Abstract
Total hip arthroplasty (THA) is a highly effective technique for relieving pain and reconstructing joint structures. However, even several years after THA, the preexisting muscle weakness does not resolve to the level of healthy individuals. Since the 2000s, minimally invasive surgical procedures and fast-track rehabilitation have enabled early functional recovery, particularly in terms of walking ability, but solutions to these problems have not yet been established. The benefits of combined nutrition and exercise interventions for sarcopenia and frailty are gaining widespread acceptance. Elements of sarcopenia and frailty may be inherently present in patients who have undergone THA, and a combination of nutritional and exercise interventions may be useful in treating post-prosthetic muscle weakness and prolonged muscle atrophy. This chapter describes their usefulness and implementation for patients who have undergone THA.
Keywords
- nutritional support
- muscle atrophy
- nutritional physiology
- total hip arthroplasty
- muscle weakness
1. Introduction
Total hip arthroplasty (THA) is an excellent surgical technique to alleviate hip pain and improve activities of daily living (ADL) and quality of life (QOL) in patients with end-stage hip osteoarthritis, and the number of THAs performed worldwide has increased year after year [1]. Particularly, THA has helped achieve favorable long-term results due to innovations in prosthetic implants and surgical techniques in recent years [1, 2].
However, most patients undergoing THA develop muscle atrophy and weakness due to joint deformity and decreased physical activity [3, 4], require a prolonged postoperative functional recovery period [5, 6], and are at increased risk of serious complications, including falls [7], dislocations [8, 9], and infections [10]. In addition, prolonged muscle atrophy and weakness after surgery have negative consequences, such as a higher risk of frailty and care needs [11, 12], postoperative complications, and an increased strain on social resources [13]. Therefore, there is an urgent need to understand the factors associated with muscle atrophy and weakness in patients who have undergone THA and to implement more effective interventions.
2. Functional and physiological changes in muscles before arthroplasty
Osteoarthritis (OA) and rheumatoid arthritis, for which THA is most commonly performed, are chronic diseases associated with changes in muscle function and quality due to both the disease and limb disuse. End-stage hip OA is associated with increased atrophy and fatty infiltration of the gluteus minor [13, 14], gluteus medius [13], and gluteus maximus muscles [14] in comparison to healthy individuals. The muscle mass of the gluteus maximus, gluteus medius, and gluteus minimus muscles in patients with end-stage OA showed 11.5, 6.9, and 13.7% asymmetry, respectively, compared to corresponding muscles in healthy individuals [14]. In the advanced stage of OA, a 12% asymmetry of the gluteus medius muscles has been reported as compared to healthy controls [15]. In addition, muscle biopsies of the quadriceps muscle have demonstrated atrophy of both type 2A and 2B muscle fibers [16]. A recent systematic review noted that muscle mass loss in patients with hip OA varied widely from muscle to muscle, while fatty infiltration was identified in several muscles [17, 18]. Against the background of these muscle mass and qualitative changes, the strength of the abductor muscle group is reduced compared to healthy subjects [13, 14, 17]. In terms of muscle activity, the gluteus minimus muscle in patients with OA is more active during the stance phase of walking compared to healthy individuals, with the degree of overactivity increasing with severity, while the gluteus medius muscle exhibits no difference [19]. On the other hand, it has been reported that there is an overall delay in muscle activity during stepping tasks, as well as increased activity in the gluteus minimus muscle, similar to walking [20].
Selective muscle atrophy of the vastus medialis muscle is observed in the early stages of knee OA [21]. Reduced quadriceps muscle strength is a risk factor for the progression of OA [22] and is 16–49% lower than in healthy older adults [21, 23, 24]. Clinical symptoms such as pain [25] and arthrogenic muscle inhibition (AMI) associated with joint edema [26] affect quadriceps muscle strength. Quadriceps muscle in knee OA patients exhibits more pronounced atrophy of type II fibers than type I fibers [27] and an increased proportion of non-contractile tissue within the muscle, such as fatty and connective tissue [21, 23, 24]. Maly et al. [28] found that females with advanced knee OA exhibit a greater percentage of fatty infiltration in the quadriceps muscle. Wada et al. [29] also reported that a one-kilogram increase in lower limb muscle mass for the joint protective effect could be expected to increase by 7.3 Nm in patients with early-stage knee OA, whereas it only increased by 3.8 Nm in end-stage knee OA. These reports confirm that changes in quadriceps muscle quality with the progression of knee OA affect muscle strength independently of muscle atrophy.
3. Dietary guidance and characteristics of dietary intake in patients with osteoarthritis
Dietary guidance for weight loss is widely used as a part of lifestyle guidance for osteoarthritis patients. However, obesity and metabolic syndrome are not risk factors for the progression of osteoarthritis [30, 31]. In contrast, Messier et al. [32, 33] reported that weight loss by restricting energy intake reduced joint pain and mechanical joint loading in knee OA patients; however, difficulties in maintaining energy intake restrictions have been noted for compliance and the long-term sustainability of weight loss. In addition, a recent scoping review suggested that the assessment for sarcopenic obesity should be included in osteoarthritis patients, as it is a risk factor for muscle weakness, loss of muscle mass, and post-prosthetic complications [34], and the risk of developing knee OA has been reported to increase approximately twofold [35]. With respect to necessary levels of protein, vitamins C, E, and omega-3 fatty acids, insufficient protein intake was observed to be more prevalent in older individuals with hip and knee osteoarthritis [36]. de Zwart et al. [37] also noted insufficient protein intake in most patients with knee OA. Additionally, there are also reports that a high intake of red meat is effective in reducing the need for THA [38]. Furthermore, adherence rates for the Mediterranean diet and dietary quality were significantly worse in OA patients [39], which suggests that a diet focused on anti-inflammatory effects may be beneficial [40].
4. Decrease in muscle mass at the perioperative period
The decrease in muscle mass after surgical procedures is partly due to accelerated catabolism caused by the surgical invasion and partly attributable to the muscle atrophy caused by rest. Surgical techniques for joint arthroplasty have developed rapidly, with mini-incision surgery techniques becoming more common since the 2000s, and surgical invasion and associated blood loss could be controlled [41]. Prevention of postoperative anemia is important because preoperative serum hemoglobin (Hb) is affected during the hospital stay after THA [42], and Hb decline after surgery affects lower limb muscle strength [43]. However, it was shown that moderate anemia had a limited impact on functional recovery [44].
In contrast, the effect of bed rest has been reported in patients with ankle fractures, where 7 days of bed rest with unloading resulted in a 6–16% reduction in the cross-sectional area of the quadriceps femoris muscle, whereas inpatients admitted to an intensive treatment unit experienced a 1.0–8.7% reduction over 3 days and an 8.8–13.7% reduction over 5 days [45]. In patients with hip fractures, type I muscle fibers did not differ among younger patients, while type II muscle fibers exhibited significant muscle atrophy compared to healthy older adults [46].
Enhanced Recovery After Surgery (ERAS) programs and early mobilization have become widespread in recent years and have been applied early in the area of arthroplasty. However, a systematic review [47] reported that employing ERAS after THA has no impact on functional improvement or complication prevention. This point should be considering Dreyer et al.’s [48] report of a decrease in muscle mass on both limbs on the operative and non-operative side after Total Knee Arthroplasty (TKA). Because patients requiring THA exhibit gluteal muscle atrophy before surgery, an assessment of postoperative muscle atrophy must also take into account the effects of increased bedrest, decreased loading of the lower limb that cannot be compensated by ERAS, selective atrophy of type II muscle fibers, and anemia due to blood loss.
5. Factors associated with medium- and long-term recovery in muscle strength and mass after THA
What will be the degree of functional improvement after early recovery and discharge by the ERAS program or fast-track surgery? Fukumoto et al. [49] found it to be lower than that of healthy controls [49, 50], although it did not reach preoperative levels at 1 month, but improved compared to preoperative values at 4 to 6 months, and hip abductor muscle strength was still affected by preoperative values at 6 months postoperatively. One year after THA, Judd et al. [51] reported that hip flexion and knee extension muscle strength were significantly lower in OA patients than in healthy individuals of the comparable age, whereas hip extension, abduction, and adduction muscle strength improved to the same level as healthy individuals of the same age. Recovery in muscle strength and mass up to 1 year after THA shows differences for each muscle group, reflecting differences in the site of muscle atrophy and fatty infiltration before THA (para 1). Furthermore, regarding the long-term course, it has also been reported that patients up to 6 years after THA did not achieve the level of healthy individuals of the comparable age [52], and similarly, hip abductor muscle strength remained significantly lower than in healthy individuals [53] after 10 years after THA.
Previous studies have shown that the hip and knee periprosthetic muscle strength after THA initially decreases compared to the level of preoperative strength up to about 1 month postoperatively but exceeds preoperative values at about 6 months postoperatively. Improvement thereafter is slower, and values are still lower than those of healthy individuals of comparable age more than 2 years after surgery. In other words, it can be inferred that in patients who have undergone THA, the hip and knee periprosthetic muscle weakness persists during the mid-to-long-term postoperative period. However, no consensus has been reached due to differences in surgical technique, femoral offset, and postoperative rehabilitation programs [54]. Furthermore, further research is needed on the medium- to long-term recovery of periprosthetic hip muscle strength and related factors, as there are few reports on patients who are more than 2 years postoperative.
Several observational studies on changes in skeletal muscle mass after THA have been published, supporting reports of prolonged muscle weakness over the medium and long term. Reports up to 2 years after surgery show that the muscle mass of the affected side does not improve to the level of the healthy side in the whole gluteus muscle group [55, 56], while it has been reported that there is no difference in the ratio of its muscle mass between the healthy and affected side 1 year after surgery when limited to the gluteus medius muscle. Isshiki et al. [57] found that in patients with posterior lateral THA over 8 years postoperatively, the muscle cross-sectional area of the affected side did not improve to the level of the healthy side throughout the entire preoperative, 3-year, and 8-year postoperative periods (Figure 1). These findings suggest that atrophy of the periprosthetic hip muscle, mainly the gluteus medius muscle, in patients who have undergone THA patients may persist in the mid-to-long-term postoperatively. However, this is debatable due to differences in age, surgical technique, and postoperative rehabilitation [58, 59, 60]. Further studies are needed to adjust for influencing factors such as surgical technique and postoperative rehabilitation.
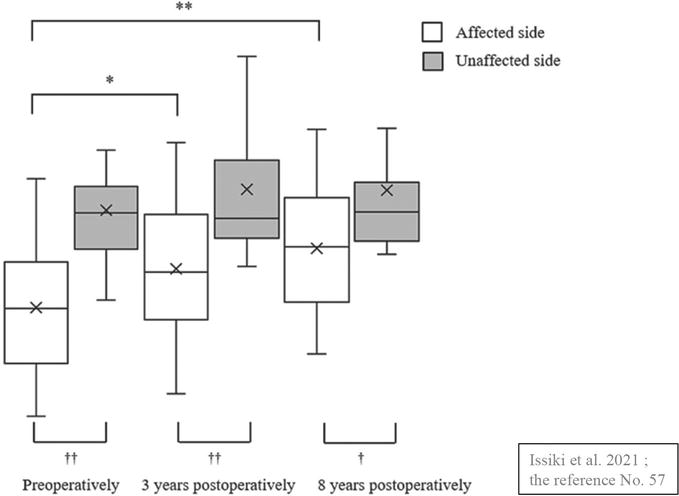
Figure 1.
Asymmetry of the cross-sectional area of the gluteus medius muscle. In the cross-sectional area of the gluteus medius muscle from preoperative to 8 year after THA, the muscle mass on the operated side was still significantly lower than on the healthy side at 8 years after THA, although it recovers over time. Source: Isshiki et al. [
6. Sarcopenia-frail as a comorbidity after THA
Sarcopenia as a comorbidity has received increased attention in recent years. Regarding the prevalence of sarcopenia, Chang et al. [61] reported that of the 307,678 patients who underwent THA, 1319 patients (0.43%) had a prior diagnosis of sarcopenia, while Koto et al. [62] found it to be 8%, with differences in both reports, these prevalence ratios were less than the 47.3% of proximal femur fractures [63]. However, preoperative physical function was impaired in patients with concomitant sarcopenia [64], and they are at an increased risk of complications due to implant-related dislocation in the first year postoperatively [61]. Additionally, a higher incidence of falls and fragility fractures is observed [61, 65], resulting in higher readmission rates and healthcare costs [61]. At 1-year follow-up, patients with concomitant sarcopenia who had undergone THA were 62% more likely to fall and 77% more likely to develop fragility fractures [41], which suggests that falls and fragility fractures not only reduce the QOL but also increase patient mortality [65].
Frailty after THA has been reported to increase the incidence of postoperative complications, revision surgery, readmission, and mortality, as well as prolong hospital stays, as with sarcopenia, and ultimately increase healthcare costs [66, 67, 68, 69, 70]. Therefore, early diagnosis and prevention of frailty in patients who have received THA are urgent issues. We investigated the prevalence of frailty and associated factors in community-dwelling elderly patients 1 year following THA and found a prevalence of 11.2% for frailty and 51.0% for pre-frailty [11]. The results showed a higher percentage compared to the prevalence in Japanese community-dwelling older adults (frail: 8.7%, pre-frail: 40.8%). In addition, the history of falls, maximum leg circumference, hip abductor strength, knee extensor strength, and performance on the Timed up & go test were significantly associated with frailty in patients who had undergone THA. These findings suggest that improving muscle mass and dynamic balance capacity as well as increasing muscle strength, particularly hip abductor and knee extensor strength, may be important to prevent frailty after THA. However, there are few reports on the coexistence of osteoarthritis and frailty [71, 72], and the causal relationship with frailty is also unclear [73].
7. Consensus on therapeutic interventions for sarcopenia and frailty
Research on sarcopenia has progressed dramatically in the last decade, and in recent years’ guidelines [74], consensus papers [75, 76], and position papers [77] have been successively published. The common view shared by these publications is that treatments can improve muscle strength and mass when combined with exercise and nutritional therapy. Muscle mass loss can occur under any of the conditions of sarcopenia, malnutrition, and cachexia [76], but each of these factors needs to be taken into account, as they are exacerbated by one another [77]. The recommended exercise intensity is 50% of 1 repetition maximum (1RM) and 80% of 1RM for muscle hypertrophy if feasible [78]. For nutritional therapy, a protein intake of 1–1.5 g per kg body weight per day is recommended, and there is insufficient evidence regarding the effectiveness of vitamin D [77]. The International Clinical Practice Guidelines for Sarcopenia [74] also note the importance of exercise therapy and protein-rich diet or supplementation, as well as education on these treatments.
Sarcopenia is also encompassed within the concept of frailty and overlaps with physical frailty in many aspects [79]. As with sarcopenia, several guidelines [80, 81] and recommendations [82] have been reported. As with sarcopenia, a combination of exercise and nutritional therapy is recommended, with exercise therapy encompassing resistance training and nutritional therapy consisting of protein and caloric supplementation [80, 81, 82]. In addition, vitamin D is recommended only in Asian regions [75], and cognitive or problem-solving therapy is not recommended [81]. Also, a recent recommendation has proposed screening for frailty in inpatients over 70 years of age [82].
8. Fast-track surgery and rehabilitation in THA
Fast-track surgery is a multimodal effort involving a multidisciplinary team of anesthetists, physiotherapists, and nurses in addition to the surgeon. Enhanced recovery requires a combination of preoperative education, stress reduction, pain relief, early ambulation and mobilization, drain and tube management and their removal to enable mobility, as well as fluid and nutritional therapy [83]. Fast-track surgery in THA, together with minimally invasive techniques, facilitates early functional recovery and discharge through multimodal pain management [84] and clinical nursing pathways [85]. This fast-track initiative was also reported to have no problems in terms of medical safety [86]. Compared to conventional perioperative management, fast-track surgery has been reported to reduce hospital stay [87], which is becoming more prevalent in patients undergoing THA, although there is less emphasis on nutritional support.
The main outcomes of fast-track surgery in THA are early mobility and discharge from the hospital. As described in the previous paragraphs, patients who have undergone THA exhibit the following characteristics: the preoperative presence of disuse changes in muscle function and qualitative aspects of physiology (par. 1), poor protein intake and adherence rate (par. 2), increased bedrest and decreased loading of the lower limb that cannot be compensated for even with the ERAS program, and selective atrophy of type II muscle fibers (par. 3). Additionally, prolonged muscle weakness in the mid-to-long-term postoperative period (par. 4) and the coexistence of frailty and sarcopenia in patients who received THA (par. 5) have also been identified, which have not been adequately addressed by initiatives such as fast-track surgery and rehabilitation. As the consensus for the combination of exercise and nutritional therapy as a therapeutic intervention in frailty and sarcopenia grows, initiatives to incorporate nutritional therapy into the fast-track component of THA are emerging.
9. Combination of exercise and nutritional interventions and their effects before surgery
Resistance training in combination with high protein intake for patients with OA has been suggested in systematic reviews to be potentially useful in improving lower limb muscle strength and motor function [88]. In one of the reviews, Ikeda et al. [89] randomly provided 6 g of branched-chain amino acid (BCAA) for 4 weeks to patients with end-stage hip OA scheduled for THA. An improvement in hip abductor muscle strength on the healthy side was observed (Figure 2), but they reported no difference in the affected side’s muscle strength compared to the placebo. One reason for this is that resistance exercise in combination with nutritional therapy has been reported to have no significant effect on improving muscle mass, muscle strength, and physical functions in healthy older people [90, 91]. In other words, the additional effect of nutritional supplementation is unlikely to be observed or limited in older people who were able to obtain sufficient amounts of nutrition, including protein, from their daily diet. As common challenges in OA patients, inadequate protein intake [36], and poor adherence to a Mediterranean diet are prevalent [39]. Future large-scale studies are needed to assess confounding factors such as age, nutritional status, daily diet, and physical activity of the participants and to adjust for these factors.
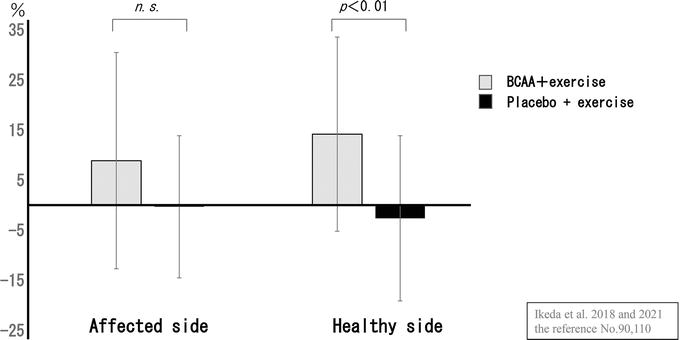
Figure 2.
Improvement rate in hip abductor strength (patients with hip OA). The BCAA group showed a greater rate of improvement on both sides and was significantly higher on the healthy side. Source: Ikeda et al. [
10. Combination of exercise and nutritional interventions and their effects after THA
In recent years, patients have been able to attain early mobility following THA, from the day of surgery due to remarkable advances in prosthetic implants and surgical techniques, which have also enabled the reduction of healthcare costs and hospital stays [92, 93, 94]. However, it has also been noted that approximately 70% of elderly inpatients for THA were discharged with the same degree of physical function as before surgery [95] and that prolonged functional decline is associated with muscle atrophy and weakness. Most of the patients who underwent THA exhibit inadequate nutritional intake preoperatively [36, 37]. This malnutrition not only prolongs functional recovery but also increases the incidence of postoperative complications, readmission rates, and hospital stays [61, 96]. In short, we believe that exercise therapy in combination with nutritional interventions, rather than exercise therapy alone, can maximize the induction of muscle protein synthesis, reduce muscle atrophy, and lead to a more efficient and early functional recovery.
Two randomized controlled trials (RCTs) in patients who underwent TKA and two in those who received THA have examined the influence of dietary intake on functional recovery following arthroplasty. In perioperative TKA patients, Ueyama et al. [97] conducted an RCT in which 3 g of essential amino acids (EAA) was provided three times daily from 1 week before to 2 weeks after surgery and investigated quadriceps muscle atrophy, knee extension muscle strength, and ADL ability up to 4 weeks after TKA. The authors reported that the intervention group showed significantly reduced postoperative muscle atrophy and early improvement in ADL functions. In addition, Dreyer et al. [48] conducted an RCT in perioperative patients planned for TKA and who were administered 20 g of EAA twice daily from 1 week before to 6 weeks after surgery to investigate quadriceps muscle atrophy and knee extensor strength up to 6 weeks after TKA. They reported significantly reduced postoperative muscle atrophy in the intervention group but no significant difference in knee extensor strength.
Ninomiya and Ikeda [98] compared 29 perioperative THA patients who were administered a high protein dietary supplement containing 3.4 g BCAA twice daily from 4 weeks before surgery to 8 weeks after surgery (BCAA group) with 29 patients who were administered a regular program (control group). They compared hip abductor strength and knee extensor strength between both groups for up to 8 weeks after surgery. The strength of hip abductors on the affected side did not differ between the two groups, whereas the strength of hip abductors on the healthy side and bilateral knee extensors was considerably greater in the BCAA group. In a study of patients who underwent THA and were in the convalescent rehabilitation period, Ikeda et al. [99] compared 18 patients (the BCAA group) who were provided with 3.4 g BCAA once daily for 1 month in conjunction with physiotherapy twice daily and 13 patients (the control group) who were provided with 1.2 g placebo with physiotherapy twice daily. They found that the BCAA prevented a decrease in skeletal muscle mass and reported significantly higher values for the knee extensor muscles on the operated side (Figures 3 and 4). However, no significant difference was observed in bilateral hip abduction muscle strength between both groups. These reports suggest that adequate nutritional intake in patients requiring THA or TKA may prevent postoperative muscle atrophy and have a limited effect on muscle strength and functional recovery, although this is debatable. This is because (1) AMI due to swelling and pain is associated with early postoperative muscle strength [26, 100, 101, 102]; (2) the effects of nutritional intake may be synergistic when combined with resistance training [103, 104], and additionally, the type, amount, and timing of nutrient intake may play a role. Future studies are needed to examine the effect of nutritional intake on functional recovery in patients undergoing THA after adjusting for these factors.
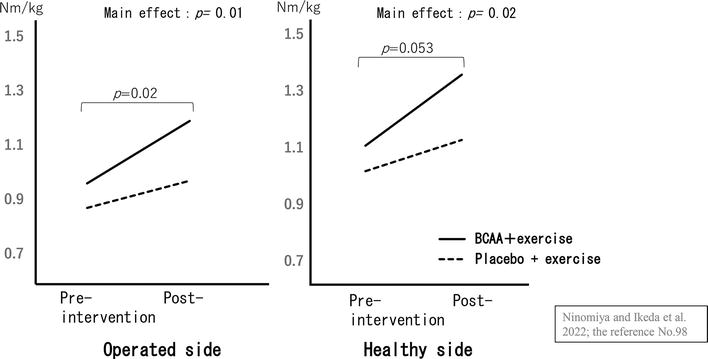
Figure 3.
Knee extensor strength pre-intervention and post-in-perioperative THA patients. There was no interaction between the two groups in terms of change in knee extensor strength. However, there was a significant main effect between groups and at time on the both side. Source: Ninomiya et al. [
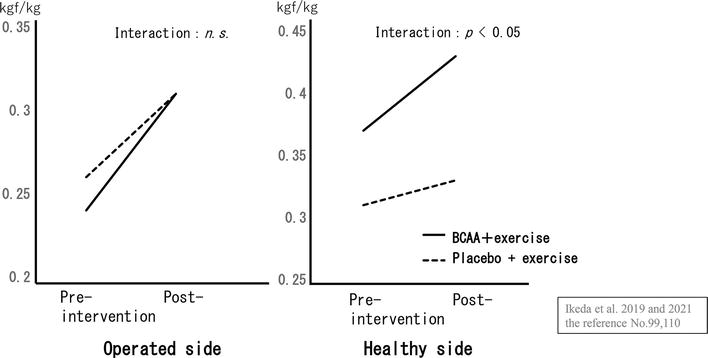
Figure 4.
Interaction plot of knee extensor strength pre-intervention and post-in convalescent THA patients. A two-way analysis of variance showed an interaction (synergy) between exercise and nutritional therapy in knee extensor strength on the healthy side. Source: Ikeda et al. [
11. Problems in preventing prolonged muscle weakness after THA
We have previously reported that limb muscle strength and motor function in patients 10 years after THA were significantly lower, and the incidence of falls was 2.8 times higher than in healthy individuals of comparable age [53]. The incidence of fractures resulting from falls was reported to be about twice as high as the incidence of implant-related postoperative complications (dislocation and wear) [105]. While THA in recent years has undergone dramatic advancements in prosthetic implants that have reduced the incidence of revision surgery due to dislocation and wear and increased implant survival rates, patients are likely to be at increased risk of requiring care due to functional decline associated with age-related loss of muscle mass and strength. Currently, physiotherapy for patients undergoing THA includes exercise therapy and patient education with a focus on resistance training, with outcomes of improved lower limb muscle strength, walking ability, ADL functions, and QOL [106, 107]. However, recent studies have reported that physiotherapy after THA is as effective as independent training [108], pointing to the need to reconsider intervention methods. We reported that protein intake was associated with muscle strength in patients 1 year after THA [109]. Ueyama et al. [110] also reported that nutritional interventions were associated with enhanced muscle mass and strength in patients 2 years after THA. Therefore, to maintain and improve muscle mass, strength, and motor function in patients in the mid-to long-term following THA, it may be necessary not only to use exercise therapy but also to monitor the nutritional status of the patient and to use nutritional supplementation in combination (Figure 5).
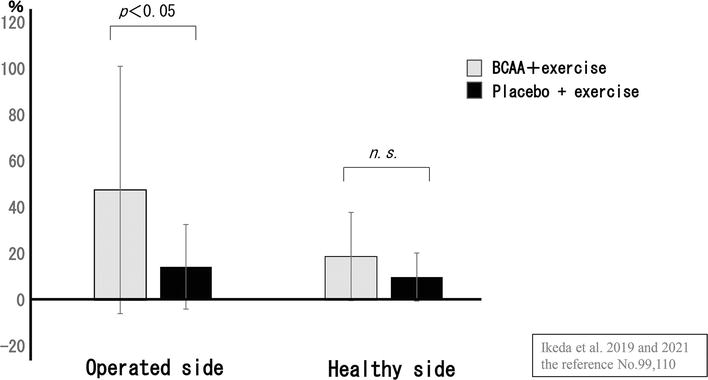
Figure 5.
Improvement rate in knee extensor strength (convalescent THA patients). The BCAA group showed that improvement rate in knee extensor strength was significantly higher on the healthy side. Source: Ikeda et al. [
12. Conclusion
Postoperative outcomes in patients undergoing THA have been highly successful in terms of early postoperative functional recovery and implant survival. We had assumed that the prolonged muscle weakness following THA would resolve with time. However, recent findings have indicated a situation that worsens with time. Fast-track rehabilitation, which focuses on a combination of exercise and nutritional therapy as a strategy to address preexisting muscle atrophy, fatty infiltration, and postoperative challenges, is a new technique that may provide a new tool for mitigating the problem of prolonged postoperative muscle weakness, which has remained unresolved for 20 years.
Acknowledgments
This book chapter writing was funded by Showa University Research Administration Center (Grant Number: 13000157).
Conflict of interest
The authors declare no conflict of interest.
References
- 1.
Fontalis A, Epinette JA, Thaler M, et al. Advances and innovations in total hip arthroplasty. SICOT Journal. 2021; 7 :26. DOI: 10.1051/sicotj/2021025 - 2.
Hopper RH Jr, Ho H, Sritulanondha S, et al. Crosslinking reduces THA Wear, osteolysis, and revision rates at 15-year Followup compared with noncrosslinked polyethylene. Clinical Orthopaedics and Related Research. 2018; 476 (2):279-290. DOI: 10.1007/s11999.0000000000000036 - 3.
Rasch A, Byström AH, Dalen N, et al. Reduced muscle radiological density, cross-sectional area, and strength of major hip and knee muscles in 22 patients with hip osteoarthritis. Acta Orthopaedica. 2007; 78 (4):505-510. DOI: 10.1080/17453670710014158 - 4.
Loureiro A, Constantinou M, Diamond LE, et al. Individuals with mild-to-moderate hip osteoarthritis have lower limb muscle strength and volume deficits. BMC Musculoskeletal Disorders. 2018; 19 (1):303. DOI: 10.1186/s12891-018-2230-4 - 5.
Nanri Y, Shibuya M, Nozaki K, et al. The impact of sarcopenia risk on postoperative walking independence in older adults undergoing total joint arthroplasty. Journal of Geriatric Physical Therapy. 2024; 47 (1):28-35. DOI: 10.1519/JPT.0000000000000368 - 6.
Nankaku M, Tsuboyama T, Aoyama T, et al. Preoperative gluteus medius muscle atrophy as a predictor of walking ability after total hip arthroplasty. Physical Therapy Research. 2016; 19 (1):8-12. DOI: 10.1298/ptr.e9884 - 7.
Ninomiya K, Takahira N, Ikeda T, et al. Predictors of falls in patients during the first year after total hip arthroplasty: A prospective cohort study. Health Science Reports. 2020; 3 (3):e184. DOI: 10.1002/hsr2.184 - 8.
García-Rey E, García-Cimbrelo E. Abductor biomechanics clinically impact the total hip arthroplasty dislocation rate: A prospective long-term study. The Journal of Arthroplasty. 2016; 31 (2):484-490. DOI: 10.1016/j.arth.2015.09.039 - 9.
Elbuluk AM, Coxe FR, Schimizzi GV, et al. Abductor deficiency-induced recurrent instability after Total hip arthroplasty. JBJS Reviews. 2020; 8 (1):e0164. DOI: 10.2106/JBJS.RVW.18.00164 - 10.
Babu JM, Kalagara S, Durand W, et al. Sarcopenia as a risk factor for prosthetic infection after Total hip or knee arthroplasty. The Journal of Arthroplasty. 2019; 34 (1):116-122. DOI: 10.1016/j.arth.2018.09.037 - 11.
Ninomiya K, Takahira N, Ikeda T, et al. Prevalence of frailty and associated factors among community-dwelling older adults after total hip arthroplasty. HIP International. 2023; 33 (3):397-403. DOI: 10.1177/11207000211048180 - 12.
Johnson RL, Frank RD, Abdel MP, et al. Frailty transitions one year after Total joint arthroplasty: A cohort study. The Journal of Arthroplasty. 2022; 37 (1):10-18.e2. DOI: 10.1016/j.arth.2021.08.022 - 13.
Kivle K, Lindland ES, Mjaaland KE, et al. Gluteal atrophy and fatty infiltration in end-stage osteoarthritis of the hip: A case-control study. Bone & Joint Open. 2021; 2-1 :40-47. DOI: 10.1302/2633-1462.21.BJO-2020-0179.R1 - 14.
Zacharias A, Pizzari T, English DJ, et al. Hip abductor muscle volume in hip osteoarthritis and matched controls. Osteoarthritis and Cartilage. 2016; 24 (10):1727-1735. DOI: 10.1016/j.joca.2016.05.002 - 15.
Grimaldi A, Richardson C, Stanton W, et al. The association between degenerative hip joint pathology and size of the gluteus medius, gluteus minimus and piriformis muscles. Manual Therapy. 2009; 14 :605-610. DOI: 10.1016/j.math.2009.07.004 - 16.
Reardon K, Galea M, Dennett X, et al. Quadriceps muscle wasting persists 5 months after total hip arthroplasty for osteoarthritis of the hip: A pilot study. Internal Medicine Journal. 2001; 31 (1):7-14. DOI: 10.1046/j.1445-5994.2001.00007.x - 17.
Marshall AR, de Noronha M, Zacharias A, et al. Structure and function of the abductors in patients with hip osteoarthritis: Systematic review and meta-analysis. Journal of Back and Musculoskeletal Rehabilitation. 2016; 29 (2):191-204. DOI: 10.3233/BMR-150614 - 18.
Lawrenson PR, Crossley KM, Vicenzino BT, et al. Muscle size and composition in people with articular hip pathology: A systematic review with meta-analysis. Osteoarthritis and Cartilage. 2019; 27 :181-195. DOI: 10.1016/j.joca.2018.10.008 - 19.
Zacharias A, Pizzari T, Semciw AI, et al. Comparison of gluteus medius and minimus activity during gait in people with hip osteoarthritis and matched controls. Scandinavian Journal of Medicine & Science in Sports. 2019; 29 (5):696-705. DOI: 10.1111/sms.13379 - 20.
Zacharias A, Pizzari T, Semciw AI, et al. Gluteus medius and minimus activity during stepping tasks: Comparisons between people with hip osteoarthritis and matched control participants. Gait & Posture. 2020; 80 :339-346. DOI: 10.1016/j.gaitpost.2020.06.012 - 21.
Taniguchi M, Fukumoto Y, Kobayashi M, et al. Quantity and quality of the lower extremity muscles in women with knee osteoarthritis. Ultrasound in Medicine & Biology. 2015; 41 (10):2567-2574. DOI: 10.1016/j.ultrasmedbio.2015.05.014 - 22.
Øiestad BE, Juhl CB, Culvenor AG, et al. Knee extensor muscle weakness is a risk factor for the development of knee osteoarthritis: An updated systematic review and meta-analysis including 46 819 men and women. British Journal of Sports Medicine. 2022; 56 (6):349-355. DOI: 10.1136/bjsports-2021-104861 - 23.
Kumar D, Karampinos DC, MacLeod TD, et al. Quadriceps intramuscular fat fraction rather than muscle size is associated with knee osteoarthritis. Osteoarthritis and Cartilage. 2014; 22 (2):226-234. DOI: 10.1016/j.joca.2013.12.005 - 24.
Noehren B, Kosmac K, Walton RG, et al. Alterations in quadriceps muscle cellular and molecular properties in adults with moderate knee osteoarthritis. Osteoarthritis and Cartilage. 2018; 26 (10):1359-1368. DOI: 10.1016/j.joca.2018.05.011 - 25.
Riddle DL, Stratford PW. Impact of pain reported during isometric quadriceps muscle strength testing in people with knee pain: Data from the osteoarthritis initiative. Physical Therapy. 2011; 91 (10):1478-1489. DOI: 10.2522/ptj.20110034 - 26.
Rice DA, McNair PJ. Quadriceps arthrogenic muscle inhibition: Neural mechanisms and treatment perspectives. Seminars in Arthritis and Rheumatism. 2010; 40 (3):250-266. DOI: 10.1016/j.semarthrit.2009.10.001 - 27.
Fink B, Egl M, Singer J, et al. Morphologic changes in the vastus medialis muscle in patients with osteoarthritis of the knee. Arthritis and Rheumatism. 2007; 56 (11):3626-3633. DOI: 10.1002/art.22960 - 28.
Maly MR, Calder KM, Macintyre NJ, et al. Relationship of intermuscular fat volume in the thigh with knee extensor strength and physical performance in women at risk of or with knee osteoarthritis. Arthritis Care & Research (Hoboken). 2013; 65 (1):44-52. DOI: 10.1002/acr.21868 - 29.
Wada O, Kurita N, Kamitani T, et al. Influence of the severity of knee osteoarthritis on the association between leg muscle mass and quadriceps strength: The SPSS-OK study. Clinical Rheumatology. 2019; 38 (3):719-725. DOI: 10.1007/s10067-018-4337-2 - 30.
Grotle M, Hagen KB, Natvig B, et al. Obesity and osteoarthritis in knee, hip and/or hand: An epidemiological study in the general population with 10 years follow-up. BMC Musculoskeletal Disorders. 2008; 9 :132. DOI: 10.1186/1471-2474-9-132 - 31.
Walter SS, Wintermeyer E, Klinger C, et al. Association between metabolic syndrome and hiposteoarthritis in middle-aged men and women from the general population. PLoS One. 2020; 15 (3):e0230185. DOI: 10.1371/journal.pone.0230185 - 32.
Messier SP, Beavers DP, Mihalko SL, et al. The effects of intensive dietary weight loss and exercise on gait in overweight and obese adults with knee osteoarthritis. The intensive diet and exercise for arthritis (IDEA) trial. Journal of Biomechanics. 2020; 98 :109477. DOI: 10.1016/j.jbiomech.2019.109477 - 33.
Messier SP, Mihalko SL, Legault C, et al. Effects of intensive diet and exercise on knee joint loads, inflammation, and clinical outcomes among overweight and obese adults with knee osteoarthritis: The IDEA randomized clinical trial. Journal of the American Medical Association. 2013; 310 (12):1263-1273. DOI: 10.1001/jama.2013.277669 - 34.
Godziuk K, Prado CM, Linda J, et al. The impact of sarcopenic obesity on knee and hip osteoarthritis: A scoping review. BMC Musculoskeletal Disorders. 2018; 19 :271. DOI: 10.1186/s12891-018-2175-7 - 35.
Hall KD, Kahan S. Maintenance of lost weight and long-term management of obesity. The Medical Clinics of North America. 2018; 102 (1):183-197. DOI: 10.1016/j.mcna.2017.08.012 - 36.
Purcell S, Thornberry R, Elliott SA, et al. Body composition, strength, and dietary intake of patients with hip or knee osteoarthritis. Canadian Journal of Dietetic Practice and Research. 2016; 77 (2):98-102. DOI: 10.3148/cjdpr-2015-037 - 37.
de Zwart AH, van der Leeden M, Roorda LD, et al. Dietary protein intake and upper leg muscle strength in subjects with knee osteoarthritis data from the osteoarthritis initiative. Rheumatology International. 2019; 39 (2):277-284. DOI: 10.1007/s00296-018-4223-x - 38.
Wang Y, Simpson JA, Wluka EA, et al. Meat consumption and risk of primary hip and knee joint replacement due to osteoarthritis: A prospective cohort study. BMC Musculoskeletal Disorders. 2011; 12 :17. DOI: 10.1007/s00296-018-4223-x - 39.
Comee L, Taylor CA, Nahikian-Nelms M, et al. Dietary patterns and nutrient intake of individuals with rheumatoid arthritis and osteoarthritis in the United States. Nutrition. 2019; 67-68 :110533. DOI: 10.1016/j.nut.2019.06.014 - 40.
Sadeghi A, Zarrinjooiee G, Mousavi SN, et al. Effects of a Mediterranean diet compared with the low-fat diet on patients with knee osteoarthritis: A randomized feeding trial. International Journal of Clinical Practice. 2022; 2022 :7275192. DOI: 10.1155/2022/7275192 - 41.
Xu CP, Li X, Song JQ , et al. Mini-incision versus standard incision Total hip arthroplasty regarding surgical outcomes: A systematic review and meta-analysis of randomized controlled trials. PLoS One. 2013; 8 (11):e80021. DOI: 10.1371/journal.pone.0080021 - 42.
Sicat CS, Muthusamy N, Singh V, et al. Impact of preoperative Anemia severity on primary Total hip arthroplasty outcomes. The Journal of Arthroplasty. 2022; 37 :721-726. DOI: 10.1016/j.arth.2021.12.042 - 43.
Maezawa K, Nozawa M, Yuasa T, et al. Postoperative hemoglobin and recovery of hip muscle strength after total hip arthroplasty. Journal of Orthopaedics. 2018; 15 :886-888. DOI: 10.1016/j.jor.2018.08.016 - 44.
Jans Ø, Bandholm T, Kurbegovic S, et al. Postoperative anemia and early functional outcomes after fast-track hip arthroplasty: A prospective cohort study. Transfusion. 2016; 56 (4):917-925. DOI: 10.1111/trf.13508 - 45.
Hardy EJO, Inns TB, Hatt J, et al. The time course of disuse muscle atrophy of the lower limb in health and disease. Journal of Cachexia, Sarcopenia and Muscle. 2022; 13 :2616-2629. DOI: 10.1002/jcsm.13067 - 46.
Kramer IF, Snijders T, Smeets JSJ, et al. Extensive type II muscle Fiber atrophy in elderly female hip fracture patients. The Journals of Gerontology. Series A, Biological Sciences and Medical Sciences. 2017; 72 (10):1369-1375. DOI: 10.1093/gerona/glw253 - 47.
Morrell AT, Daniel R, et al. Enhanced recovery after primary Total hip and knee arthroplasty: A systematic review. The Journal of Bone and Joint Surgery. American Volume. 2021; 103 :1938-1947. DOI: 10.2106/JBJS.20.02169 - 48.
Dreyer HC, Owen EC, Strycker LA, et al. Essential amino acid supplementation mitigates muscle atrophy after Total knee arthroplasty: A randomized, double-blind, placebo-controlled trial. The Journal of Bone and Joint Surgery. 2018:e0006. DOI: 10.2106/JBJS.OA.18.00006 - 49.
Fukumoto Y, Ohata K, Tsukagoshi R, et al. Changes in hip and knee muscle strength in patients following total hip arthroplasty. Journal of the Japanese Physical Therapy Association. 2013; 16 (1):22-27. DOI: 10.1298/jjpta.Vol16_002 - 50.
Klausmeier V, Lugade V, Jewett BA, et al. Is there faster recovery with an anterior or anterolateral THA? A pilot study. Clinical Orthopaedics and Related Research. 2010; 468 (2):533-541. DOI: 10.1007/s11999-009-1075-4 - 51.
Judd DL, Dennis DA, Thomas AC, et al. Muscle strength and functional recovery during the first year after THA. Clinical Orthopaedics and Related Research. 2014; 472 (2):654-664. DOI: 10.1007/s11999-013-3136-y - 52.
Sicard-Rosenbaum L, Light KE, Behrman AL. Gait, lower extremity strength, and self-assessed mobility after hip arthroplasty. The Journals of Gerontology. Series A, Biological Sciences and Medical Sciences. 2002; 57 (1):M47-M51. DOI: 10.1093/gerona/57.1.m47 - 53.
Ninomiya K, Hirakawa K, Ikeda T, et al. Patients 10 years after total hip arthroplasty have the deficits in functional performance, physical activity, and high fall rate compared to healthy adults. Physical Therapy Research. 2018; 21 (2):53-58. DOI: 10.1298/ptr.E9941 - 54.
Ismailidis P, Kvarda P, Vach W, et al. Abductor muscle strength deficit in patients after Total hip arthroplasty: A systematic review and meta-analysis. The Journal of Arthroplasty. 2021; 36 (8):3015-3027 - 55.
Rasch A, Byström AH, Dalén N, et al. Persisting muscle atrophy two years after replacement of the hip. Journal of Bone and Joint Surgery. British Volume (London). 2009; 91 (5):583-588 - 56.
Kawano T, Nankaku M, Murao M, et al. Recovery of muscle atrophy and fatty infiltration in patients with acetabular dysplasia after Total hip arthroplasty. Journal of the American Academy of Orthopaedic Surgeons. 2022; 30 (3):e317-e326 - 57.
Isshiki K, Jinno T, Aizawa J, et al. Asymmetry of the cross-sectional area of the gluteus Medius muscle persists eight years after Total hip arthroplasty for osteoarthritis of the hip. Progress in Rehabilitation Medicine. 2021; 25 (6):20210052. DOI: 10.2490/prm.20210052 - 58.
Müller M, Tohtz S, Dewey M, et al. Age-related appearance of muscle trauma in primary total hip arthroplasty and the benefit of a minimally invasive approach for patients older than 70 years. International Orthopaedics. 2011; 35 (2):165-171. DOI: 10.1007/s00264-010-1166-6 - 59.
Lalevée M, Curado J, Matsoukis J, et al. Comparative MRI assessment of three minimally invasive approaches in total hip arthroplasty. Orthopaedics & Traumatology, Surgery & Research. 2022; 108 (6):103354. DOI: 10.1016/j.otsr.2022.103354 - 60.
Rykov K, Meys TWGM, Knobben BAS, et al. MRI assessment of muscle damage after the posterolateral versus direct anterior approach for THA (Polada trial). A Randomized Controlled Trial, Journal of Arthroplasty. 2021; 36 (9):3248-3258.e1. DOI: 10.1016/j.arth.2021.05.00 - 61.
Chang KJ, Albright A, Testa EJ, et al. Sarcopenia is associated with an increased risk of postoperative complications following Total hip arthroplasty for osteoarthritis. Biology. 2023; 12 (2):295. DOI: 10.3390/biology12020295 - 62.
Koto S, Ikeda T, Inoue S, et al. Differences in preoperative function and outcome of patients with versus without sarcopenia after total hip arthroplasty. Journal of Physical Therapy Science. 2022; 34 (1):60-64. DOI: 10.1589/jpts.34.60 - 63.
Hida T, Ishiguro N, Shimokata H, et al. High prevalence of sarcopenia and reduced leg muscle mass in Japanese patients immediately after a hip fracture. Geriatrics & Gerontology International. 2013; 13 (1):413-420. DOI: 10.1111/j.1447-0594.2012.00918.x - 64.
Ueoka K, Kabata T, Kajino Y, et al. The prevalence and impact of sarcopenia in females undergoing total hip arthroplasty: A prospective study. Modern Rheumatology. 2022; 32 (1):193-198. DOI: 10.1080/14397595.2021.1899603 - 65.
Brown JP, Adachi JD, Schemitsch E, et al. Mortality in older adults following a fragility fracture: Real-world retrospective matched-cohort study in Ontario. BMC Musculoskeletal Disorders. 2021; 22 :105. DOI: 10.1186/s12891-021-03960-z - 66.
Johnson RL, Abdel MP, Frank RD, et al. Impact of frailty on outcomes after primary and revision Total hip arthroplasty. The Journal of Arthroplasty. 2019; 34 (1):56-64.e5. DOI: 10.1016/j.arth.2018.09.078 - 67.
Wen H, Liu T, Li J. Association between frailty and clinical post-operative outcomes in patients following hip arthroplasty: A systematic review and meta-analysis. International Orthopaedics. 2023; 47 (3):667-675. DOI: 10.1007/s00264-022-05657-x - 68.
Cook MJ, Lunt M, Ashcroft DM, et al. The impact of frailty on patient-reported outcomes following hip and knee arthroplasty. Age and Ageing. 2022; 51 (12):afac288. DOI: 10.1093/ageing/afac288 - 69.
Bai Y, Zhang XM, Sun X, et al. The association between frailty and mortality among lower limb arthroplasty patients: A systematic review and meta-analysis. BMC Geriatrics. 2022; 22 (1):702. DOI: 10.1186/s12877-022-03369-w - 70.
Tram MK, O’Connor CM, Qian AS, et al. Frailty is associated with increased 30-day adverse events and hospitalization costs after primary Total hip arthroplasty. The Journal of Arthroplasty. 2022; 37 (8S):S925-S930. DOI: 10.1016/j.arth.2022.01.047 - 71.
Oosting E, Jans MP, Dronkers JJ, et al. Preoperative home-based physical therapy versus usual care to improve functional health of frail older adults scheduled for elective total hip arthroplasty: A pilot randomized controlled trial. Archives of Physical Medicine and Rehabilitation. 2012; 93 (4):610-616. DOI: 10.1016/j.apmr.2011.11.006 - 72.
Hoogeboom TJ, Dronkers JJ, van den Ende CH, et al. Preoperative therapeutic exercise in frail elderly scheduled for total hip replacement: A randomized pilot trial. Clinical Rehabilitation. 2010; 24 (10):901-910. DOI: 10.1177/0269215510371427 - 73.
O’Brien MS, McDougall JJ. Age and frailty as risk factors for the development of osteoarthritis. Mechanisms of Ageing and Development. 2019; 0 :21-28. DOI: 10.1016/j.mad.2019.03.003 - 74.
Dent E, Morley JE, Cruz-Jentoft AJ, et al. International clinical practice guidelines for sarcopenia (ICFSR): Screening, diagnosis and management. The Journal of Nutrition, Health & Aging. 2018; 22 (10):1148-1161. DOI: 10.1007/s12603-018-1139-9 - 75.
Chen LK, Woo J, Assantachai P, et al. Asian working Group for Sarcopenia: 2019 consensus update on sarcopenia diagnosis and treatment. Journal of the American Medical Directors Association. 2020; 21 (3):300-307.e2. DOI: 10.1016/j.jamda.2019.12.012 - 76.
Sayer AA, Cruz-Jentoft A. Sarcopenia definition, diagnosis and treatment: Consensus is growing. Age and Ageing. 2022; 51 (10):afac220. DOI: 10.1093/ageing/afac220 - 77.
Bauer J, Morley JE, Schols AMWJ, et al. Sarcopenia: A time for action. An SCWD Position Paper. Journal of Cachexia, Sarcopenia and Muscle. 2019; 10 (5):956-961. DOI: 10.1002/jcsm.12483 - 78.
Beckwée D, Delaere A, Aelbrecht S, et al. Exercise interventions for the prevention and treatment of sarcopenia. A systematic umbrella review. The Journal of Nutrition, Health & Aging. 2019; 23 (6):494-502. DOI: 10.1007/s12603-019-1196-8 - 79.
Cruz-Jentoft AJ, Sayer AA. Sarcopenia. Lancet. 2019; 393 (10191):2636-2646. DOI: 10.1016/S0140-6736(19)31138-9 - 80.
Dent E, Lien C, Lim WS, et al. The Asia-Pacific clinical practice guidelines for the Management of Frailty. Journal of the American Medical Directors Association. 2017; 18 (7):564-575. DOI: 10.1016/j.jamda.2017.04.018 - 81.
Dent E, Morley JE, Cruz-Jentoft AJ, et al. Physical frailty: ICFSR international clinical practice guidelines for identification and management. The Journal of Nutrition, Health & Aging. 2019; 23 (9):771-787. DOI: 10.1007/s12603-019-1273-z - 82.
Daly RM, Iuliano S, Fyfe JJ, et al. Screening, diagnosis and Management of Sarcopenia and Frailty in hospitalized older adults: Recommendations from the Australian and new Zealand Society for Sarcopenia and Frailty Research (ANZSSFR) expert working group. The Journal of Nutrition, Health & Aging. 2022; 26 (6):637-651. DOI: 10.1007/s12603-022-1801-0 - 83.
Kehlet H, Wilmore DW. Evidence-based surgical care and the evolution of fast-track surgery. Annals of Surgery. 2008; 248 (2):189-198. DOI: 10.1097/SLA.0b013e31817f2c1a - 84.
Gaffney CJ, Pelt CE, Gililland JM, et al. Perioperative pain Management in hip and Knee Arthroplasty. The Orthopedic Clinics of North America. 2017; 48 (4):407-419. DOI: 10.1016/j.ocl.2017.05.001 - 85.
Zhang C, Xiao J. Application of fast-track surgery combined with a clinical nursing pathway in the rehabilitation of patients undergoing total hip arthroplasty. The Journal of International Medical Research. 2020; 48 (1):300060519889718 - 86.
Schneider M, Kawahara I, Ballantyne G, et al. Predictive factors influencing fast track rehabilitation following primary total hip and knee arthroplasty. Archives of Orthopaedic and Trauma Surgery. 2009; 129 (12):1585-1591. DOI: 10.1007/s00402-009-0825-9 - 87.
de Carvalho Almeida RF, Serra HO, de Oliveira LP. Fast-track versus conventional surgery in relation to time of hospital discharge following total hip arthroplasty: A single-center prospective study. Journal of Orthopaedic Surgery and Research. 2021; 16 (1):488. DOI: 10.1186/s13018-021-02640-x - 88.
Liao CD, Wu YT, Tsauo JY, et al. Effects of protein supplementation combined with exercise training on muscle mass and function in older adults with lower-extremity osteoarthritis: A systematic review and meta-analysis of randomized trials. Nutrients. 2020; 12 (8):2422. DOI: 10.3390/nu12082422 - 89.
Ikeda T, Jinno T, Masuda T, et al. Effect of exercise therapy combined with branched-chain amino acid supplementation on muscle strengthening in persons with osteoarthritis. Hong Kong Physiotherapy Journal. 2018; 38 (1):23-31. DOI: 10.1142/S1013702518500038 - 90.
Labata-Lezaun N, Llurda-Almuzara L, López-de-Celis C, et al. Effectiveness of protein supplementation combined with resistance training on muscle strength and physical performance in elderly: A systematic review and meta-analysis. Nutrients. 2020; 12 (9):2607. DOI: 10.3390/nu12092607 - 91.
Ten Haaf DSM, Nuijten MAH, Maessen MFH, et al. Effects of protein supplementation on lean body mass, muscle strength, and physical performance in nonfrail community-dwelling older adults: A systematic review and meta-analysis. The American Journal of Clinical Nutrition. 2018; 108 (5):1043-1059. DOI: 10.1093/ajcn/nqy192 - 92.
Skoffer B, Dalgas U, Mechlenburg I. Progressive resistance training before and after total hip and knee arthroplasty: A systematic review. Clinical Rehabilitation. 2015; 29 (1):14-29. DOI: 10.1177/0269215514537093 - 93.
Temporiti F, Draghici I, Fusi S, et al. Does walking the day of total hip arthroplasty speed up functional independence? A non-randomized controlled study. Archives of Physiotherapy. 2020; 24 (10):8. DOI: 10.1186/s40945-020-00079-7 - 94.
Büttner M, Mayer AM, Büchler B, et al. Economic analyses of fast-track total hip and knee arthroplasty: A systematic review. European Journal of Orthopaedic Surgery and Traumatology. 2020; 30 (1):67-74. DOI: 10.1007/s00590-019-02540-1 - 95.
Boyd CM, Landefeld CS, Counsell SR, et al. Recovery of activities of daily living in older adults after hospitalization for acute medical illness. Journal of the American Geriatrics Society. 2008; 56 (12):2171-2179. DOI: 10.1111/j.1532-5415.2008.02023.x - 96.
Weijzen MEG, Kouw IWK, Verschuren AAJ, et al. Protein intake falls below 0.6 g•kg-1•d-1 in healthy, older patients admitted for elective hip or knee arthroplasty. The Journal of Nutrition, Health & Aging. 2019; 23 (3):299-305. DOI: 10.1007/s12603-019-1157-2 - 97.
Ueyama H, Kanemoto N, Minoda Y, et al. Perioperative essential amino acid supplementation suppresses rectus femoris muscle atrophy and accelerates early functional recovery following total knee arthroplasty. The Bone & Joint Journal. 2020; 102-B :10-18. DOI: 10.1302/0301-620X.102B6.BJJ-2019-1370.R1 - 98.
Ninomiya K, Takahira N, Ikeda T, et al. Effects of perioperative exercise therapy combined with nutritional supplementation on functional recovery after fast-track total hip arthroplasty. Journal of Orthopaedic Science. DOI: 10.1016/j.jos.2022.09.012 - 99.
Ikeda T, Matsunaga Y, Kanbara M, et al. Effect of exercise therapy combined with branched-chain amino acid supplementation on muscle strength in elderly women after total hip arthroplasty: A randomized controlled trial. Asia Pacific Journal of Clinical Nutrition. 2019; 28 (4):720-726. DOI: 10.6133/apjcn.201912_28(4).0007 - 100.
Freeman S, Mascia A, McGill S. Arthrogenic neuromusculature inhibition: A foundational investigation of existence in the hip joint. Clinical Biomechanics (Bristol, Avon). 2013; 28 (2):171-177 - 101.
Lewis GN, Rice DA, McNair PJ, et al. Predictors of persistent pain after total knee arthroplasty: A systematic review and meta-analysis. British Journal of Anaesthesia. 2015; 114 (4):551-561. DOI: 10.1093/bja/aeu441 - 102.
Pua YH. The time course of knee swelling post Total knee arthroplasty and its associations with quadriceps strength and gait speed. The Journal of Arthroplasty. 2015; 30 (7):1215-1219 - 103.
Kerksick CM, Arent S, Schoenfeld BJ, et al. International society of sports nutrition position stand: Nutrient timing. Journal of the International Society of Sports Nutrition. 2017; 14 :33. DOI: 10.1186/s12970-017-0189-4 - 104.
Stokes T, Hector AJ, Morton RW, et al. Recent perspectives regarding the role of dietary protein for the promotion of muscle hypertrophy with resistance exercise training. Nutrients. 2018; 10 (2):180. DOI: 10.3390/nu10020180 - 105.
Ninomiya K, Takahira N, Ochiai S, et al. Incidence of postoperative complications and non- periprosthetic fractures after total hip arthroplasty: A more than 10-year follow-up retrospective cohort study. Physical Therapy Research. 2020; 24 (1):77-83. DOI: 10.1298/ptr.E10043 - 106.
Moyer R, Ikert K, Long K, et al. The value of preoperative exercise and education for patients undergoing Total hip and knee arthroplasty: A systematic review and meta-analysis. JBJS Reviews. 2017; 5 (12):e2. DOI: 10.2106/JBJS.RVW.17.00015 - 107.
Saueressig T, Owen PJ, Zebisch J, et al. Evaluation of exercise interventions and outcomes after hip arthroplasty: A systematic review and meta-analysis. JAMA Network Open. 2021; 4 (2):e210254. DOI: 10.1001/jamanetworkopen.2021.0254 - 108.
Austin MS, Urbani BT, Fleischman AN, et al. Formal physical therapy after Total hip arthroplasty is not required: A randomized controlled trial. The Journal of Bone and Joint Surgery. American Volume. 2017; 99 (8):648-655. DOI: 10.2106/JBJS.16.00674 - 109.
Ikeda T, Sato R, Ninomiya K, et al. Nutritional factors related to muscle weakness 1 year after Total hip arthroplasty: A case-control study. Annals of Nutrition & Metabolism. 2022; 78 (2):73-79. DOI: 10.1159/000520551 - 110.
Jinno T, Ikeda T. Update of exercise therapy for osteoarthritis of the hip and total hip arthroplasty. Orthopaedic Surgery and Traumatology. 2021:427-433. DOI: 10.18888/se.0000001681