Abstract
Accumulation of polymeric solid wastes is a matter of distress and concern in the modern era. Plastic wastes are easier to recycle than rubbers, especially composite products like tires are very difficult to recycle. Finding sustainable end-of-life solutions for waste rubber products is crucial at present. The use of ground rubber, popularly known as crumb rubber obtained from the shredding of waste rubber goods in the construction of road pavements is one of the best possible ways to recycle rubber. About a billion end-of-life (ELT) tires are globally discarded every year, yet the use of crumb rubber generated from these tires in road construction is still negligible. The addition of crumb rubber not only permits the consumption of a significant amount of waste rubber but also provides numerous benefits to asphalt road pavement. Crumb rubber improves the rutting resistance, fatigue cracking and durability of roads. Rubberized asphalt roads also exhibit reduced traffic noise and increased driving safety. Though there are some limitations of crumb rubber as an additive, such as phase separation and poor workability, recent technical advancements are finding ways to overcome them. Further development of this technology is crucial for the establishment of a sustainable and circular economy.
Keywords
- crumb rubber
- rubber modified asphalt
- durability
- workability
- rheological properties
- dynamic shear modulus
1. Introduction
Construction and maintenance of roadways in modern civilization are the two most imperative civil engineering tasks to keep the world running. Owing to the proliferating number of passenger cars, public transport and heavy-duty vehicles, road pavements are subjected to massive and quick damages. Frequent maintenance is also often uneconomical and impractical. Solutions to these issues have been explored in the past decades, mainly focusing on the modification of the conventional bituminous binder which is used with asphalt for the construction of roads. The use of polymeric modifies, especially various rubbers has been beneficial in improving the durability, flexibility and resistance to permanent deformation. The first known experimental use of natural rubber in bitumen took place almost two centuries ago, in the 1840s. The first use of synthetic rubber modifier in bitumen was reported in 1923 and the first use of waste-tire-derived rubber to modify bitumen and its consequent application in asphalt roads was reported in 1944 [1].
Nevertheless, the advancement of human civilization has started to suffocate the earth with enormous amounts of industrial, construction and consumer wastes being dumped daily. A significantly large amount of the total waste constitutes polymeric materials, either plastic or rubber. Some plastics are somewhat recyclable as they are thermoplastics. Thermoplastics can usually undergo a few cycles of melting and reshaping. Rubbers are, on the other hand, thermosets, i.e., they undergo an irreversible curing process when moulded into a specific shape. Most rubber products are cured with sulphur or other chemicals that cause the polymeric rubber molecules to form a 3-dimensional network that cannot be easily reversed [2]. Numerous moulded rubber products are used commercially and they are dumped as non-degradable solid wastes after the end of their service life. Tires are the most notable amongst the rubber products used in modern civilization. Good quality tires may last up to 5–10 years, yet, with the enormous number of passenger cars, public transport and industrial vehicles that run globally, the annual generation of waste tires is more than 1.5 billion. Scrap tires are often abandoned on the ground, becoming a sanctuary for vermin and insects leading to biohazards. Burning of scrap tires emits toxic gases into the environment. Chemicals and microplastic from tires and other rubber products also pollute the soil and water. The sustainable and hazardous solutions for scrap tires are pictorially displayed in Figure 1.
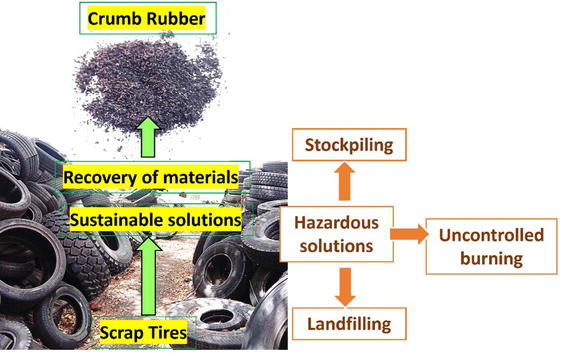
Figure 1.
The sustainable and hazardous solutions for scrap tires.
United Nations has recently set sustainable development goals with a focus on ‘responsible consumption and production’ and the 3R waste management system. Rubber and recycling industries are becoming more serious in their endeavour towards waste management. Industries are gradually developing a 4R waste management system that includes reducing, reusing, recycling and recovering. At present, 41% of end-of-life tires (ELT) still end up stockpiling and landfilling, and 42% of them are used for material recovery, 15% for energy recovery and only 2% for civil engineering [3]. Rubber recycling is possible in several techniques, such as reclamation and devulcanization, pyrolysis, downsizing or grinding. Grinding moulded rubber goods is an efficacious recycling technique that produces crumb rubber. Crumb rubber finds application in many subsidiary industries, most significantly in road pavement construction. The incorporation of crumb rubber has also remarkably improved the performance of asphalt in road pavements as shown in Figure 2 [4]. Apart from being a sustainable solution for end-of-life tires (ELT), the use of tire-derived crumb rubber significantly improves the durability, temperature resistance, fatigue cracking and rutting resistance of asphalt road pavements [5]. The practise of adding crumb rubber as well as other polymer wastes such as high and low-density polyethene has been extremely advantageous for use in road pavements for improved weathering and fatigue conditions [6].
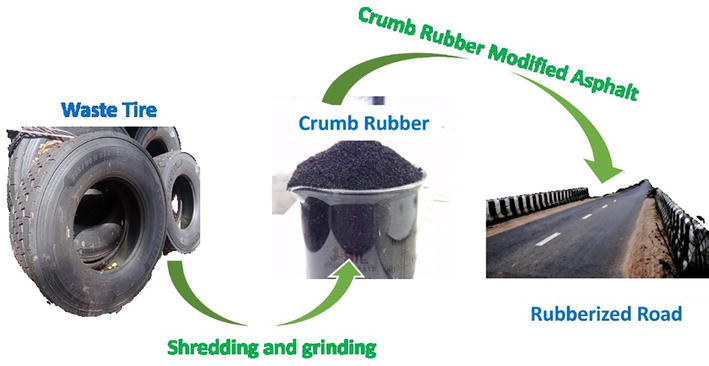
Figure 2.
Application of crumb rubber obtained from grinding of waste tires in rubberized roads.
Road construction is an important component of the modern city infrastructure. Ancient roadways were built with materials like clay, sandstone or gypsum. Since the beginning of the twentieth century, roads have generally been built with asphalt which is a composite of sand and bitumen. Bitumen is a mixed hydrocarbon residue obtained from the petroleum distillation process. This highly viscous liquid works as a binder for asphalt and protects from repetitive shock or load cycles. In modern days, concrete, a mixture of Portland cement, sand and water has become a popular road construction material. In asphalt or cement concrete, two types of aggregates, typically known as coarse aggregate and fine aggregate are mixed. Fine aggregate comprises sand, clay, fly ash and fine stones that pass through a 4.75 mm sieve but retain over a 0.075 mm sieve. Coarse aggregate is retained on a 4.75 mm sieve and they contain gravel, stone and brick chips, clinker, cinder etc. The idea of incorporating rubber in bitumen for road construction emerged way back in the nineteenth century soon after natural rubber was introduced as an industrial material. The actual implementation of this idea started in the middle of the twentieth century. Ground rubber from scrap tires was added to bitumen as a modifier in 1960 in Arizona, USA. The use of crumb rubber in road materials soon became a popular trend as it enhanced the properties of the roadways. The incorporation of rubber from scrap tires is usually carried out by dissolving crumb rubber in a bitumen binder. Another way of incorporating rubber in the construction of roadways is to partially replace the fine aggregate in the asphalt or cement composite with crumb rubber. The application of ground rubber in road construction is an important step towards sustainability and is also more eco-friendly than using waste rubber for landfilling. Ground rubber provides several advantages to the performance of the roadways such as:
Resistance towards rutting (longitudinal depression on the road surfaces), thermal cracking and other kinds of deformations like fatigue and reflective cracking
Resistance towards damages caused by rain and high temperature
Improvement in tensile properties, resistance from stress-cracking
Improvement in adhesion with the aggregates, increased viscosity of the binder preventing softening of the pavement surface
Enhanced road grip, skid and impact resistance leading to lower possibilities of accidents and related damages
Increased comfort during driving and reduced traffic noise
Enhanced durability of the road pavements
This chapter reports a comprehensive study on rubber-modified asphalts, their preparation, properties and recent technological advancements.
2. Preparation of crumb rubber
Crumb rubber is generally produced by shredding waste rubber products. Modern grinding techniques allow even large products such as whole tires to be converted to crumb rubber. Grinding of waste rubber product is usually done in three steps as follows:
Primary step: Whole tires or other moulded rubber goods are downsized in a cracker mill, hammer mill or rotary shear mill. This step produces coarse rubber pieces or chips through crushing and shredding processes. The steel wires and textile reinforcing elements are also removed from the rubber.
Secondary step: Rubber chips are further downsized in the second step where rubber granules are formed. High-load and high-speed granulators are used in this step. Rubber pieces are often brought to a brittle and glassy state by the application of liquid nitrogen to make the cutting process easier.
Tertiary step: Fine ground rubber is produced in the third step by wet grinding methods.
The surface area and properties of crumb rubber depend on the temperature maintained during the grinding process. Grinding at or above ambient temperature yields crumb rubber with a larger surface area. The particle size of this crumb rubber usually ranges between 0.5 and 5 mm. Ambient grinding is also cost-effective compared to low-temperature grinding. Low-temperature or cryogenic grinding is assisted by liquid nitrogen below −80°C. The particle size of this crumb rubber is around 50 mm [7].
3. Preparation of crumb rubber-modified asphalt (CRMA)
There are two common procedures to prepare crumb rubber modified asphalt (CRMA), the dry and the wet processes. A schematic diagram of the dry and wet processes is shown in Figure 3.
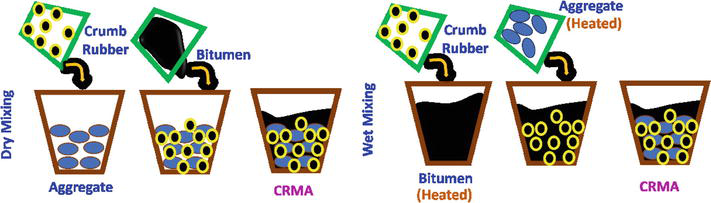
Figure 3.
Dry and wet mixing processes for CRMA.
3.1 Dry process
A portion of the fine aggregate, ideally 1–4% by weight is generally replaced with crumb rubber in this process [8]. The size of the crumb rubber particles mixed in the dry process is typically within the range of 0.4–9.5 mm. Dry mixing increases the stiffness of the mixture, making it less susceptible to stress-induced damage. Dry mixing reportedly improved the low-temperature cracking and high-temperature deformation properties of CRMA pavements [9]. The cohesion between the aggregate and asphalt is generally reduced in this dry mixing process as there is no significant interaction between the asphalt binder and crumb rubber. Crumb rubber is also said to increase the air void in the asphalt mixture in the dry process leading to damage by external factors like moisture.
However, plenty of research has been carried out to find the remedies to these issues. The use of certain reactive species to enhance the adhesion between the crumb rubber and asphalt binder has been a useful technique in the dry mixing process. An organo-silicon type nanomaterial with the commercial name of Zycosoil has been reported as an adhesion enhancer [10]. The aggregate was heated at 190°C before the addition of crumb rubber and mixed for 20 s. Varying doses of zycosoil were mixed with the asphalt binder which was preheated at 155°C. Homogeneous mixing of zycosoil was ensured by vigorous rotation of the mixture at 2500 rpm for half an hour. The softening point of the binder increased with the incorporation of the nanomaterial which improved its resistance towards high-temperature deformation. Zycosoil reportedly increased the surface free energy of adhesion between the binder and aggregate. A notable improvement in moisture resistance of the mixture was also observed as the hydrophilic aggregate surface was converted into a hydrophobic one owing to the formation of siloxane (Si-O-Si) bonds. This modification also resulted in the modulus, rutting and fatigue performance of the asphalt.
3.2 Wet process
In the wet process crumb rubber is mixed with asphalt preferably at elevated temperatures and the binder is added subsequently into the mix. Fine ground rubber, preferably within a size range of 0.075–1.2 mm is mixed with bitumen binder. Rubber particles swell in bitumen in heated conditions inducing physical changes in the bitumen. Lighter fractions of bitumen, mostly aromatic oils, are absorbed by rubber, and the binder’s viscosity increases notably. Crumb rubber modifies the binder in this process and hence this method yields significant improvement in the properties of road pavement compared to the dry process.
In a wet mixing process, the mixing sequence was studied thoroughly. The temperature of the binder was raised to 176°C when crumb rubber was added and 60 minute reaction time was given [11]. This sample was compared with a dry mixed sample. The high-temperature stability of the sample was better than the DM sample as inferred from the results of the Marshall stability test. High-temperature performance of was also superior owing to high dynamic modulus as low frequency. Conversely, the dry mix sample displayed better low-temperature performance because of its low modulus at high frequencies. The wet mix sample was also reported to display significantly higher rutting resistance in the same study.
3.3 Terminal blend asphalt rubber
Terminal blending (TB) is a modern technique that refers to a process where crumb rubber is digested by asphalt at elevated temperatures in a closed and controlled environment. Asphalt is generally heated to 200–300°C in a closed chamber where the crumb rubber is added and is subjected to high shear mixing, typically at 3000–8000 r/min speed for a sufficiently long period that enables complete and uniform blending between rubber and asphalt [12]. This procedure allows desulphurization and degradation of rubber to some extent that decreases the viscosity and enhances workability. The rheological properties of TB asphalt rubber were found to vary with the blending time as well as the temperature [13]. Longer time stimulates better dissolution of rubber and elevated temperature causes polymeric chain rupture of the rubber molecules resulting in reduced viscosity. Temperatures above 260°C cause severe chain breakage and the molecular weight of these polymer molecules is reduced to 3000–19,000 Daltons, comparable to the asphalt components. These degraded medium polymers are also known as apparent asphaltenes. The degradation of high polymers (molecular weight > 19,000 Daltons) to apparent asphaltenes aids in lowering viscosity but may deteriorate the hardness of the material over time. The stress relaxation properties of TB were found to be temperature-dependent. Shorter stress relaxation time indicates rapid dissipation of stress or high elasticity. Higher interaction temperature often results in longer relaxation time at low temperatures which is detrimental to temperature susceptibility at low-temperature ranges [14]. However, terminal blend in general provides improved workability and wide range applicability. Terminal blends consume a larger quantity of crumb rubber which has great environmental and economic benefits [15].
4. Effect of rubber on the chief properties of CRMA
The properties of rubberised bitumen depend on several variables, such as the properties and constituents of the bitumen, temperature and procedure of mixing, type of ground rubber etc. The common properties of asphalt and the effect of the addition of crumb rubber on these properties are discussed herein.
4.1 Durability
The durability of asphalt pavement indicated its resistance to ageing or age-hardening with time. The term ‘durability’ broadly describes a pavement’s resistance to all kinds of deformations with time. Modification of bitumen with crumb rubber generally enhances the time-dependent stability as the addition of rubber increases the pavement’s resilience and elasticity. Though rubberized asphalt roads may appear to be more energy-consuming due to the process of crumb rubber preparation and incorporation, rubberized roads require less frequent maintenance due to reduced wear [16]. Resistance to the damages caused by acoustic vibration is also remarkably improved in rubberized pavements [17]. Warm mix asphalt (WMA) is reported to be 50% more resistant to weathering effects. WM techniques reduced the asphalt mixing and compaction temperature by 20–40°C compared to the traditional techniques, making the process eco-friendly by reducing fuel use and emissions [18]. As the mechanical properties of the CRMA pavements are improved, a reduction in pavement thickness is possible with longer service life [19].
4.2 Workability and compaction
The workability of CRMA is the ease of mixing and compaction process of asphalt, with better workability indicating easier mixing. Workability is related to the volumetric properties as well as the pavement performance. Compaction is the process of reducing the volume of asphalt concrete during pavement construction. As the volume of the asphalt decreases, density increases and the air voids in the asphalt decrease. The incorporation of crumb rubber generally increases the compaction temperature as the air void volume increases with the addition of crumb rubber [20]. Some pieces of literature have criticised the role of crumb rubber in asphalt mixture as the absorption of the lightweight components of asphalt on the rubber surface may lead to reduced workability. However, it was also reported that if the content of crumb rubber was limited within a threshold level where the particle effect was negligible, the risk of poor workability could be avoided [21]. Controlling the viscosity of the binder at the mixing temperature is the key to achieving better workability. Road transport and highway regulations suggest that the viscosity of the modified asphalt binders should be within a limit of 300 mPa.s at 135°C. It was also observed that warm mixing techniques resulted in an improvement in the workability of CRMA. Additives like synthetic paraffin or zeolite have been reported to provide significant advantages in high-temperature performance and workability in the warm mix (WM) asphalts with low crumb rubber content [22]. The addition of the binder additives yielded low viscosity in 10–15% crumb rubber content. Another study also reported the use of commercial additives, such as Evotherm-DAT, Evotherm-3G, Sasobit etc. [23]. The Evotherm class binding additives improved workability by reducing the void content. These additives also reduced the construction temperature of CRMA by 16°C without any effect on compaction. A unique study reported the addition of natural rubber latex along with crumb rubber and organo-silane binder additive in CRMA which resulted in remarkable improvement in workability as well mechanical properties like resilience, fracture resistance, moisture resistance and reduced degrees of permanent deformation [24].
4.3 Storage stability
Crumb rubber modified asphalt (CRMA) faces the issue of phase separation of rubber from bitumen binder during the storage period. The difference between the solubility parameters of rubber and asphalt as well as the difference in density between cross-linked rubber and asphalt causes the separation or gravitational settling of rubber particles from the system. This occurrence is more frequent in the wet mixing process. It has also been observed that the larger particles have a faster rate of settling. On the other hand, more uniform compatibility and dispersion can be achieved by the use of smaller-sized rubber particles leading to superior storage stability [25]. Several attempts like the addition of crosslinking agents and modifiers have been made by researchers to increase the storage stability of CRMA. The use of styrene butadiene styrene copolymer as a modifier has been reported [26]. The formation of an internal 3D structure achieved by terminal blending at a specific time and temperature was also reported for improved storage stability [27].
4.4 Rheological properties
Rheological properties, mainly the viscosity and flow characteristics of CRMA are closely related to the workability and durability of asphalt pavement. Though very high content of crumb rubber resulting viscosity often leads to poor workability, the physical properties of asphalt binder are known to improve with the crumb rubber content. High viscosity gives rise to certain benefits such as low traffic noise, resistance to thermal damage and ageing, high rutting resistance, and longer lifetime. Rotational viscometer test is ideally used to determine the viscosity of CRMA Increased viscosity also resulted in improved penetration resistance and resistance to time-dependent deformations or creep [28]. The addition of crumb rubber to bitumen imparts a shear-thinning nature and increased elasticity. Epoxidized natural rubber (ENR) has been investigated to understand the effect of ageing on the rubberized asphalt’s rheological properties [29]. The ENR-modified binders exhibited increased hardness and penetration resistance up to a certain loading of rubber. Ageing resistance was also found to increase with the addition of crumb rubber up to a certain level of rubber loading. Ductility tests of the ENR-modified asphalts displayed an initial decrease followed by an increase in ductility with increasing rubber content. An increase in ductility also indicated improved low-temperature elasticity of the asphalt pavements. The addition of trans-polyoctenamer rubber (TOR) as an additive to CRMA was reported to further improve the rheological properties and storage stability [30]. Another study also reported the benefit of using a hot recycled asphalt mixture for achieving better rheological properties. The study suggested the use of 50% recycled asphalt with 50% CRMA for optimum rheological as well as economical interest [31].
4.5 Dynamic shear modulus: Rutting resistance and fatigue cracking
Dynamic shear modulus or the complex modulus of rubber-modified asphalt binder is another critical parameter that affects the performance of CRMA. The complex modulus (G*) is determined by the dynamic shear rheometer (DSR) test. Complex modulus (G*) crudely indicates the bitumen binder’s resistance towards deformation. The ratio of complex modulus and sin of the phase angle (G*/sinδ) is correlated with the rutting resistance and the parameter G*
5. Factors influencing the properties of CRMA
The properties of CRMA are influenced by several factors, such as the size of the crumb rubber particles, blending time and temperature, content of rubber etc. Smaller particle size produced at high-temperature or ambient grinding results in smaller particle size which is known to have an irregular surface and larger surface area as well as better interaction with the binder. Rubber particles with cubic or regular shapes and smooth surfaces offer a smaller area for interaction with the asphalt binder. Cryogenic grinding lowers the temperature of rubber below the glass transition temperature and thus the rubber undergoes brittle fracture and smooth surfaces are produced upon grinding. On the other hand, ambient grinding generates a spongy surface which is particularly beneficial for rubber-bitumen bonding. Smaller particles also result in a dense aggregate whereas larger rubber particles produce a coarse or gap-graded aggregate. A dry mixing process with coarse rubber particles incorporating gaps between the aggregate was expected to improve the skid resistance of pavement but ultimately displayed poor resistance to low-temperature cracking [42]. A study reported a comparative analysis of crumb rubber of particle sizes ranging from 20 to 120 mesh. The content of rubber was also varied as 10, 15 and 20% by weight of the total amount of CRM-asphalt. Change in particle size changes the degree of interaction between the crumb rubber and bitumen, though the relationship is nonlinear. The softening point of CRMA displayed an increasing trend up to 60 mesh particle size with 15 and 20% rubber content and decreased after further increase in particle size. Ductility was found to be increasing with the increase in rubber content. The viscosity of CRMA decreased when the blending time was varied from 0 to 200 minutes at temperatures 180, 200, and 225°C. The ductility of CRMA was prone to increase with blending or reaction time. The study conclusively reported that crumb rubber of 80 mesh particle size displayed the best combination of properties at 15% rubber content. As the viscosity significantly reduced at elevated temperature (200°C) with blending time longer than 60 minutes, these reaction conditions were found to be optimal for fair workability [43]. Another temperature-dependent study revealed that moderately elevated temperature imparts flexibility to CRMA resulting in improved rutting resistance. The optimum temperature for the absorption of crumb rubber particles on the light components of asphalt was reported to be 190°C. Further rise in the temperature to as high as 220°C initiates intense reactions, generates new functional groups and decreases the mechanical properties and heat-stability of CRMA. Hence, it is crucial to limit the blending temperature to 190–200°C [44].
6. Challenges associated with CRMA
Regardless of the number of benefits of CRMA mentioned in this review, there are a few challenges and gaps in the development of CRMA technology. The relationship between temperature with the viscosity and workability of rubber-modified bitumen is complicated. The workability is also often dependent on manual factors. The maximum working temperature varies with different climatic conditions in different countries, e.g., 190°C in Denmark and 195°C in China. A comprehensive survey involving global participants such as the USA, India, Japan, Denmark, Australia, Canada, China etc. conducted in 2010 failed to determine a unified standard of mixing and compaction temperature (MCT) and viscosity of CRMA. Hence, there is a lacuna in the common global standard for the application of CRMA. Moreover, the reaction between rubber-modified bitumen and asphalt may continue for an indefinite period after the blending process causing untraceable changes in the properties of CRMA. There are possibilities that CRMA may not be a classically homogeneous mixture at all even after thorough blending; in most cases, the rubber particles are only encapsulated with the binders [45]. Crumb rubber contains sulphur and other organic chemicals which produce harmful smoke at elevated temperatures that are detrimental to the environment as well as the workers’ health. Swelling of crumb rubber is also a common factor that increases the viscosity and reduces the workability [46]. Maintaining the storage stability of crumb rubber-modified bitumen is another practical challenge. CRMA produced by the dry process has also been reported to be susceptible to moisture damage [47].
7. Recent developments and future scope
Despite the few limitations associated with the application of CRMA, crumb rubber modification in asphalt and cement concrete appears to be indispensable for achieving sustainability in the construction sector. Recent scientific research on CRMA focuses on the limitations of its application like high viscosity, volatile content, high-temperature requirements and poor storage stability. Polyurethane was used as an additive to improve storage stability and workability and to reduce the volatile content from CRMA [42]. UiO-66 class of metal-organic complexes were reported to incredibly reduce the volatile organic contents (VOCs) resulting from the presence of unsaturated metal atoms and high surface area [43]. A blend of rubber and plastic (crumb rubber and polyethene) was reported to remarkably reduce the viscosity and improve the workability of CRMA [44]. Increasing interest is observed in the incorporation of nanomaterials like graphene and carbon nanotubes to enhance the properties of CRMA. The effect of the number of layers and size of graphene sheets on the properties of CRMA was thoroughly studied and graphene was also estimated to effectively improve high-temperature deformation and rheological behaviour [45].
The aspect of economic and environmental benefits of CRMA are in supreme focus for the future development of this technology. Refinement of the wet mixing and terminal blending methods and the use of novel additives may further reduce the viscosity and improve workability resulting in at least 20–25% fuel reserve. The emission of carbon monoxide and methane was remarkably low for CRMA pavement construction, but the emission of CO2, NOx, and SO2 is still a concern that needs to be addressed. However, a quantitative study of the energy benefits of the CRMA pavements in their service life and recycling is required at this stage. The recycling and rehabilitation process of the CRMA pavements also requires further structural improvement [48].
8. Conclusions
Crumb rubber modified asphalt (CRMA) is extensively used for road construction in parts of the USA, Western Europe, Australia and South Africa. Flexible CRMA pavements are extremely durable, recyclable and require low maintenance. Crumb rubber is also a popular additive in cement-based roadways at present. It is estimated that building a kilometre-long road of 6 cm thickness with 0.5% of the total weight of bitumen modified with crumb rubber could reuse a thousand scrap tires. Replacement of bitumen with crumb rubber also significantly reduces the carbon footprint. Hence, the use of crumb rubber in road construction as well as other possible civil engineering works is probably the most sustainable solution for end-of-life tires (ELTs). Numerous long-lasting benefits of crumb rubber modification have already been established through a few decades of application and extensive research. The most advantageous feature of the rubberized roads is the crumb rubber’s ability to resist fatigue cracking and plastic deformation resulting in outstanding durability and low maintenance. A 40–80% reduction in tire-pavement friction noise is also achievable by the use of CRMA. The recyclability of CRMA pavement and a significant reduction in carbon emission compared to conventional pavement construction are the two most eco-friendly features that add special value to this technology. There are disadvantages as well, such as low storage stability, rise in viscosity and phase separation of the CRMA-modified bitumen with time, difficult optimization of blending time and temperature etc., yet, the economic and environmental benefits of CRMA outweigh these process difficulties. Ample research is also going on to overcome these processing issues and the use of certain additives like paraffin wax, zeolite and co-polymers such as styrene-butadiene-styrene have been proven efficacious. Special attention is being given to the improvement of CRMA road durability in extreme and severe climate conditions as well. Further refining the CRMA technology and overcoming the few shortcomings, will be an important milestone towards sustainability. Application of CRMA and rubberized cement concrete in road construction as well as other civil engineering sectors ought to be popularised globally to establish a shared circular economy in rubber recycling and construction industries shortly.
References
- 1.
Mashaan NS, Ali AH, Karim MR, Abdelaziz M. An overview of crumb rubber modified asphalt. International Journal of Physical Sciences. 2012; 7 (2):166-170. DOI: 10.5897/IJPSX11.007 - 2.
Adhikari B, De D, Maiti S. Reclamation and recycling of waste rubber. Progress in Polymer Science. 1 Sep 2000; 25 (7):909-948 - 3.
Valentini F, Pegoretti A. End-of-life options of tyres. A review. Advanced Industrial and Engineering Polymer Research. 2022; 5 (4):203-213. DOI: 10.1016/j.aiepr.2022.08.006. KeAi Communications Co - 4.
Leong SY, Lee SY, Koh TY, Ang DTC. 4R of rubber waste management: Current and outlook. Journal of Material Cycles and Waste Management. 2023; 25 (1):37-51. DOI: 10.1007/s10163-022-01554-y. Springer - 5.
Myhre M, Saiwari S, Dierkes W, Noordermeer J. Rubber recycling: Chemistry, processing, and applications. Rubber Chemistry and Technology. 2012; 85 (3):408-449. DOI: 10.5254/rct.12.87973 - 6.
Khan IM, Kabir S, Alhussain MA, Almansoor FF. Asphalt design using recycled plastic and crumb-rubber waste for sustainable pavement construction. In: Procedia Engineering. 2016. pp. 1557-1564. DOI: 10.1016/j.proeng.2016.04.196 - 7.
Milad A, Ahmeda AGF, Taib AM, Rahmad S, Solla M, Yusoff NIM. A review of the feasibility of using crumb rubber derived from end-of-life tire as asphalt binder modifier. Journal of Rubber Research. 2020; 23 (3):203-216. DOI: 10.1007/s42464-020-00050-y - 8.
Airey GD, Rahman MM, Collop AC. Absorption of bitumen into crumb rubber using the basket drainage method. International Journal of Pavement Engineering. 2003; 4 (2):105-119. DOI: 10.1080/1029843032000158879 - 9.
Cao W. Study on properties of recycled tire rubber modified asphalt mixtures using dry process. Construction and Building Materials. 2007; 21 (5):1011-1015. DOI: 10.1016/j.conbuildmat.2006.02.004 - 10.
Arabani M, Tahami SA, Hamedi GH. Performance evaluation of dry process crumb rubber-modified asphalt mixtures with nanomaterial. Road Materials and Pavement Design. 2018; 19 (5):1241-1258. DOI: 10.1080/14680629.2017.1302356 - 11.
Yu H et al. Effect of mixing sequence on asphalt mixtures containing waste tire rubber and warm mix surfactants. Journal of Cleaner Production. 2020; 246 . DOI: 10.1016/j.jclepro.2019.119008 - 12.
Xie J, Zhang Y, Yang Y. Study on preparation method of terminal blend rubberized asphalt binder. Frontiers in Materials. 2020; 7 . DOI: 10.3389/fmats.2020.00279 - 13.
Huang W, Lin P, Tang N, Hu J, Xiao F. Effect of crumb rubber degradation on components distribution and rheological properties of terminal blend rubberized asphalt binder. Construction and Building Materials. 2017; 151 :897-906. DOI: 10.1016/j.conbuildmat.2017.03.229 - 14.
Li B et al. Evolution of components distribution and its effect on low temperature properties of terminal blend rubberized asphalt binder. Construction and Building Materials. 2017; 136 :598-608. DOI: 10.1016/j.conbuildmat.2017.01.118 - 15.
Han L, Zheng M, Wang C. Current status and development of terminal blend tyre rubber modified asphalt. Construction and Building Materials. 2016; 128 :399-409. DOI: 10.1016/j.conbuildmat.2016.10.080. Elsevier Ltd - 16.
Riekstins A, Haritonovs V, Straupe V. Economic and environmental analysis of crumb rubber modified asphalt. Construction and Building Materials. 2022; 335 . DOI: 10.1016/j.conbuildmat.2022.127468 - 17.
Licitra G, Cerchiai M, Teti L, Ascari E, Fredianelli L. Durability and variability of the acoustical performance of rubberized road surfaces. Applied Acoustics. 2015; 94 :20-28. DOI: 10.1016/j.apacoust.2015.02.001 - 18.
Puccini M, Leandri P, Tasca AL, Pistonesi L, Losa M. Improving the environmental sustainability of low noise pavements: Comparative life cycle assessment of reclaimed asphalt and crumb rubber based warm mix technologies. Coatings. 2019; 9 (5). DOI: 10.3390/coatings9050343 - 19.
Farina A, Zanetti MC, Santagata E, Blengini GA. Life cycle assessment applied to bituminous mixtures containing recycled materials: Crumb rubber and reclaimed asphalt pavement. Resources, Conservation and Recycling. 2017; 117 :204-212. DOI: 10.1016/j.resconrec.2016.10.015 - 20.
Çelik ON, Atiş CD. Compactibility of hot bituminous mixtures made with crumb rubber-modified binders. Construction and Building Materials. 2008; 22 (6):1143-1147. DOI: 10.1016/j.conbuildmat.2007.02.005 - 21.
Yu H, Deng G, Zhang Z, Zhu M, Gong M, Oeser M. Workability of rubberized asphalt from a perspective of particle effect. Transportation Research Part D: Transport and Environment. 2021; 91 . DOI: 10.1016/j.trd.2021.102712 - 22.
Wang H, Li X, Xiao J, You Z, Yang X, Irfan M. High-temperature performance and workability of crumb rubber-modified warm-mix asphalt. Journal of Testing and Evaluation. 2018; 48 (4). DOI: 10.1520/JTE20170384 - 23.
Yu H, Leng Z, Dong Z, Tan Z, Guo F, Yan J. Workability and mechanical property characterization of asphalt rubber mixtures modified with various warm mix asphalt additives. Construction and Building Materials. 2018; 175 :392-401. DOI: 10.1016/j.conbuildmat.2018.04.218 - 24.
Poovaneshvaran S, Zheng LW, Hasan MRM, Yang X, Diab A. Workability, compactibility and engineering properties of rubber-modified asphalt mixtures prepared via wet process. International Journal of Pavement Research and Technology. 2021; 14 (5):560-569. DOI: 10.1007/s42947-020-1006-z - 25.
Liang M, Xin X, Fan W, Sun H, Yao Y, Xing B. Viscous properties, storage stability and their relationships with microstructure of tire scrap rubber modified asphalt. Construction and Building Materials. 2015; 74 :124-131. DOI: 10.1016/j.conbuildmat.2014.10.015 - 26.
Cheng G, Shen B, Zhang J. A study on the performance and storage stability of crumb rubber-modified asphalts. Petroleum Science and Technology. 2011; 29 (2):192-200. DOI: 10.1080/10916460903070421 - 27.
Ragab M, Abdelrahman M. Enhancing the crumb rubber modified asphalt’s storage stability through the control of its internal network structure. International Journal of Pavement Research and Technology. 2018; 11 (1):13-27. DOI: 10.1016/j.ijprt.2017.08.003 - 28.
Kim HS, Lee SJ, Amirkhanian S. Rheology investigation of crumb rubber modified asphalt binders. KSCE Journal of Civil Engineering. 2010; 14 (6):839-843. DOI: 10.1007/s12205-010-1020-9 - 29.
Al-Mansob RA, Ismail A, Yusoff NIM, Albrka SI, Azhari CH, Karim MR. Rheological characteristics of unaged and aged epoxidised natural rubber modified asphalt. Construction and Building Materials. 2016; 102 :190-199. DOI: 10.1016/j.conbuildmat.2015.10.133 - 30.
Liu H, Chen Z, Wang W, Wang H, Hao P. Investigation of the rheological modification mechanism of crumb rubber modified asphalt (CRMA) containing TOR additive. Construction and Building Materials. 2014; 67 (Part B):225-233. DOI: 10.1016/j.conbuildmat.2013.11.031 - 31.
Chen T, Ma T, Huang X, Guan Y, Zhang Z, Tang F. The performance of hot-recycling asphalt binder containing crumb rubber modified asphalt based on physiochemical and rheological measurements. Construction and Building Materials. 2019; 226 :83-93. DOI: 10.1016/j.conbuildmat.2019.07.253 - 32.
Palit SK, Reddy KS, Pandey BB. Laboratory evaluation of crumb rubber modified asphalt mixes. Journal of Materials in Civil Engineering. Feb 2004; 16 (1):45-53 - 33.
Petersen JC, Robertson RE, Branthaver JF, Harnsberger PM, Duvall JJ, Kim SS, et al. Binder characterization and evaluation: Volume 1. Rep. No. SHRP-A-367, Strategic Highway Research Program. Washington, DC: National Research Council; May 1994 - 34.
Wang L, Xing Y, Chang C. Microscopic and dynamic rheological characteristics of crumb rubber modified asphalt. Journal Wuhan University of Technology, Materials Science Edition. 2010; 25 (6):1022-1026. DOI: 10.1007/s11595-010-0142-8 - 35.
Navarro FJ, Partal P, Martínez-Boza F, Gallegos C. Influence of crumb rubber concentration on the rheological behavior of a crumb rubber modified bitumen. Energy and Fuels. 2005; 19 (5):1984-1990. DOI: 10.1021/ef049699a - 36.
Poovaneshvaran S, Mohd Hasan MR, Putra Jaya R. Impacts of recycled crumb rubber powder and natural rubber latex on the modified asphalt rheological behaviour, bonding, and resistance to shear. Construction and Building Materials. 2020; 234 . DOI: 10.1016/j.conbuildmat.2019.117357 - 37.
Ameri M, Afshin A, Ebrahimzadeh Shiraz M, Yazdipanah F. Effect of wax-based warm mix additives on fatigue and rutting performance of crumb rubber modified asphalt. Construction and Building Materials. 2020; 262 . DOI: 10.1016/j.conbuildmat.2020.120882 - 38.
Kataware AV, Singh D. Dynamic mechanical analysis of crumb rubber modified asphalt binder containing warm mix additives. International Journal of Pavement Engineering. 2019; 20 (9):1044-1054. DOI: 10.1080/10298436.2017.1380806 - 39.
Ming LY, Feng CP, Siddig EAA. Effect of phenolic resin on the performance of the styrene-butadiene rubber modified asphalt. Construction and Building Materials. 2018; 181 :465-473. DOI: 10.1016/j.conbuildmat.2018.06.076 - 40.
Kumar SA, Veeraragavan A. Dynamic mechanical characterization of asphalt concrete mixes with modified asphalt binders. Materials Science and Engineering A. 2011; 528 (21):6445-6454. DOI: 10.1016/j.msea.2011.05.008 - 41.
Li Y et al. Anti-rutting performance evaluation of modified asphalt binders: A review. Journal of Traffic and Transportation Engineering (English Edition). 2021; 8 (3):339-355. DOI: 10.1016/j.jtte.2021.02.002. Chang’an University - 42.
Hassan NA, Airey GD, Jaya RP, Mashros N, Aziz MA. A review of crumb rubber modification in dry mixed rubberised asphalt mixtures. Jurnal Teknologi. 2014; 70 (4):127-134. DOI: 10.11113/jt.v70.3501 - 43.
Cao WD, Liu ST, Cui XZ, Yu XQ. Effect of crumb rubber particle size and content on properties of crumb rubber modified (CRM) asphalt. Applied Mechanics and Materials. 15 Oct 2011; 99 :955-959 - 44.
Yang X, Shen A, Guo Y, Lyu Z. Effect of process parameters on the high temperature performance and reaction mechanism of CRMA. Petroleum Science and Technology. 2018; 36 (19):1537-1543. DOI: 10.1080/10916466.2018.1482315 - 45.
Mohammadi I, Khabbaz H. Challenges associated with optimisation of blending, mixing and compaction temperature for asphalt mixture modified with crumb rubber modifier (CRM). Applied Mechanics and Materials. 15 Feb 2013; 256 :1837-1844 - 46.
Zheng W, Wang H, Chen Y, Ji J, You Z, Zhang Y. A review on compatibility between crumb rubber and asphalt binder. Construction and Building Materials. 2021; 297 . DOI: 10.1016/j.conbuildmat.2021.123820. Elsevier Ltd - 47.
Ghabchi R, Arshadi A, Zaman M, March F. Technical challenges of utilizing ground tire rubber in asphalt pavements in the United States. Materials. 2021; 14 (16). DOI: 10.3390/ma14164482 - 48.
Wang T, Xiao F, Zhu X, Huang B, Wang J, Amirkhanian S. Energy consumption and environmental impact of rubberized asphalt pavement. Journal of Cleaner Production. 2018; 180 :139-158. DOI: 10.1016/j.jclepro.2018.01.086. Elsevier Ltd