene; if just part of the progeny expresses it, then the parent is heterozygous.
Finally, for transgenes not plausible of phenotypic screen, the solution is to collect a biological sample for total DNA extraction and processing for genotyping. The DNA source can be either whole embryos, larvae finfold, adult fin biopsies, skin swabs or the bathing water of larvae subject to microfluidic harmonic oscillation. The latter two are less-invasive so less likely to cause pain to the animals [31, 32, 33, 34].
3.2.3 Mutant lines
Like for transgenics, preservation of mutant zebrafish across generations involves the conservation of the mutation at the same time that the genetic diversity of the remaining genome is preserved. If a mutation is lethal in heterozygosity, it is not possible to raise a line, but lines with lethal recessive mutations can be maintained in heterozygosity by outcrossing with a different line (typically a wild type) using the breeding scheme that best meets the needs. The offspring is then screened to distinguish mutation carriers from fish that only have the wild alleles [10]. Screening relies on phenotyping or genotyping depending if there is a detectable phenotype or not. For non-lethal mutations, a similar outcross approach can be used to preserve the lines as long as there is not a research need for homozygosity. In fact, keeping mutations in heterozygosity is best for prevention of unexpected harmful phenotypes. The downside is that screens can become expensive if there is not a phenotype that is easy to trace, because it is necessary to run genotyping every time a new generation is raised.
To cut screening time and costs, or if research demands it, viable mutations can also be propagated by incross of the homozygous fish. In this case, all offspring are also homozygous for the mutation, so there is no need for screens other than selecting individuals that look robust. However, unless fish numbers are large, inbreeding escalates steeply. So, a compromise approach between reduction of screening costs and maintenance of line hardiness is to use a two-generation cycle that includes an additional and optional sidestep, as depicted in Figure 5.
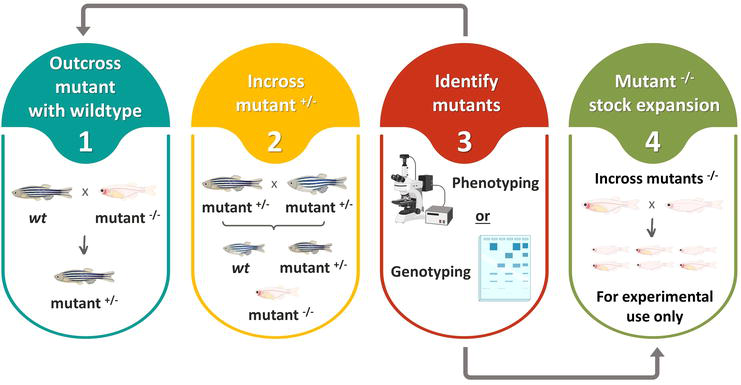
Figure 5.
Example of a cost-effective line maintenance approach for mutant zebrafish. (1) mutants (homozygous) are outcrossed with a robust wild-type line and the heterozygous progeny is raised and (2.1) incrossed when it is time to launch the next generation. (2.2) homozygous mutants are identified by phenotyping or by genotyping and are used to restart the cycle on step 1. In parallel, the screened and raised homozygous mutants can be (3) incrossed for direct mutant stock expansion for experimental use. These fish should not be included in the line maintenance cycle.
It starts by outcrossing homozygous mutants with a wild type so that all offspring are heterozygous for the mutation (no need to screen) and genome diversity is refreshed by the alleles passed on by the wild-type parents. For the next generation, incross the heterozygous and screen the offspring for homozygous mutants. For cost reduction, screen for a low number of mutants of each gender. Then, the line propagation cycle restarts using multiple paired mattings between each mutant and a wild type. This way, the reduction in allele diversity caused by mutant colony downsizing is compensated by the new alleles brought by the outcross. The sidestep is introduced when there is a demand for larger numbers of homozygous mutants. In that case, the screened mutants are incrossed for line expansion as a sidestep. Importantly, this group is not used for line propagation to avoid faster build-up of the line inbreeding.
3.3 Alternative breeding strategies
Having clear mating and screening strategies is fundamental but not always enough to ensure the continuity of fish lines throughout time. Factors like advanced age, isolation, stress, nutrition and health issues can lead to the decline of fish fitness independently of the size of the fish stock [2]. If fish fail to breed naturally,
A more complex approach is to cryopreserve sperm in liquid nitrogen [35, 37, 38]. Cryopreservation responds to the challenge of long-term storage of an ever-increasing number of fish lines in the limited space of fish facilities, by saving the time and space it takes for fish housing and husbandry. In addition, it meets two of the three 3Rs principles: ‘Reduction’, by allowing a decrease in the number of fish raised while still preserving lines that may be useful in future research, and ‘Replacement’ of fish shipping with cells shipping for sharing of lines between institutions. It can also be a way of preventing the loss of lines due to natural catastrophes, the spread of infectious diseases or failures in fish breeding capacity [39]. Importantly, in the context of maintenance of lines away from inbreeding, colony management programmes can include the sperm cryopreservation as soon as lines are established or soon after they are imported as a means of preserving genetic diversity before inbreeding and genetic drift act. Some generations later, those sperm samples can be used to reintroduce lost alleles through IVF using freshly collected oocytes. Programmes for cryopreservation of whole embryos are not a reality yet, but there is promising work in progress [39].
3.4 Complementary approaches against inbreeding
No matter what breeding strategy is applied, inbreeding and genetic drift are inevitable. As such, colony managers may want to complement line maintenance with additional measures that help keeping populations hardy. These include (a) reduction of stock renewal frequency by extending the lifetime of each generation within the limits of reproductive capacity, (b) increase of the number of fish maintained per line, (c) outcross of genetically modified lines with a robust wild type at least every 1–3 generations and (d) import fish, especially wild types, from health-accredited centres or trusted facilities every 1–3 years (larger stocks and with longer generation time require less frequent import); upon fish import, either outcross the new fish with the in-house ones to introduce new alleles, or replace the former stock completely if its fitness is very poor [10, 15, 40].
4. Prevention of genetic cross contamination
There are no standard guidelines on how to manage the daily activities of a fish facility, but prevention of genetic contamination, or line mixing, should always be a matter of concern. In many cases, fish from different lines look alike under visual inspection, so accidental mixes will not be noticed unless genetic screens are made and can jeopardise the accuracy of experimental results.
Centralising colony management on the fish facility staff is ideal to avoid errors; nevertheless, human resources are often insufficient to do so. Providing extensive training to researchers is also helpful. Two effective practices to save time and keep the focus on the ongoing task are to have fish containers clearly identified at all times and to have the most frequently required information readily available on tanks labels. It is also convenient to register all crosses that are set so that the genealogical history of each batch can be traced as a way of finding sources of genetic contamination [23]. Moreover, a close and open communication with all the facility users helps in identifying cases of line mixing faster, as well as learning more about the quality of the experimental fish. Finally, there are additional and simple actions that can be implemented to prevent genetic cross contaminations at different steps of the line maintenance cycle, as listed on Table 2.
Line maintenance step | Actions against genetic cross contamination |
---|---|
Crosses & housing | Bring one tank at a time to the working area. House it back before bringing the next one. Use one net per tank. |
Embryo plates cleaning | Use one pipette per plate. |
Surface disinfection | Ensure clear labelling of embryo containers to identify the corresponding fish lines. |
Phenotypic screens | Use one pipette per plate. Check fluorescence on all emission spectra present in lines housed at the facility. |
Genotypic screens | Identify tanks with the isolated fish. Ensure clear match between fish and genotyping samples. |
Larvae entrance in the holding system | Ensure clear correspondence between each embryos plate and the tank where they will be housed. |
Fish transfer to clean tank | Label the new tank before transferring the fish. Work one tank at a time. Use one net per tank. |
At all times, keep all fish containers clearly identified and avoid distractions |
Table 2.
Preventive actions against genetic cross contamination of fish lines during line maintenance.
5. Record keeping and databases
A colony management program is not complete without accurate records of a multitude of details that characterise the lines and influence its husbandry and maintenance cycle. No matter how many tanks and fish lines a facility has, it is valuable to keep as many records as possible. Relevant information often includes:
Date of crosses. Many facilities implement a minimal resting period of one to 2 weeks to allow the development of a new batch of gametes under no reproductive stress and to preserve the spawning capacity for longer since fish spawned more frequently have shorter reproductive span [2, 23].
Spawning success and embryo quality. Both inform about the breeding performance and the fitness of the animals. A simple way of assessing them is through code systems that indicate ranges of fertilised eggs (for spawning success) and ranges of survival rate at defined ages (for embryo quality).
Pedigree for each stock of a line. This is to avoid matting of closely related individuals. At least keep record of the parents for each new batch that is raised. From here, it is possible to trace back family trees.
Centralised line maintenance calendar. Examples of information to include for each line: line designation, propagation frequency, developmental stage for screening, screening method, what to screen for, month and year of birth of the latest generation and for launching the next one, lab that owns the line and person responsible for it and a comments section for relevant information.
Age of fish on each tank. As fish get older, spawning success and gametes quality decrease and susceptibility to pathogens is higher. Renewal of the lines’ stock is recommended in general after 1.5 years old [2, 23].
Health monitoring and clinical cases.
Survival rate at defined developmental stages (e.g., 30, 60, 90 days old).
Number of fish and sex ratio on each tank.
Information required for animal usage reports. Examples: number of animals used per experiment and per project, individual severity.
Location of single stocks within a holding system, to save time.
Line original lab and any paperwork related to fish imports or exports.
Notebooks, labels posted on the tanks’ outer surface, worksheets and spreadsheets are helpful and inexpensive to keep all records but are not efficient to navigate through complex data collected over years and for many fish lines. For that reason, digital databases were developed to save comprehensive and detailed records of fish lines [23, 41, 42, 43, 44, 45]. Databases are expected to compile many of the information listed above or more, to have extensive search data filter options and to connect to printers for creation of tank labels with the pre-defined information, such as the line name, stock number, date of birth, parents and name of the person responsible. Some databases also generate statistics and plots for different parameters. Options available include ZeBase [42], DanioData [40], Zebrabase [44], PyRAT Aquatic, Facile Fish, ZebraFish Database [43], ARMIS and FishNET [41]. Some are open-source software; others are commercially available at different price levels. The ones that can be opened on any device with a web browser allow for remote access. Regarding data storage, some databases include an option for storage on a web server running on a personal computer or in a dedicated server. Other software requires a full licence purchase, and others depend on variable dedicated computer storage capacities. Databases with good and fast technical assistance are always preferable.
6. Conclusions
Effective colony management programmes are complex yet necessary to maintain fish lines healthy and vigorous across many generations. Colony management programs should be designed and understood as part of the solution against the lack of genetically stable zebrafish lines, which is a recognised problem that limits the reliability of data produced from this animal model. Frequent genetic monitoring of fish lines could be the next best alternative, but its implementation as part of colony management programs is not standard yet and so was left out of this chapter. Breeding and embryo selection strategies are the heart of colony management plans and typically centred in the reduction of the deleterious effects of the inbreeding depression and genetic drift that inevitably accumulate as the number of generations of a fish line advance. Whatever the options implemented, line maintenance needs to take into account the interests of both the animals and the researchers. Fortunately, many times, the protocols that favour fish health and robustness are also the best for the production of solid experimental outcomes.
Fish facilities can house from a few units to thousands of different lines, many of which are phenotypically alike at visual inspection, which makes accidental mixes possible, no matter how careful people are. As such, it is important to assume that possibility and include in the colony management programme as many preventive actions as possible, as correction measures are much more time-consuming and possibly more expensive. As years go by and generations accumulate, the amount of relevant information also increases, including family trees that get more complex and observations on each fish line that may be relevant to adjust propagation protocols. Consequently, finding good record keeping methods to organise and save all that data is thus another topic to bring to the table while designing facility-specific colony management plans.
The methods and ideas present here are commonly applied as described, but the main goal of this chapter was to provide tools that can and should be adjusted according to the fish lines and conditions of each facility.
Acknowledgments
This work was developed with support from the Champalimaud Foundation (CF) and from the research infrastructure Congento, co-financed by Lisboa Regional Operational Programme (Lisboa2020), under the PORTUGAL 2020 Partnership Agreement, through the European Regional Development Found (ERDF) and Fundação para a Ciência e Tecnologia (FCT), Portugal, under the project LISBOA-01-0145-FEDER-022170. This work was also supported by Portuguese national funds, through FCT, in the context of the project UIDB/04443/2020.
The authors would like to thank the CF Research community and the CF Vivarium. A special thank you to the CF Fish Platform team, for all the support and team spirit. The authors also thank the Zebrafish Husbandry Association (ZHA) community for all the discussions and troubleshooting.
References
- 1.
Tave D. Inbreeding and Brood Stock Management. Food and Agriculture Organization of the UN; 1999. Fisheries Technical Paper. No. 392. Rome: FAO; 1999. 122 p - 2.
Nasiadka A, Clark MD. Zebrafish breeding in the laboratory environment. ILAR Journal. 2012; 53 :161-168. DOI: 10.1093/ilar.53.2.161 - 3.
Crim MJ, Lawrence C. A fish is not a mouse: Understanding differences in background genetics is critical for reproducibility. Lab Animal (NY). 2021; 50 :19-25. DOI: 10.1038/s41684-020-00683-x - 4.
Streisinger G, Walker C, Dower N, Knauber D, Singer F. Production of homozygous diploid zebra fish (Brachydanio rerio). Nature. 1981; 291 (5813):293-296. DOI: 10.1038/291293a0 - 5.
Johnson SL, Africa D, Home S, Postlethwait JH. Half-tetrad analysis in zebrafish: Mapping the ros mutation and the centromere of linkage group I. Genetics. 1995; 139 :1727-1735. DOI: 10.1093/genetics/139.4.1727 - 6.
Mizgirev I, Revskoy S. Generation of clonal zebrafish lines and transplantable hepatic tumors. Nature Protocols. 2010; 5 :383-394. DOI: 10.1038/nprot.2010.8 - 7.
Shinya M, Sakai N. Generation of highly homogeneous strains of zebrafish through full sib-pair mating. G3: Genes, genomes. Genetics. 2011; 1 :377-386. DOI: 10.1534/g3.111.000851 - 8.
Whiteley AR, Bhat A, Martins EP, Mayden RL, Arunachalam M, Uusi-Heikkilä S, et al. Population genomics of wild and laboratory zebrafish (Danio rerio). Molecular Ecology. 2011; 20 :4259-4276. DOI: 10.1111/j.1365-294X.2011.05272.x - 9.
Lafave MC, Varshney GK, Vemulapalli M, Mullikin JC, Burgess SM. A defined zebrafish line for high-throughput genetics and genomics: NHGRI-1. Genetics. 2014; 198 :167-170. DOI: 10.1534/genetics.114.166769 - 10.
Lawrence C. Advances in zebrafish husbandry and management. Methods in Cell Biology. 2011; 104 :429-451. DOI: 10.1016/B978-0-12-374814-0.00023-9 - 11.
Suurväli J, Whiteley AR, Zheng Y, Gharbi K, Leptin M, Wiehe T. The laboratory domestication of zebrafish: From diverse populations to inbred substrains. Molecular Biology and Evolution. 2019; 37 (4):1056-1069. DOI: 10.5061/dryad.1rn8pk0pz - 12.
Mrakovcic M, Haley LE. Inbreeding depression in the zebra fish Brachydanio rerio (Hamilton Buchanan). Journal of Fish Biology. 1979; 15 :323-327. DOI: 10.1111/j.1095-8649.1979.tb03612.x - 13.
Monson CA, Sadler KC. Inbreeding depression and outbreeding depression are evident in wild-type zebrafish lines. Zebrafish. 2010; 7 (2):189-197. DOI: 10.1089/zeb.2009.0648 - 14.
Harris MP, Henke K, Hawkins MB, Witten PE. Fish is fish: The use of experimental model species to reveal causes of skeletal diversity in evolution and disease. Journal of Applied Ichthyology. 2014; 30 :616-629. DOI: 10.1111/jai.12533 - 15.
Edmands S. Between a rock and a hard place: Evaluating the relative risks of inbreeding and outbreeding for conservation and management. Molecular Ecology. 2007; 16 :463-475. DOI: 10.1111/j.1365-294X.2006.03148.x - 16.
Songer M. Wildlife ecology. In: Encyclopedia of Ecology. 2nd ed. Vol. 1-4. Oxford, UK: Elsevier; 2019. pp. 509-516. DOI: 10.1016/B978-0-12-409548-9.11177-7 - 17.
Avdesh A, Chen M, Martin-Iverson MT, Mondal A, Ong D, Rainey-Smith S, et al. Regular care and maintenance of a zebrafish (Danio rerio) laboratory: An introduction. Journal of Visualized Experiments. 2012; 69 :4196. DOI: 10.3791/4196 - 18.
Westerfield M. A Guide for the Laboratory Use of Zebrafish Danio (Brachydanio) Rerio. 4th ed. Eugene: Univ. of Oregon Press; 2000 - 19.
Tsang B, Zahid H, Ansari R, Lee RCY, Partap A, Gerlai R. Breeding zebrafish: A review of different methods and a discussion on standardization. Zebrafish. 2017; 14 :561-573. DOI: 10.1089/zeb.2017.1477 - 20.
Spence R, Ashton R, Smith C. Oviposition decisions are mediated by spawning site quality in wild and domesticated zebrafish, Danio rerio. Behaviour. 2007; 144 :953-966 - 21.
Waples RS. What is Ne, anyway? Journal of Heredity. 2022; 113 :371-379. DOI: 10.1093/jhered/esac023 - 22.
Wang J, Santiago E, Caballero A. Prediction and estimation of effective population size. Heredity (Edinb). 2016; 117 :193-206. DOI: 10.1038/hdy.2016.43 - 23.
Castranova D, Wang C. Zebrafish breeding and colony management. In: The Zebrafish in Biomedical Research: Biology, Husbandry, Diseases, and Research Applications. London, UK: Academic Press; 2019. pp. 357-364. DOI: 10.1016/B978-0-12-812431-4.00031-2 - 24.
Abrams EW, Mullins MC. Early zebrafish development: It’s in the maternal genes. Current Opinion in Genetics & Development. 2009; 19 :396-403. DOI: 10.1016/j.gde.2009.06.002 - 25.
Ord J, Heath PR, Fazeli A, Watt PJ. Paternal effects in a wild-type zebrafish implicate a role of sperm-derived small RNAs. Molecular Ecology. 2020; 29 :2722-2735. DOI: 10.1111/mec.15505 - 26.
Felker A, Mosimann C. Contemporary zebrafish transgenesis with Tol2 and application for Cre/lox recombination experiments. Methods in Cell Biology. 2016; 135 :219-244. DOI: 10.1016/bs.mcb.2016.01.009 - 27.
Ghosh A, Halpern ME. Transcriptional regulation using the Q system in transgenic zebrafish. Methods in Cell Biology. 2016; 135 :205-218. DOI: 10.1016/bs.mcb.2016.05.001 - 28.
Asakawa K, Kawakami K. Targeted gene expression by the Gal4-UAS system in zebrafish. Development, Growth & Differentiation. 2008; 50 :391-399. DOI: 10.1111/j.1440-169X.2008.01044.x - 29.
Davidson A. Efficient gene delivery and gene expression in zebrafish using the sleeping beauty transposon. Developmental Biology. 2003; 263 (2):191-202. DOI: 10.1016/s0012-1606(03)00439-1 - 30.
Huang CJ, Tu CT, Der HC, Hsieh FJ, Tsai HJ. Germ-line transmission of a myocardium-specific GFP transgene reveals critical regulatory elements in the cardiac myosin light chain 2 promoter of zebrafish. Developmental Dynamics. 2003; 228 :30-40. DOI: 10.1002/dvdy.10356 - 31.
Tilley CA, Carreño Gutierrez H, Sebire M, Obasaju O, Reichmann F, Katsiadaki I, et al. Skin swabbing is a refined technique to collect DNA from model fish species. Scientific Reports. 2020; 10 :18212. DOI: 10.1038/s41598-020-75304-1 - 32.
Venditti M, Pedalino C, Rosello M, Fasano G, Serafini M, Revenu C, et al. A minimally invasive fin scratching protocol for fast genotyping and early selection of zebrafish embryos. Scientific Reports. 2022; 12 :22597. DOI: 10.1038/s41598-022-26822-7 - 33.
Xing L, Quist TS, Stevenson TJ, Dahlem TJ, Bonkowsky JL. Rapid and efficient zebrafish genotyping using PCR with high-resolution melt analysis. Journal of Visualized Experiments. 2014; 84 :e51138. DOI: 10.3791/51138 - 34.
Lambert CJ, Freshner BC, Chung A, Stevenson TJ, Bowles DM, Samuel R, et al. An automated system for rapid cellular extraction from live zebrafish embryos and larvae: Development and application to genotyping. PLoS One. 2018; 13 :e0193180. DOI: 10.1371/journal.pone.0193180 - 35.
Carmichael C, Westerfield M, Varga ZM. Cryopreservation and in vitro fertilization at the zebrafish international resource center. Methods in Molecular Biology. 2009; 546 :45-65. DOI: 10.1007/978-1-60327-977-2_4 - 36.
Hagedorn M, Carter VL. Zebrafish reproduction: Revisiting in vitro fertilization to increase sperm cryopreservation success. PLoS One. 2011;6 :e21059. DOI: 10.1371/journal.pone.0021059 - 37.
Matthews JL, Murphy JM, Carmichael C, Yang H, Tiersch T, Westerfield M, et al. Changes to extender, cryoprotective medium, and in vitro fertilization improve zebrafish sperm cryopreservation. Zebrafish. 2018;15 :279-290. DOI: 10.1089/zeb.2017.1521 - 38.
Diogo P, Martins G, Eufrásio A, Silva T, Cabrita E, Gavaia P. Selection criteria of zebrafish male donors for sperm cryopreservation. Zebrafish. 2019; 16 :189-196. DOI: 10.1089/zeb.2018.1660 - 39.
Khosla K, Kangas J, Liu Y, Zhan L, Daly J, Hagedorn M, et al. Cryopreservation and laser nanowarming of zebrafish embryos followed by hatching and spawning. Advanced Biosystems. 2020; 4 (11):e2000138. DOI: 10.1002/adbi.202000138 - 40.
Martins S, Monteiro JF, Vito M, Weintraub D, Almeida J, Certal AC. Toward an integrated zebrafish health management program supporting cancer and neuroscience research. Zebrafish. 2016; 13 :S47-S55. DOI: 10.1089/zeb.2015.1198 - 41.
Gutierrez AC, Gutierrez MC, Rhyner AM, Ruiz OE, Eisenhoffer GT, Wythe JD. FishNET: An automated relational database for zebrafish colony management. PLoS Biology. 2019; 17 (6):e3000343. DOI: 10.1371/journal.pbio.3000343 - 42.
Hensley MR, Hassenplug E, McPhail R, Leung YF. ZeBase: An open-source relational database for zebrafish laboratories. Zebrafish. 2012; 9 :44-49. DOI: 10.1089/zeb.2011.0730 - 43.
Yakulov TA, Walz G. Zebrafish database: Customizable, free, and open-source solution for facility management. Zebrafish. 2015; 12 :462-469. DOI: 10.1089/zeb.2015.1122 - 44.
Oltova J, Jindrich J, Skuta C, Svoboda O, Machonova O, Bartunek P. Zebrabase: An intuitive tracking solution for aquatic model organisms. Zebrafish. 2018; 15 :642-647. DOI: 10.1089/zeb.2018.1609 - 45.
Lacoursiere CM, Ullmann JFP, Poduri A. An open-source husbandry repository. Zebrafish. 2018; 15 :656-658. DOI: 10.1089/zeb.2018.1583