Tafel parameters for a 316L SS electrode uncovered and covered with the different coatings.
Abstract
Interest in cerium-based coatings has increased in recent years due to their low toxicity, biocompatibility, and improved corrosion protection performance. The formation of a coating from a solution containing cerium nitrate (Ce(NO3)3) has been investigated as a good surface modification strategy for the production of protective coatings on 316L SS. The effect of various additives in the treatment solution (sodium molybdate, sodium salicylate, and ascorbic acid) on the corrosion protection properties of the coatings was evaluated. The protection performance of the coated samples in a physiological simulated fluid (Ringer solution) was examined by electrochemical methods. The composition and morphology of all coatings were analyzed by scanning electron microscopy (SEM) and energy dispersive spectroscopy (EDX).
Keywords
- 316L stainless steel
- corrosion protection
- cerium
- molybdate
- salicylate
- ascorbic acid
1. Introduction
In recent years, 316L stainless steel (316L SS) has been widely used for different applications in various fields because of its good corrosion resistance. Its biocompatibility, high corrosion resistance, and mechanical properties make it an excellent alternative for biomedical applications [1, 2]. Nevertheless, this material suffers pitting corrosion in aqueous solutions, which limits its potential applications [1, 2].
The release of unwanted ions in the surroundings of the 316L SS implant causes problems that are usually difficult to treat, leading to an early implant failure and inducing a risk for the living tissues [3, 4]. In addition, stainless steel is susceptible to bacterial growth under physiological conditions, which is another severe cause of implant failure [5]. In order to avoid this type of degradation, the use of protective coatings is a promising alternative. Several electrochemical methods were employed for the formation of protective coatings. Among these, the conversion treatment is distinguish for its cost-effective use and easy operation [3]. These coatings act as a barrier between the substrate and the corrosive environment [4].
In order to enhance the corrosion resistance of stainless steel in chloride containing media, cerium-based coatings have been investigated [6]. Cerium oxides have been extensively studied for biomedical applications due to their low toxicity, corrosion inhibition, and self-healing properties [7, 8]. The improvement in the corrosion resistance is attributed to the role of Ce+4 and Ce+3 ions within the film, which have the ability to supply oxygen and promote the formation of the passive layer on the SS surface. In addition, self-healing ability of cerium films is activated when the local pH is sufficiently high [9]. Cerium oxides and hydroxides are deposited on the substrate surface as a result of local pH increase, thereby preventing the spread of corrosion [9].
Salicylates have been reported to have important biological and pharmacological beneficial properties for human health. They are one of the oldest and most widely used anti-inflammatory drugs and also exhibit various recognized pharmacological roles such as anticancer, neuroprotective, antipyretic, skin antiaging, and antidiabetic effects [10]. It was reported that the presence of sodium salicylate in a polypyrrole coating improves the corrosion protection of 316L SS, promoting the formation of the passive layer on the surface of substrate [11, 12]. Moreover, it has been informed a favorable
On the other hand, molybdate anion has many advantages, such as low toxicity and high stability in aqueous media [14, 15]. Particularly, molybdate was also used extensively as a corrosion inhibitor of iron and stainless steels over a wide-ranging temperature and pH [16, 17]. The anion positively affects the passivity breakdown and pitting initiation of 316L SS. Moreover, it has been reported that the use of molybdate as dopant in the polypyrrole electrodeposition on 316L SS results in the formation of coatings with good anticorrosive properties [18]. The improvement in the corrosion resistance is associated with the presence of molybdenum species in the obtained film.
Ascorbic acid (HAsc) has been extensively studied for steel in acid and neutral media due to its inhibitor character [19, 20, 21]. The formation of an oxide film with protective properties on steel is attributed to the deposition of insoluble chelates on the substrate surface that occurs at an optimal concentration of 10−3 M [21]. Nevertheless, the authors also reported that concentrations higher than 5 × 10−3 M lead to the formation of soluble chelates that promotes Fe dissolution. In addition, the HAsc showed a dual behavior. Depending on the conditions, it can behave as a corrosion inhibitor or it can promote the corrosion of stainless-steel (SS)X4Cr13 in HCl solutions [22].
The presence of cerium-based coatings on steel surfaces has been previously demonstrated as a major advance in the engineering field. Recently, Hayajneh et al. have presented the results of improving the corrosion behavior of 316L SS by developing a thin cerium oxide gelatin nanolaminate coating. The results confirmed the formation of homogeneous and crack-free coatings without any apparent defects. The prepared films significantly improved the corrosion resistance of 316L SS in a simulated marine environment [6]. Pitting corrosion of 304 SS in an alkaline 3.5% NaCl solution was significantly improved by a chemical cerium conversion coating. The results showed that both the Ecorr and Epit values of 304 SS could be greatly increased by the coating [23]. In addition, some recent work related to biomedical engineering can be mentioned. Abdulaah et al. applied a cerium tricalcium phosphate (CTP) coating on the surface of 316L SS using the high-frequency sputtering technique. They investigated the corrosion protection performance in a simulated body fluid, and the experimental results showed that the CTP coating improved the corrosion resistance in a simulated body fluid with a protective efficiency of 91% [24].
Critical issues in implant surgery are bacterial infections. In order to prevent infections, the implant surfaces could be an effective alternative to avoid the adhesion and growth of bacteria [25]. Hence, the development of coatings with antibacterial activity has recently attracted significant attention. Silver nanoparticles (AgNps) are well-known as a wide-spectrum antibacterial agents. Stable and long-term antibacterial activity shows the AgNps without affected by drug resistance [26]. The antibacterial mechanism of Ag is not fully understood; however, it is known that silver ions are the ones that interact with bacteria cell walls, resulting in bacterial death [27].
The aim of this study is the formation of a biocompatible, anticorrosive, and antibacterial film on 316L SS in order to functionalize the substrate for stent applications. Thus, the coatings were formed in a solution containing cerium nitrate and hydrogen peroxide on 316L SS by a potentiostatic technique. The influence of the presence of additives as sodium salicylate, sodium molybdate, and ascorbic acid in the treatment solution and employed technique on the properties of the coatings was evaluated. Films were characterized using electrochemical techniques and SEM/EDX. In order to compare, the corrosive behavior was also evaluated for the different films and the bare 316L SS.
2. Corrosion inhibition study of 316L SS in presence of additives
The working electrodes used for
To increase the anticorrosive performance of the cerium-based film formed in combination with H2O2, the addition of different compounds was studied. The procedure used to evaluate the effect of the additives in the cerium treatment was similar to that reported by us for AZ91D Mg alloy [28].
Thereby, the inhibition effect of sodium salicylate (NaSa), sodium molybdate (Na2MoO4), and ascorbic acid (HAsc) on the electrochemical behavior of bare 316L SS in Ringer solution was evaluated. In order to establish the most favorable conditions for cerium-based coating generation, different concentrations of each additive were analyzed. Polarization curves of 316L SS in Ringer solutions containing different additive concentrations are presented in Figures 1–3. It can be observed that the corrosion rate of 316L SS is delayed by the presence of the NaSa for all concentrations evaluated (Figure 1). The best inhibition effect was obtained for 5 mM NaSa (Figure 1, curve c). In order to analyze the effect of presence of Na2MoO4 and ascorbic acid (HAsc), a similar procedure was carried out (Figures 2 and 3, respectively). The highest corrosion inhibition effect of Na2MoO4 was obtained for 15 mM (Figure 2, curve d), while for HAsc, the optimal concentration was 1 mM (Figure 3, curve b).
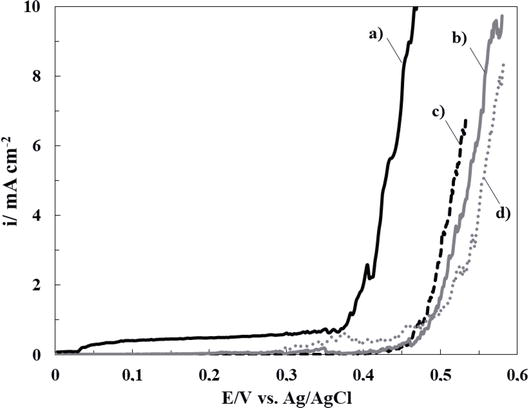
Figure 1.
Polarization curves obtained at scan rate of 0.001 Vs−1 for bare 316L SS alloy in ringer solution containing different NaSa concentrations: (a) 0 mM, (b) 1 mM, (c) 5 mM, and (d) 15 mM.
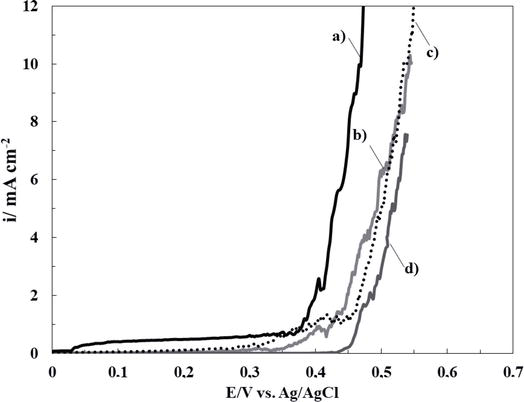
Figure 2.
Polarization curves obtained at scan rate of 0.001 Vs−1 for bare 316L SS alloy in ringer solution containing different Na2MoO4 concentrations: (a) 0 mM, (b) 1 mM, (c) 5 mM, and (d) 15 mM.
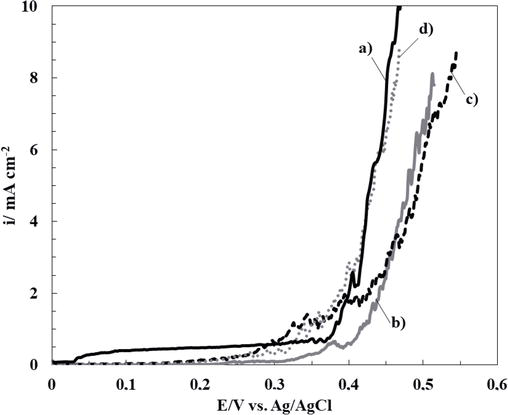
Figure 3.
Polarization curves obtained at scan rate of 0.001 Vs−1 for bare 316L SS alloy in ringer solution containing different HAsc concentrations: (a) 0 mM, (b) 1 mM, (c) 5 mM, and (d) 15 mM.
It was reported that the NaSa is predominantly an anodic inhibitor [29]. In the case of molybdate was also used extensively as a corrosion inhibitor of iron and stainless steels over a wide-ranging temperature and pH range [11, 13]. The anion positively affects the passivity breakdown and pitting initiation of 316L SS.
It has been published that in acid and neutral media, the deposition of an insoluble chelate occurs at an optimal concentration of 10−3 M HAsc, allowing the formation of a protective oxide film on the steel surface [19, 20, 21]. According to our results, Fe dissolution is promoted at concentrations above 5 × 10−3 M. In addition, several works reported a dual role of HAsc; it can increase the dissolution rate of substrates or act as a corrosion inhibitor depending on the circumstances [22, 30].
As previously reported, the improved inhibition performance of RCeNaSa is due to a synergistic effect of this compound in which both components, which are inhibitors of 316L SS by themselves, are simultaneously incorporated into a surface coating, as shown by the above results. This is because the organic component (NaSa) is predominantly an anodic inhibitor [29], while the cerium (under these circumstances) proves to be predominantly a cathodic inhibitor [31].
For all the coatings studied, optimal conversion parameters such as additive concentrations, applied potential, and temperature were determined in order to obtain protective cerium coatings on 316L SS. Thus, the corrosion efficiency was evaluated for the electrodes treated in different cerium-based solutions by the potentiodynamic polarization tests in Ringer solution at 37°C. Firstly, cerium-based coating was formed onto the 316L SS. More protective film was electrosynthetized from an electrolyte solution containing 30 mM Ce (NO3)3·6H2O and 25 mM H2O2 in a purified nitrogen gas saturated atmosphere. The applied potential applied was of - 0.7 V, and the temperature employed was 50°C. For simplicity purposes, this coating will be called RCe. The presence of hydrogen peroxide favors the oxidation of Ce3+ to Ce4+, incorporating the cerium (IV) species in the film [32]. It has been reported that the presence of cerium (IV) results in a yellow-colored film [33], whereas the presence of cerium (III) is responsible for the white color [28, 30]. This film obtained will be identified in the text as RCe.
The optimal concentration of each additive for coating formation was established from the corrosion inhibition results shown above (Figures 1–3). Thus, the cerium-based coatings were formed in a solution containing 30 mM Ce(NO3)3, 25 mM H2O2, and:
5 mM NaSa at - 0.70. This film will be identified as RCeNaSa.
15 mM Na2MoO4 at - 1.00 V. This film will be called RCeMo.
1 mM HAsc at - 0.80 V. This film will be called RCeHAsc.
After 30 min of the conversion treatment at 50°C, a golden yellow-colored was observed with the naked eyes when the substrate for all coatings was obtained.
3. Morphological and compositional characterization of cerium-based coatings
Figures 4–6 show the SEM micrographs of RCeNaSa, RCeMo, and RCeHAsc films, respectively. For all coatings, the electrode surface is characterized by cracked-mud structure. A similar morphology was reported for cerium-based coatings [34]. The cracked morphology is associated with different causes: the generation of gas bubbles on the electrode surface during the coating formation, the dehydration process during coating drying, or the shearing stresses between the substrate surface and the coating [35].
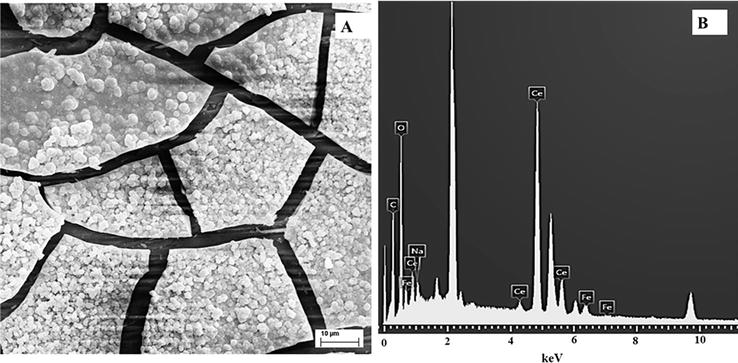
Figure 4.
SEM image (A) and EDX examination (B) of the 316L SS covered with RCeNaSa coating.
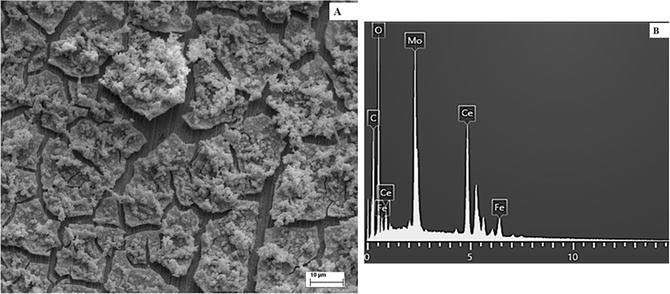
Figure 5.
SEM image (A) and EDX examination (B) of the 316L SS covered with RCeMo coating.
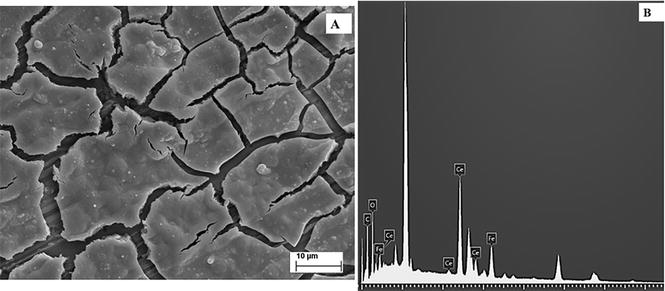
Figure 6.
SEM image (A) and EDX examination (B) of the 316L SS covered with RCeHAs coating.
The presence of Ce and Fe in the coatings was confirmed by EDX analysis. Also, the signal of additives incorporated in the coating as Mo and Na was distinguished in the EDX examination.
4. Evaluation of anticorrosive performance of the cerium-based films
Various experiments were performed to test the corrosion performance of the coatings. The OCP change with time was measured over 4 days (Figure 7). At first, the OCP value for bare steel sample stabilizes after the second day of immersion at a value around - 0.15 V. The samples covered with the different coatings show slightly more positive values than the uncoated substrate, suggesting a protective effect of the coatings. These preliminary results show that all coatings improve the corrosion protection of the bare steel.
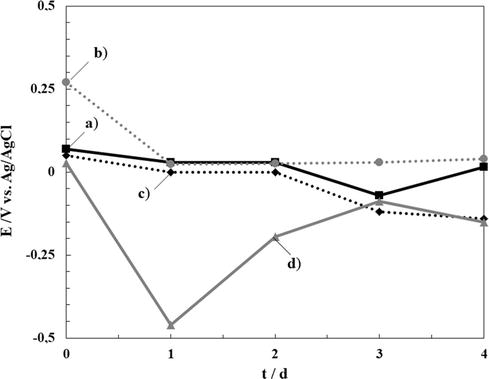
Figure 7.
Time dependence of OCP in ringer solution of 316L SS electrode covered by: (a) RCeNaSa coating, (b) RCeMo coating, and (c) RCeHAsc. The variation of bare steel electrode is also included (d).
The improved corrosion resistance can also be evaluated by comparing the polarization curves in Ringer solution (Figure 8). The potentiodynamic curve for bare 316L SS (curve d) shows a passive region followed by an increase in current density at 0.35 V versus Ag/AgCl, indicating the breakdown of passivity and the onset of pitting corrosion [36]. In the case of the substrate coated with RCeNaSa and RCeHAsc, no change in the pitting corrosion potential is observed due to the presence of films. This result can be explained by the open structure of the formed coatings observed by SEM microscopy, which allows aggressive ions to easily reach the substrate and causes pitting at the studied potentials. In contrast, the RCeMo film causes a slight shift of the corrosion potential to more positive values, suggesting a low barrier effect against a corrosive species.
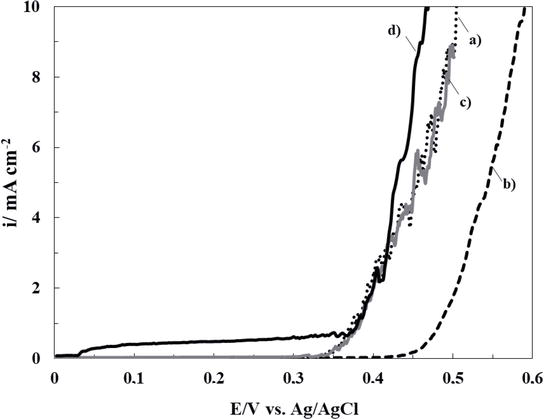
Figure 8.
Polarization behavior in ringer solution of 316L SS electrode covered with: (a) RCeNaSa coating, (b) RCeMo coating, and (c) RCeHAsc coating. The scan rate was 0.001 Vs−1. The data of bare steel electrode is also included (d).
To evaluate the anticorrosion properties of 316L SS covered with the different coatings, Tafel polarization curves for the uncoated and coated substrates are presented in Figure 9. Table 1 lists the parameters obtained from extrapolation of the curves. As can be seen from Figure 9, none of the cerium coatings reduce the rate of corrosion the corrosion rate of the substrate in Ringer’s solution, but they shift the corrosion potential to more anodic values. On the other hand, the data in Table 1 show that for RCeNaSa coated sample, the Ecorr value increases by 280 mV compared to bare 316L SS. According to the above results, it can be suggested that RCeNaSa sample coating presents the best corrosion resistance in Ringer solution. The anticorrosive properties are associated as previously reported; the improved inhibition performance of RCeNaSa is due to a synergistic effect of this compound in which both components, which are inhibitors of 316L SS by themselves, are simultaneously incorporated into a surface coating, as shown by the above results. This is because the organic component (NaSa) is predominantly an anodic inhibitor [29], while the cerium (under these circumstances) proves to be predominantly a cathodic inhibition [31].
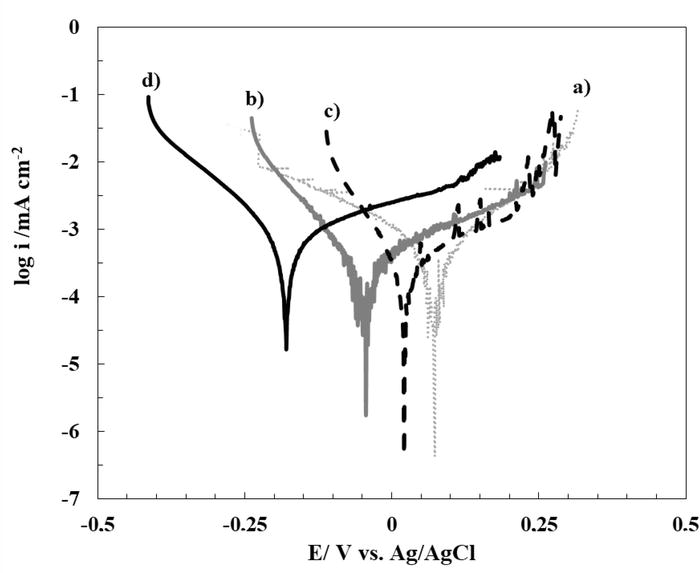
Figure 9.
Tafel curves obtained in ringer solution of 316L SS electrode covered with: (a) RCeNaSa coating, (b) RCeMo coating, and (c) RCeHAsc film. Tafel polarization plot of the uncoated steel is also included (d). The scan rate was 0.001 Vs−1.
Sample | Ecorr (V) | icorr (mA cm−2) |
---|---|---|
RCeNaSa | 0.100 ± 0.018 | 5.0 × 10−4 ± 2.35 × 10−6 |
RCeMo | −0.016 ± 0.017 | 4.5 × 10−5 ± 1.56 × 10−6 |
RCeHAsc | 0.016 ± 0.015 | 4.0 × 10−6 ± 1.70 × 10−7 |
316L SS | −0.180 ± 0.013 | 5.0 × 10−4 ± 3.55 × 10−6 |
Table 1.
Considering the obtained results, it was decided to continue the studies with the 316L SS electrode covered with RCeNaSa coating. In a further step and with the aim of obtaining antibacterial properties, the 316L SS electrode covered with RCeNaSa coating was modified with silver nanoparticles. The incorporation of AgNps on the cerium-salicylate based film from a solution containing 0.01 M AgNO3 and 0.1 M KNO3, was carried out using a double-pulse technique. When RCeNaSa is modified with AgNps, the surface becomes silvery. Now, RCeNaSaAg also showed the morphology of mud cracks, but in this case, it was completely covered with AgNps with spherical morphology (Figure 10A). The size of the nanospheres is estimated by SEM to be between 50 and 150 nm. This type of silver nanospheres morphology was previously studied by Wang and Zheng, who electrodeposited silver nanoparticles on a zinc oxide layer [37]. As expected, the EDX spectrum of RCeNaSaAg showed the signal of Ag, confirming the presence of silver nanoparticles.
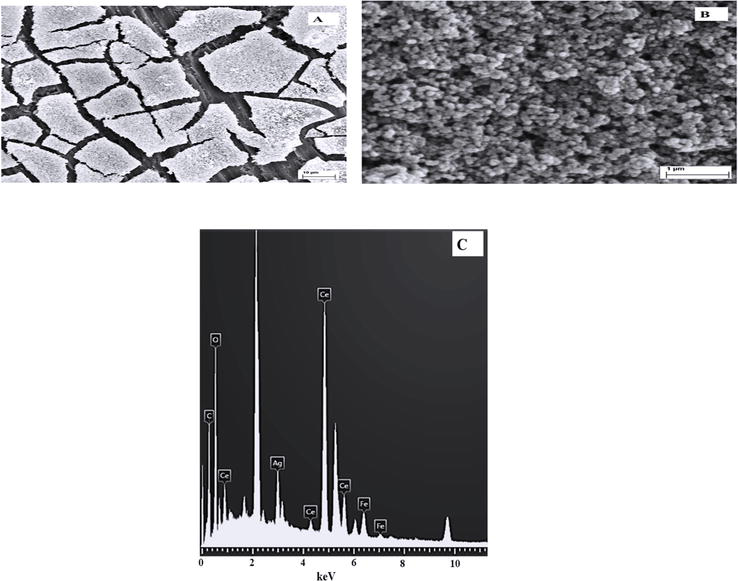
Figure 10.
(A) SEM image of the 316L SS covered with RCeNaSaAg coating, (B) magnified area of (A), and (C) EDX examination.
5. Antibacterial activity of RCeNaSa coating
The next step was evaluating the antibacterial activity of RCeNaSa film modified with AgNps against the Gram-negative bacterium
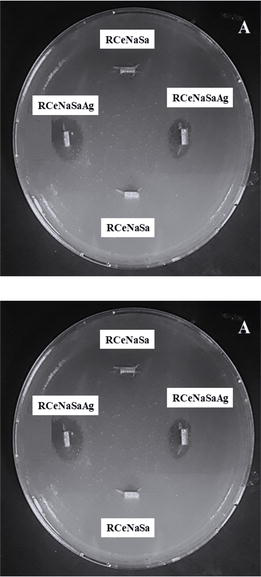
Figure 11.
Photograph of the inhibition zone diameter against
In latest years, several interrelated studies have aimed to improve the use of AgNps for bacterial inhibition. However, the debate on the bactericidal mechanism of action of AgNps continues. It has been shown that the antibacterial properties of AgNps are mainly due to a two-step mechanism. First, Ag+ ions are released from the nanoparticles. Then, the Ag+ ions take interaction with cellular targets, leading to their antibacterial effect [27]. Another proposed mechanism is cellular uptake of AgNps, which is related to physical interaction and takes place when the Nps are small enough to cross the cell membrane [38]. Mukha et al. reported that AgNps smaller than 10 nm present antibacterial activity due to both membrane damage and their ability to penetrate into the cell [39]. In general, bacterial cells have micron-scale size, while their outer cell membranes have nanometer-scale pores (< 50 nm). As the AgNps synthesized in this study have larger size than the outer cell membrane pores, it can be assumed that they cannot penetrate the cell membrane.
6. Conclusion
Anticorrosive, adherent, and morphologically homogeneous cerium-based films were obtained on 316L SS in solutions containing cerium nitrate, hydrogen peroxide, and three different additives (NaSa, Na2MoO4, and HAsc). The corrosion resistance of all RCe films electrogenerated in the presence of additives is superior to RCe coating in simulated body fluid solution. The RCeNaSa-coated 316L SS showed
Acknowledgments
CONICET, ANPCYT and Universidad Nacional del Sur, Bahía Blanca, Argentina are acknowledged for financial support.
Nomenclature
Silver nanoparticles | |
Energy Dispersive X-Ray Spectroscopy | |
Ascorbic acid | |
Sodium salycilate | |
Sodium molybdate | |
Cerium-based coating obtained at - 0.70 V in 30 mM Ce(NO3)3, 25 mM H2O2 solution | |
Cerium-based coating obtained at - 0.70 V in 30 mM Ce(NO3)3, 25 mM H2O2, and 5 mM NaSa solution | |
Cerium-based coating obtained at - 0.70 V in 30 mM Ce(NO3)3, 25 mM H2O2, and 5 mM NaSa solution. The AgNps were synthesized from a solution containing 0.01 M AgNO3 and 0.1 M KNO3, was carried out using a double-pulse technique. | |
Cerium-based coating obtained at - 1.00 V in 30 mM Ce(NO3)3, 25 mM H2O2, and 15 mM Na2MoO4 solution | |
Cerium-based coating obtained at - 0.80 V in 50 mM Ce(NO3)3, 25 mM H2O2, and 1 mM HAsc solution | |
316L stainless steel |
References
- 1.
Katta PPK, Nalliyan R. Corrosion resistance with self-healing behavior and biocompatibility of Ce incorporated niobium oxide coated 316L SS for orthopedic applications. Surface and Coatings Technology. 2019; 375 :715-726. DOI: 10.1016/j.surfcoat.2019.07.042 - 2.
Pandey AK, Kumar A, Kumar R, Gautam RK, Behera CK. Tribological performance of SS 316L, commercially pure titanium, and Ti6Al4V in different solutions for biomedical applications. Materials Today Proceedings. 2023; 78 :A1-A8. DOI: 10.1016/j.matpr.2023.03.736 - 3.
Wu G, Zhang S, Wang Y, Sun M, Qli Z, Kovalenko V, et al. Porous ceramic coating formed on 316L by laser cladding combined plasma electrolytic oxidation for biomedical application. Transactions of the Nonferrous Metals Society of China. 2022; 32 :2993-3004. DOI: 10.1016/S1003-6326(22)65998-3 - 4.
Say Y, Aslan N, Alla AMA, Özmen H. Influence of chemical etchings on surface properties, in-vitro degradation and ion releases of 316L stainless steel alloy for biomedical applications. Materials Chemistry and Physics 2023; 295: 127139-127153 DOI:10.1016/j.matchemphys.2022.127139. - 5.
Zhuang Y, Zhang S, Yang K, Ren L, Dai K. Antibacterial activity of copper-bearing 316L stainless steel for the prevention of implant-related infection. Journal of Biomedical Materials Research Part B: Applied Biomaterials. 2020; 108 :484-495. DOI: 10.1002/jbm.b.34405 - 6.
Hayajneh MT, Almomani M, Al-Daraghmeh M. Development and evaluation of a thin cerium oxide-gelatin nanolaminate coating for corrosion protection of AISI 316L stainless steel. Manufacturing Technology. 2021; 21 :330-339. DOI: 10.21062/mft.2021.036 - 7.
Rajeshkumar S, Naik P. Synthesis and biomedical applications of cerium oxide nanoparticles – A review. Biotechnology Reports. 2018; 17 :1-5. DOI: 10.1016/j.btre.2017.11.008 - 8.
Yang S, Sun R, Chen K. Self-healing performance and corrosion resistance of phytic acid/cerium composite coating on microarc-oxidized magnesium alloy. Chemical Engineering Journal. 2022; 428 :131198-131210. DOI: 10.1016/j.cej.2021.131198 - 9.
Calado LM, Taryba MG, Morozov Y, Carmezim MJ, Montemor MF. Novel smart and self-healing cerium phosphate-based corrosion inhibitor for AZ31 magnesium alloy. Corrosion Science. 2020; 170 :108648-108660. DOI: 10.1016/j.corsci.2020.108648 - 10.
Yeasmin F, Choi HW. Natural salicylates and their roles in human health. International Journal of Molecular Sciences. 2020; 21 :1-16. DOI: 10.3390/ijms21239049 - 11.
Hamdaoui S, Lambert A, Khireddine H, Agniel R, Cousture A, Coulon R, et al. An efficient and inexpensive method for functionalizing metallic biomaterials used in orthopedic applications. Colloids and Interface Science Communication. 2020; 37 :100282-100291. DOI: 10.1016/j.colcom.2020.100282 - 12.
Ananth KP, Nathanael AJ, Jose SP, Oh TH, Mangalaraj D. A novel silica nanotube reinforced ionic incorporated hydroxyapatite composite coating on polypyrrole coated 316L SS for implant application. Materials Science and Engineering: C. 2016; 59 :1110-1124. DOI: 10.1016/j.msec.2015.10.045 - 13.
Jabara R, Chronds N, Robinson K. Novel bioabsorbable salicylate-based polymer as a drug-eluting stent coating. Catheterization and Cardiovascular Interventions. 2008; 72 :186-194. DOI: 10.1002/ccd.21607 - 14.
Li X, Deng S, Fu H. Sodium molybdate as a corrosion inhibitor for aluminium in H3PO4 solution. Corrosion Science. 2011; 53 :2748-2753. DOI: 10.1016/j.corsci.2011.05.002 - 15.
Kapp RWJr. Molybdenum In: Wexler P (Editor–in-Chief), Encyclopedia of Toxicology, third ed., Elsevier, London. 2014 p. 383-388. ISBN-13: 978-0123864543; ISBN-10: 0123864542 - 16.
Mu S, Du J, Jiang H, Li W. Composition analysis and corrosion performance of a Mo–Ce conversion coating on AZ91 magnesium alloy. Surface and Coating Technology. 2014; 254 :364-370. DOI: 10.1016/j.surfcoat.2014.06.044 - 17.
Hu J, Li Q , Zhong X, Zhang L, Chen B. Composite anticorrosion coatings for AZ91D magnesium alloy with molybdate conversion coating and silicon sol–gel coatings. Progress in Organic Coating. 2009; 66 :199-205. DOI: 10.1016/j.porgcoat.2009.07.003 - 18.
González MB, Saidman SB. Electrodeposition of Polypyrrole on 316 stainless steels for corrosion prevention. Corrosion Science. 2011; 53 :276-282. DOI: 10.1016/j.corsci.2010.09.021 - 19.
Ferrerira ES, Giacomellu C, Spinelli A. Evaluation of the inhibitor effect of L-ascorbic acid on the corrosion of mild steel. Materials Chemistry and Physics. 2004; 83 :129-134. DOI: 10.1016/j.matchemphys.2003.09.020 - 20.
Akrout H, Bousselmi L, Triki E, Maximovitch S, Dalard F. Effect of non-toxic corrosion inhibitors on steel in chloride solution. Journal of Materials Science. 2004; 39 :7341-7350. DOI: 10.1023/B:JMSC.0000048749.31437.b9 - 21.
Valek L, Martinez S, Mikulic D, Brnardic I. The inhibition activity of ascorbic acid towards corrosion of steel in alkaline media containing chloride ions. Corrosion Science. 2008; 50 :2705-2709. DOI: 10.1016/j.corsci.2008.06.018 - 22.
Fuchs-Godex R, Pavlovic M, Tomic M. The inhibitive effect of vitamin-C on the corrosive performance of steel in HCl solutions. International Journal of Electrochemical Science. 2013; 8 :1511-1519 - 23.
Xin S, Xu R, Yan H, Shen P, Li M. Electrochemical corrosion behaviour of cerium chemical conversion coatings on 304 stainless steel in alkaline solutions containing chloride ions. International Journal of Electrochemical Science. 2019; 14 :11012-11018. DOI: 10.20964/2019.12.84 - 24.
Abdulaah HA, Ghaban AA, Anaee RA, Khadom AA, Kadhim MM. Cerium-tricalcium phosphate coating for 316L stainless steel in simulated human fluid: Experimental, biological, theoretical, and electrochemical investigations. Journal of Electrochemical Science and Engineering. 2023; 13 :115-126. DOI: 10.5599/jese.1257 - 25.
Alias R, Mahmoodian R, Abd Shukor MH. Development and characterization of a multilayer silver/silver-tantalum oxide thin film coating on stainless steel for biomedical applications. International Journal of Adhesion and Adhesives. 2019; 92 :89-98. DOI: 10.1016/j.ijadhadh.2019.04.010 - 26.
Wang H, Xu X, Wang X, Qu W, Qing Y, Li S, et al. Performance optimization of biomimetic ant-nest silver nanoparticle coatings for antibacterial and osseointegration of implant surfaces. Biomaterials Advances. 2023; 149 :213394. DOI: 10.1016/j.bioadv.2023.213394 - 27.
Sotiriou GA, Meye A, Knijnenburg JT, Panke S, Pratsinis SE. Quantifying the origin of released Ag+ ions from nanosilver. Langmuir. 2012; 28 :15929-15936. DOI: 10.1021/la303370d - 28.
Loperena AP, Lehr IL, Saidman SB. Cerium oxides for corrosion protection of AZ91D Mg alloy. In: Khan SB, Akhtar K, editors. Cerium Oxide—Applications and Attributes. London: IntechOpen; 2019. pp. 23-41. DOI: 10.5772/intechopen.79329 - 29.
González MB, Saidman SB. Corrosion protection properties of polypyrrole electropolymerized onto steel in the presence of salicylate. Progress in Organic Coating. 2012; 75 (3):178-183. DOI: 10.1016/j.porgcoat.2012.04.015 - 30.
Loperena AP, Lehr IL, Saidman SB. Formation of a cerium conversion coating on magnesium alloy using ascorbic acid as additive. Characterisation and anticorrosive properties of the formed films. Journal of Magnesium and Alloys. 2016; 4 (4):278-285. DOI: 10.1016/j.jma.2016.10.002 - 31.
Breslin C, Chen C, Mansfeld F. The electrochemical behaviour of stainless steels following surface modification in cerium containing solutions. Corrosion Science. 1997; 39 (6):1061-1073. DOI: 10.1016/S0010-938X(97)00007-3 - 32.
Chen S, Zhang S, Ren X, Xu S, Yin L. Cerium-based chemical conversion coating on aluminum alloy to inhibits corrosion in chloride solution. International Journal of Electrochemical Science. 2015; 10 :9073-9088 - 33.
Wang C, Zhu S, Jiang F, Wang F. Cerium conversion coatings for AZ91D magnesium alloy in ethanol solution and its corrosion resistance. Corrosion Science. 2009; 51 :2916-2923. DOI: 10.1016/j.corsci.2009.08.003 - 34.
Lehr IL, Saidman SB. Corrosion protection of AZ91D magnesium alloy by a cerium-molybdenum coating-the effect of citric acid as an additive. Journal of Magnesium and Alloys. 2018; 6 :356-365. DOI: 10.1016/j.jma.2018.10.002 - 35.
Hamlaoui Y, Pedraza F, Remazeilles C, Cohendoz S, Rébéré C, Tifouti L, et al. Cathodic electrodeposition of cerium-based oxides on carbon steel from concentrated cerium nitrate solutions: Part I, electrochemical and analytical characterization. Materials Chemistry and Physics. 2009; 113 :650-657. DOI: 10.1016/j.matchemphys.2008.08.027 - 36.
Sudesh TL, Wijesinghe L, Blackwood DJ. Real time pit initiation studies on stainless steels: The effect of sulphide inclusions. Corrosion Science. 2007; 49 :1755-1764. DOI: 10.1016/j.corsci.2006.10.025 - 37.
Wang Q , Zheng J. Electrodeposition of silver nanoparticles on a zinc oxide film: Improvement of amperometric sensing sensitivity and stability for hydrogen peroxide determination. Microchimica Acta. 2010; 169 :361-365. DOI: 10.1007/s00604-010-0356-7 - 38.
McQuillan JS, Shaw AM. Differential gene regulation in the Ag nanoparticle and Ag(+)-induced silver stress response in Escherichia coli: A full transcriptomic profile. Nanotoxicology. 2014; 8 (Suppl. 1):177-184. DOI: 10.1021/la303370d - 39.
Mukha IP, Eremenko AM, Smirnova NP, Mikhienkova AI, Korchak GI, Gorchev VF, et al. Antimicrobial activity of stable silver nanoparticles of a certain size. Applied Biochemistry and Microbiology. 2013; 49 :199-206. DOI: 10.1134/S0003683813020117