The 12 principles of green synthesis are fulfilled with the biological approach to produce nanoparticles.
Abstract
Nanoparticles are often associated with their small size and numerous applications. However, the synthesis process is equally important as it determines the size and properties of the nanoparticles. While traditional nanoparticle synthesis methods require the use of hazardous chemicals and high-energy consumption, green synthesis offers a sustainable, cost-effective, and environmentally friendly alternative. This approach utilizes natural resources and biologically active compounds that can act as reducing, stabilizing, or capping agents in the one-step synthesis of nanoparticles. Green synthesis offers numerous advantages, including the development of processes with minimal environmental impact and improved safety for nanoparticle synthesis. Overall, the synthesis of nanoparticles using green chemistry is a promising approach for sustainable and efficient production. This chapter provides a general overview of nanoparticles, their applications, and green synthesis, and highlights the various biological resources used in these processes and the factors affecting their synthesis.
Keywords
- green synthesis
- nanoparticles
- plant extract
- microorganisms
- phytochemicals
1. Introduction
Nanoparticles are small particles with sizes ranging from 1 to 100 nanometers. These materials have gained importance and interest in recent years owing to their large number of applications, because the matter at this scale presents a more compact arrangement of atoms and molecules, generating phenomena and acquiring or enhancing mechanical [1], electrical [2], magnetic [3], optical [4], catalytic [5], and antibacterial [6, 7] properties that are completely different from those of their macroscopic counterparts [8]. They can be classified based on their composition, shape, and size. The most common types of nanoparticles are metals, metal oxides, carbon-based, and quantum dots. Owing to their unique sizes and properties, nanoparticles have attracted significant attention in various fields including medicine, electronics, energy, and environmental science [9, 10]. By reducing their size, nanoparticles can have a higher surface-to-volume ratio, enabling a greater number of atoms or molecules per volume, which means that less material is needed to obtain the same activity and exhibit other properties (Figure 1) [11].
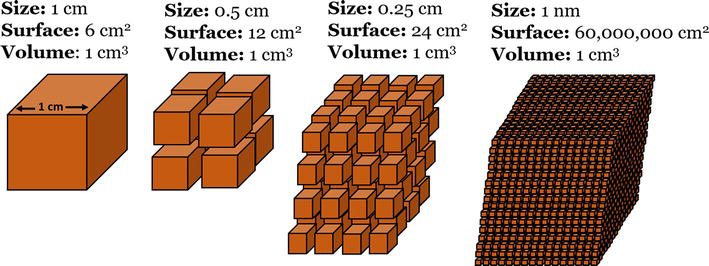
Figure 1.
Surface-to-volume ratio of nanoparticles compared with that of bulk materials.
Nanoparticles have many potential benefits for the environment. For example, nanoparticles can be used to improve the efficiency of water treatment, air filtration, and soil remediation; reduce pollution, and develop new types of renewable energy technologies [12]. In medicine, nanoparticles have shown potential for drug delivery, imaging, and cancer therapy. They can be functionalized with targeting moieties, making them capable of selectively targeting cancer cells, while sparing normal cells. Additionally, nanoparticles can enhance the efficacy of chemotherapy by improving drug delivery to the tumor site and reducing systemic toxicity [13]. In electronics, nanoparticles are used to fabricate high-performance devices such as sensors, transistors, and solar cells [14]. Nanoparticles have potential applications in fuel cells, hydrogen storage, and catalysis [15].
However, it is also important to address the environmental impact of the nanoparticles. Some studies have shown that nanoparticles can harm plants, animals, and humans, but it depends on many factors, such as concentration, size, and time of exposure [16, 17]. Nanoparticles can easily be released into the environment through various sources, such as industrial emissions, consumer products, and medical procedures. Once released into the environment, nanoparticles can be difficult to control and monitor. There is potential for long-term accumulation. Nanoparticles can accumulate in the environment, and they may be able to persist for long periods. This raises concerns about the potential for nanoparticles to cause long-term harm to the environment and human health [17, 18]. However, one of the alternatives for reducing their environmental impact is to control the synthesis process.
There are many methods for synthesizing nanoparticles, including physical, chemical, and biological processes [19]. Green synthesis, which refers to the eco-friendly and sustainable production of nanoparticles without the use of hazardous chemicals or toxic solvents, has gained attention in recent years within biological processes. Natural sources, such as plants and microorganisms, are popular green synthesis approaches [20]. This method has several advantages over traditional synthesis methods, including low cost, scalability, and reduction of hazardous waste. Moreover, green synthesis can produce nanoparticles with unique shapes, sizes, and surface properties tailored for specific applications [21]. The biological sources used for the green synthesis of nanoparticles contain biologically active compounds, such as enzymes, proteins, polyphenols, flavonoids, and terpenoids, which can act as catalyzing, reducing, stabilizing, or capping agents for one-step synthesis [20, 21].
In summary, this chapter provides a general overview of nanoparticles, their properties and applications, and how green synthesis is used to synthesize them. This chapter also discusses the different biological resources used for green synthesis, the factors that participate, and the mechanisms involved in their production.
2. Traditional nanoparticle synthesis methods
There are many methods for synthesizing nanoparticles (Figure 2). Some of the most common methods include the following.
Chemical reduction: This method involves the reduction of metal ions in solution using chemical reagents such as sodium borohydride or sodium hydroxide to form nanoparticles [22].
Coprecipitation: Synthesis involves mixing two or more solutions containing metal ions. When the solutions are mixed, metal ions precipitate out of the solution and form nanoparticles [23].
Sol-gel: The process requires mixing a metal salt with a solvent and gelling agent. The solvent is evaporated leaving behind the gel. The gel is then heated, causing it to solidify and form nanoparticles [24].
Microemulsion: This method needs surfactants, water-soluble compounds, and oil-soluble compounds. The mixture forms small droplets that contain the metal ions. When droplets are heated, metal ions precipitate out of the solution and form nanoparticles [25].
Solvothermal/hydrothermal synthesis: This reaction involves heating a solution of metal ions in water or an organic solvent under high pressure. High pressure and temperature cause metal ions to precipitate out of the solution and form nanoparticles [26].
Sonochemical/electrochemical synthesis: This process uses ultrasound or an electrical current to break down metal salts into nanoparticles [27].
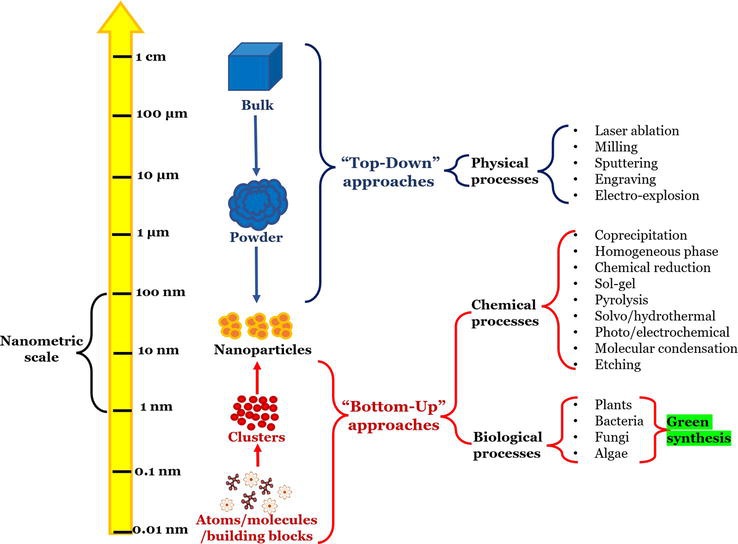
Figure 2.
Nanometric scale and different approaches to nanoparticle synthesis.
In addition, there are physical processes, such as laser ablation, milling, and sputtering, where the material is reduced to nanoparticles by the mechanical action of the equipment used [28].
The choice of method depends on the type of nanoparticles being synthesized, the desired size and shape, and the availability of equipment and reagents.
2.1 Environmental limitations in nanoparticle synthesis
Traditional methods for synthesizing nanoparticles have several limitations.
Using organic reagents can harm the environment, humans, and animals, causing illnesses, such as liver damage [18]. In addition, wastewater generated from nanoparticle synthesis can contain harmful chemicals [29].
The low yield is another disadvantage: only a small percentage of the starting materials is converted into nanoparticles, generating raw material waste. The high cost of the starting materials, equipment, labor required, long-time synthesis, and the inability to control the size and shape can limit their applications [30, 31].
2.2 Strategies to overcome barriers to nanoparticle synthesis
Several strategies can be used to overcome the disadvantages of nanoparticle synthesis, such as the use of environmentally friendly solvents, reagents, and processes. Using water, ionic liquids, and supercritical fluids are examples of eco-friendly solvents [21, 32] or we can even perform solvent-free synthesis, eliminating the need for hazardous chemicals and reducing the environmental impact of nanoparticle synthesis [33].
Many nanoparticle synthesis methods are not scalable, which limits their application. Therefore, it is necessary to develop cost-effective and efficient processes to obtain large quantities of nanoparticles [8].
Multipurpose nanoparticles can be used to improve their performance in a variety of applications and fields. For example, biocompatible nanoparticles are used in biomedicine or as stable nanoparticles for long-term applications [34].
The characterization of nanoparticles is important for understanding their size, shape, surface properties, and chemical composition. This information can be used to understand how nanoparticles interact with their environment and ensure they are safe [35].
Strategies to overcome these barriers in nanoparticle synthesis are still under study to develop more innovative, efficient, cost-effective, and environmentally friendly methods.
3. Green synthesis of nanoparticles: an overview
Green synthesis aims to promote innovative chemical technologies to reduce or eliminate the use and production of hazardous substances in the design, manufacture, and use of chemical products. This involves minimizing or, if possible, eliminating the pollution produced in the synthesis processes, avoiding the consumption and wastage of nonrenewable raw materials, using hazardous or polluting materials in product manufacturing, and reducing the synthesis time. Paul J. Anastas, considered the father of green chemistry, defined it as “a work philosophy that involves the use of alternative tools and pathways to prevent pollution,” referring to both the design of the synthetic strategy and the treatment of possible secondary products originating from that route [36, 37].
Two approaches can be used to generate nanoparticles [37, 38] (Figure 2).
“Top-down” approach: In which nanoparticles are produced using physical techniques such as grinding or abrasion of a material.
“Bottom-up” approach: Where nanoparticles are generated from “building blocks” of atoms or molecules, resulting in more complex assemblies. Three alternatives are identified using this approach.
Chemical synthesis: The method of producing molecules or particles by the reaction of substances used as raw materials.
Self-assembly: A technique in which atoms or molecules self-order through physical and/or chemical interactions.
Positional assembly: The atoms, molecules, and aggregates are deliberately manipulated and positioned individually. However, this method is extremely laborious and unsuitable for industrial applications.
The “bottom-up” approach is preferred over the “top-down” approach because specialized equipment is not required and the time to obtain nanoparticles is shorter. Green synthesis is gaining relevance in producing nanoparticles within the “bottom-up” approach [37].
The use of plant species, algae, or microorganisms such as bacteria or fungi is one of the most commonly used resources for this procedure. Various compounds from plants or microorganisms, including terpenes, polyphenols, alkaloids, carbohydrates, proteins, and genetic materials, play an important role in the synthesis of nanoparticles by acting together [39, 40].
In addition to the biological resources used to perform the synthesis (plants, algae, or microorganisms), other factors influence the shape and size of nanoparticles, such as the concentration of the metal ion, pH, reaction time, and temperature [39, 41].
In general, the phases for the green synthesis of nanoparticles include (Figure 3) [39].
Initial phase: Obtaining the reaction medium, which is the aqueous extract of one or several parts of the plant species or the culture media for the growth of microorganisms, in addition to the precursor salt, which is the source of metal ions.
Activation phase: Chemical reduction of metal ions and generation of nucleation centers occur where nanoparticles emerge and grow.
Growth phase: Small adjacent nanoparticles spontaneously fuse into larger particles, forming aggregates, which are influenced by factors such as temperature, concentration, and type of compounds, pH, and reaction time.
Termination phase: The final shape of the nanoparticles is determined, and the compounds that participate in the reaction help stabilize and enhance their properties.
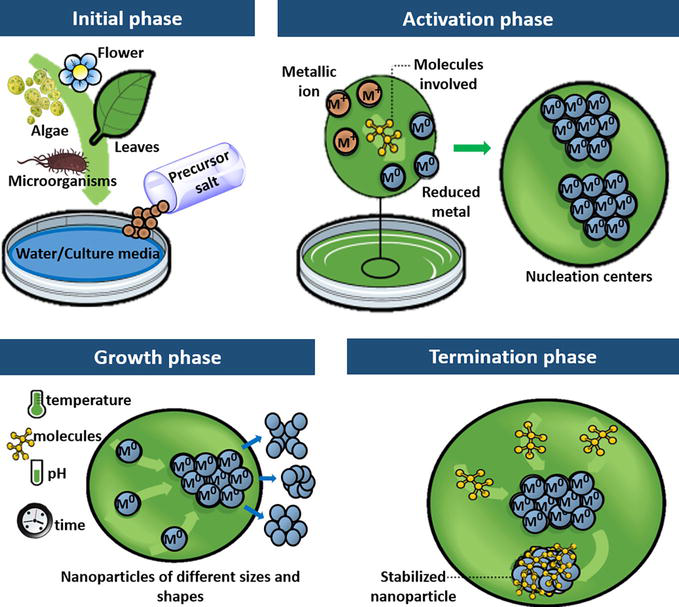
Figure 3.
Phases involved in the green synthesis of nanoparticles.
3.1 Biological resources for the green synthesis of nanoparticles
As stated previously, nanoparticles have attracted attention in the fields of biology, medicine, and electronics in recent years, owing to their remarkable applications (Figure 4). Numerous nanoparticle synthesis techniques have been developed; however, these may involve the use of toxic compounds and high-energy physical processes. An alternative is the use of biological methods to circumvent these obstacles. Bacteria, fungi, algae, and plant species are some of the most commonly used biological resources for the green synthesis of nanoparticles (Figure 4). This biological approach has provided a method that is reliable, straightforward, benign, and environmentally beneficial [40, 42].
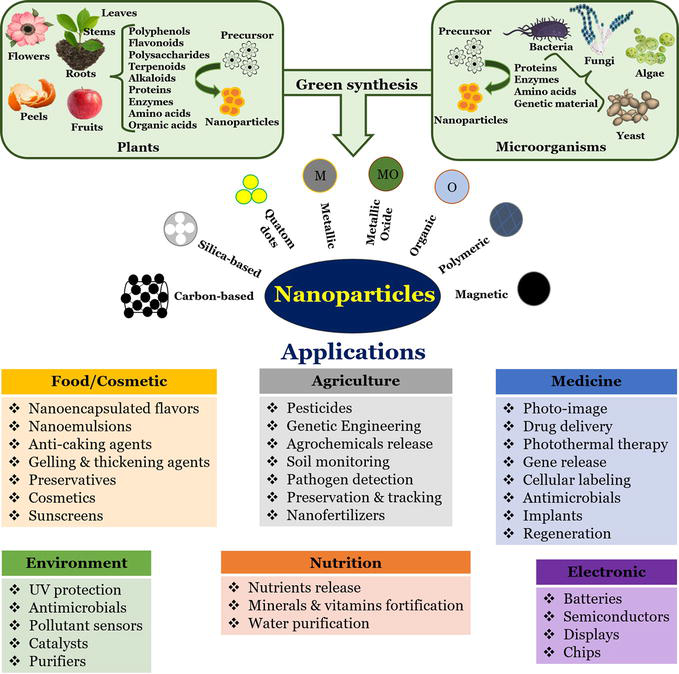
Figure 4.
Biological resources and compounds used for the green synthesis of nanoparticles and some of their applications [
3.1.1 Bacteria
Nanoparticle synthesis using bacteria is performed both extracellularly and intracellularly [38].
Intracellular: The synthesis is carried out inside the living microorganism, using its growth conditions to favor synthesis, known as “nanoparticle micro-factories.” To recover nanoparticles, bacteria must be destroyed [43].
Extracellular: The components released by the bacteria after lysis are used. The synthesis is performed by adding a metal salt precursor to the medium in which these components are located. Extracellular synthesis has the advantage of being faster because it does not require additional steps to recover nanoparticles from microorganisms [43, 44].
Enzymes, such as reductases, which catalyze the reduction of metal ions into nanoparticles, participate in the synthesis. Even components of the genetic material participate in this process [45, 46].
3.1.2 Fungi
Fungi contain active biomolecules, such as proteins or enzymes, that participate in nanoparticle synthesis, improving their yields and stability [47].
Some fungal species can synthesize nanoparticles using extracellular amino acids. For example, glutamic and aspartic acids on the surface of yeast or the reductase enzyme in the cytosol of fungi reduce metal ions to form nanoparticles. This is facilitated by the presence of hydroxyl groups in the mycelium, which donate electrons to the metal ion and reduce it to form nanoparticles. Aliphatic and aromatic amines or some proteins act as coating agents to stabilize them [48, 49].
3.1.3 Algae
Algae are used in nanotechnology because of their low toxicity and their ability to bioaccumulate and reduce metals [50].
Nanoparticle synthesis can be intracellular, with the metal ion entering the alga, or extracellular, and involves compounds such as polysaccharides, proteins, and pigments that direct the reduction of metal ions and coat the newly formed nanoparticles. These particles are subsequently released from the cell in the form of colloids [51].
3.1.4 Plant species
The use of plants in nanoparticle synthesis is one of the most widely used methods because of its environmentally friendly nature, as it avoids the use of toxic or harmful substances. It is also one of the fastest and most economical methods because it involves fewer steps [39, 40]. This makes it highly efficient in the nanoparticle production process compared to synthesis using microorganisms.
Plants contain several compounds (terpenes, flavonoids, polyphenols, alkaloids, proteins, etc.) that reduce metal ions and stabilize the resulting nanoparticles [52].
This type of synthesis can be performed using intracellular, extracellular, and phytochemical-mediated methods [53].
Intracellular: The synthesis is carried out inside the plant cell, and the nanoparticles are recovered by breaking down the structure, which is very similar to the intracellular method using microorganisms. Control of the growth factors of plant species is required so that they do not interfere with synthesis [54].
Extracellular: This method is the most commonly used because of its ease and speed. The process begins by obtaining a plant extract, which is generally water-based, to which a metal salt precursor is added. Owing to the action of the different compounds present in the extract, nanoparticles are generated and stabilized in a single step [54, 55].
Phytochemically mediated: This is based on the extracellular method, but with the difference that isolated phytochemical compounds are used and other substances are added to stabilize the nanoparticles. There is greater control over the synthesis, but more components and steps are involved [53].
3.2 Factors involved in the green synthesis of nanoparticles
As in any synthesis process, reaction conditions, such as temperature, pH, and reaction time, play an important role in the shape, size, and yield of the synthesized nanoparticles [39, 40, 41] (Figure 3).
Temperature: This is one of the most influential factors, as the shape (spherical, prismatic, flakes, triangular, octahedral, etc.), size, and synthesis depend on temperature. As the temperature increases, the reaction rate and the formation of nucleation centers increase, resulting in higher yields. Different temperatures promote different interactions between the reactants, giving rise to various shapes; the larger the temperature increase, the larger the size of the nanoparticles [56, 57].
pH: This influences the nucleation centers, generating more centers at higher pH values. Another important influence of pH is that some nanoparticles can only be synthesized in acidic or alkaline media. For example, magnetic nanoparticles are synthesized at an alkaline pH, and metal oxide nanoparticles are generally synthesized at an acidic or neutral pH [58].
Time: This parameter plays an important role in defining the size of the nanoparticles. It has been observed that longer reaction times favor an increase in the size of the nanoparticles and higher yields, owing to the prolonged interaction time between reactants [59].
3.3 The mechanism involved in the green synthesis of nanoparticles
The plant extract or organism used for the synthesis is an important factor that influences the morphology and size of nanoparticles because different concentrations of metabolites or cellular components give rise to differences in the nanoparticles [40, 60] (Figure 5).
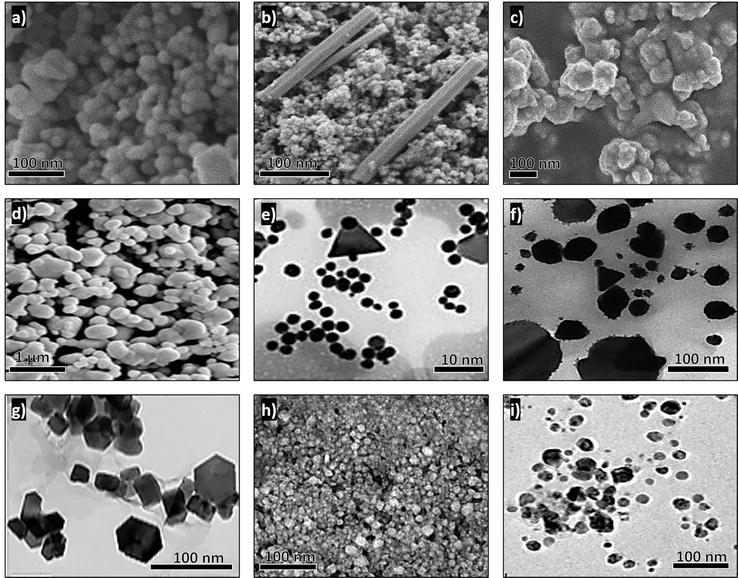
Figure 5.
Green-synthesized nanoparticles. (a) Spherical ZnO nanoparticles using the leaves of
Proteins and enzymes facilitate the formation of nanoparticles from metal ions. Because of their high reducing activity, proteins and enzymes can attract metal ions to specific regions of a molecule responsible for reduction, facilitating the formation of nanoparticles; however, their chelating activity is not excessive. The amino acids of a protein can greatly influence the size, morphology, and quantity of nanoparticles generated, thus playing a very important role in determining their shape and yield. Removing a proton from amino acids or other molecules results in the formation of resonant structures capable of further oxidation. This process is accompanied by the active reduction of metal ions followed by the formation of nanoparticles [39].
Flavonoids are a large group of polyphenolic compounds that can actively chelate and reduce metal ions because they contain multiple functional groups capable of forming these structures. Structural transformations of flavonoids also generate protons that reduce metal ions to form nanoparticles; therefore, they are involved in the nucleation stage, their formation, and further aggregation. Saccharides can also play a role in nanoparticle formation. Monosaccharides, such as glucose, can act as reducing agents, as the aldehyde group of the sugar is oxidized to a carboxyl group through the addition of hydroxyl groups, which in turn leads to the reduction of metal ions and the synthesis of nanoparticles [39].
The mechanism of green synthesis of nanoparticles has been associated with the action of polyphenols, which act as ligands. Metal ions form coordination compounds, in which the fundamental structural unit is the central metal ion surrounded by coordinated groups arranged spatially at the corners of a regular tetrahedron. The aromatic hydroxyl groups in polyphenols bind to metal ions and form stable coordinated complexes. This system undergoes direct decomposition at high temperatures, releasing nanoparticles from the complex system [65].
Flavonoids, amino acids, proteins, terpenoids, tannins, and reducing sugars have hydroxyl groups that surround the metal ions to form complexes. After this process, the hydroxyl ions are oxidized to carbonyl groups, which stabilize the nanoparticles. Synthesis is favored if the participating molecules have at least two hydroxyl groups at the ortho- and para-positions [52, 65].
Amino acids influence the size, morphology, and yield of nanoparticles generated [23], depending on the specific amino acids present in the extract and their concentration, along with the reaction conditions that give rise to nanoparticles with different shapes [65].
4. Confirming that the biological approach of nanoparticle synthesis is a green chemistry method
To corroborate that the processes of nanoparticle synthesis using biological resources are “green synthesis methods,” the 12 principles mentioned above are revisited [66, 67, 68] (Table 1).
Table 1.
Considering the above, the 12 principles of green synthesis are fulfilled using biological resources, such as plants, bacteria, fungi, and algae, to synthesize nanoparticles [69, 70, 71].
Finally, green synthesis of nanoparticles is a sustainable and environmentally friendly alternative to traditional methods of nanoparticle synthesis. Traditional methods often take long periods of time, use toxic chemicals and solvents, or generate waste products that can pollute the environment and pose health risks to humans and animals. In contrast, the green synthesis method uses renewable natural resources, such as plant extracts and microorganisms, which are less damaging and can be replenished over time. In addition, these methods are often more cost-effective and faster than traditional procedures because they do not require expensive chemicals or equipment and are considered one-step syntheses, which contribute to energy savings [72].
In furtherance of these advantages, green synthesis methods are still being developed to improve their efficiency and scalability, leading to the potential benefits of green synthesis of nanoparticles or even their application to the synthesis of other molecules as drugs or nutraceuticals.
5. Conclusion
Nanoparticles have emerged as a versatile and promising class of materials with unique properties that can be harnessed for various applications. The use of green synthesis utilizing natural resources and biologically active compounds to produce nanoparticles is an area of continuous research to improve processes, reduce environmental damage, and meet the increasing demand for the application of these nanostructures. Utilizing biological resources, the synthesis of nanoparticles is inexpensive, faster, and considered a one-step synthesis while preserving or even improving the physical and chemical properties of the nanoparticles. With the great potential of this method and the sustainable and efficient production of nanoparticles, different sizes and shapes can be obtained, which makes it a very attractive option not only for the synthesis of nanostructures, but also for the application of this technique in the synthesis of other compounds.
Acknowledgments
The authors acknowledge Dr. Samuel Tehuacanero Cuapa, Physicist. Roberto Hernández Reyes, and Arq. Diego Quiterio Vargas for their technical support.
Thanks to the Consejo Nacional de Humanidades, Ciencias y Tecnologías (CONAHCYT) for the scholarship granted to Rafael Álvarez-Chimal with the CVU number: 579637.
Funding was provided by the
References
- 1.
Wu Q , Shou MW, Du ZY, Jun GH, Hui D. Mechanical properties of nanomaterials: A review. Nanotechnology Reviews. 2020; 9 (1):259-273. DOI: 10.1515/ntrev-2020-0021 - 2.
Min SH, Lee TH, Lee S, Song JH, Lee GY, Zontar D, et al. Simulation of electrical conductivity for nanoparticles and nanotubes composite sensor according to geometrical properties of nanomaterials. Composites Part B: Engineering. 2019; 174 :107003. DOI: 10.1016/j.compositesb.2019.107003 - 3.
Martínez-Mera I, Espinosa-Pesqueira ME, Pérez-Hernández R, Arenas-Alatorre J. Synthesis of magnetite (Fe3O4) nanoparticles without surfactants at room temperature. Materials Letters. 2007; 61 (23-24):4447-4451. DOI: 10.1016/j.matlet.2007.02.018 - 4.
Kelly KL, Coronado E, Zhao LL, Schatz GC. The optical properties of metal nanoparticles: The influence of size, shape, and dielectric environment. The Journal of Physical Chemistry. B. 2003; 107 (3):668-677. DOI: 10.1021/jp026731y - 5.
Zeng J, Zhang Q , Chen J, Xia Y. A comparison study of the catalytic properties of Au-based nanocages, nanoboxes, and nanoparticles. Nano Letters. 2010; 10 (1):30-35. DOI: 10.1021/nl903062e - 6.
Álvarez-Chimal R, García-Pérez VI, Álvarez-Pérez MA, Arenas-Alatorre JÁ. Green synthesis of ZnO nanoparticles using a Dysphania ambrosioides extract. Structural characterization and antibacterial properties. Materials Science and Engineering: C. 2021;118 :111540. DOI: 10.1016/j.msec.2020.111540 - 7.
Reyes-Carmona L, Camps E, Campos-González E, Mercado-Celis G, Cervantes-Garduño A, Pérez-Ibarra EA, et al. Antimicrobial evaluation of bismuth subsalicylate nanoparticles synthesized by laser ablation against clinical oral microorganisms. Optics and Laser Technology. 2023; 158 :108930. DOI: 10.1016/j.optlastec.2022.108930 - 8.
Baig N, Kammakakam I, Falath W. Nanomaterials: A review of synthesis methods, properties, recent progress, and challenges. Materials Advances. 2021; 2 (6):1821-1871. DOI: 10.1039/D0MA00807A - 9.
Chaudhry N, Dwivedi S, Chaudhry V, Singh A, Saquib Q , Azam A, et al. Bio-inspired nanomaterials in agriculture and food: Current status, foreseen applications and challenges. Microbial Pathogenesis. 2018; 123 :196-200. DOI: 10.1016/j.micpath.2018.07.013 - 10.
Kolahalam LA, Kasi Viswanath IV, Diwakar BS, Govindh B, Reddy V, Murthy YLN. Review on nanomaterials: Synthesis and applications. Materials Today: Proceedings. 2019; 18 :2182-2190. DOI: 10.1016/j.matpr.2019.07.371 - 11.
Khan I, Saeed K, Khan I. Nanoparticles: Properties, applications and toxicities. Arabian Journal of Chemistry. 2019; 12 (7):908-931. DOI: 10.1016/j.arabjc.2017.05.011 - 12.
Lu AH, Salabas EL, Schüth F. Magnetic nanoparticles: Synthesis, protection, functionalization, and application. Angewandte Chemie, International Edition. 2007; 46 (8):1222-1244. DOI: 10.1002/anie.200602866 - 13.
Zhang L, Gu F, Chan J, Wang A, Langer R, Farokhzad O. Nanoparticles in medicine: Therapeutic applications and developments. Clinical Pharmacology and Therapeutics. 2008; 83 (5):761-769. DOI: 10.1038/sj.clpt.6100400 - 14.
Gao J, Gu H, Xu B. Multifunctional magnetic nanoparticles: Design, synthesis, and biomedical applications. Accounts of Chemical Research. 2009; 42 (8):1097-1107. DOI: 10.1021/ar9000026 - 15.
Singh R, Altaee A, Gautam S. Nanomaterials in the advancement of hydrogen energy storage. Heliyon. 2020; 6 (7):e04487. DOI: 10.1016/j.heliyon.2020.e04487 - 16.
Yao D, Chen Z, Zhao K, Yang Q , Zhang W. Limitation and challenge faced to the researches on environmental risk of nanotechnology. Procedia Environmental Sciences. 2013; 18 :149-156. DOI: 10.1016/j.proenv.2013.04.020 - 17.
Wilson N. Nanoparticles: Environmental problems or problem solvers? Bioscience. 2018; 68 (4):241-246. DOI: 10.1093/biosci/biy015 - 18.
Ray PC, Yu H, Fu PP. Toxicity and environmental risks of nanomaterials: Challenges and future needs. Journal of Environmental Science and Health, Part C. 2009; 27 (1):1-35. DOI: 10.108010590500802708267/ - 19.
Iravani S, Korbekandi H, Mirmohammadi SV, Zolfaghari B. Synthesis of silver nanoparticles: Chemical, physical and biological methods. Research in Pharmaceutical Sciences. 2014; 9 (6):385-406 - 20.
Mustapha T, Misni N, Ithnin NR, Daskum AM, Unyah NZ. A review on plants and microorganisms mediated synthesis of silver nanoparticles, role of plants metabolites and applications. International Journal of Environmental Research and Public Health. 2022; 19 (2):674. DOI: 10.3390/ijerph19020674 - 21.
Shafey AME. Green synthesis of metal and metal oxide nanoparticles from plant leaf extracts and their applications: A review. Green Processing and Synthesis. 2020; 9 (1):304-339. DOI: 10.1515/gps-2020-0031 - 22.
Manikam VR, Cheong KY, Razak KA. Chemical reduction methods for synthesizing Ag and Al nanoparticles and their respective nanoalloys. Materials Science and Engineering B. 2011; 176 (3):187-203. DOI: 10.1016/j.mseb.2010.11.006 - 23.
Nam NH, Luong NH. Nanoparticles: Synthesis and applications. In: Materials for Biomedical Engineering. Amsterdam, Netherlands: Elsevier; 2019. pp. 211-240. DOI: 10.1016/B978-0-08-102814-8.00008-1 - 24.
Bokov D, Turki Jalil A, Chupradit S, Suksatan W, Javed Ansari M, Shewael IH, et al. Nanomaterial by sol-gel method: Synthesis and application. Advances in Materials Science and Engineering. 2021; 2021 :1-21. DOI: 10.1155/2021/5102014 - 25.
Cid A. Synthesis of NPs by microemulsion method. In: Microemulsion - A Chemical Nanoreactor. London, UK: IntechOpen; 2018. DOI: 10.5772/intechopen.80633 - 26.
Ndlwana L, Raleie N, Dimpe KM, Ogutu HF, Oseghe EO, Motsa MM, et al. Sustainable hydrothermal and solvothermal synthesis of advanced carbon materials in multidimensional applications: A review. Materials. 2021; 14 (17):5094. DOI: 10.3390/ma14175094 - 27.
Ashassi-Sorkhabi H, Rezaei-moghadam B, Bagheri R, Abdoli L, Asghari E. Synthesis of Au nanoparticles by thermal, sonochemical and electrochemical methods: Optimization and characterization. Polymerase Chain Reaction. 2015; 3 (1):24-34. DOI: 10.22036/pcr.2015.7311 - 28.
Krishnia L, Thakur P, Thakur A. Synthesis of Nanoparticles by Physical Route. Synthesis and Applications of Nanoparticles. Singapore: Springer Nature Singapore; 2022. pp. 45-59. DOI: 10.1007/978-981-16-6819-7_3 - 29.
Khoshnamvand M, Hao Z, Fadare OO, Hanachi P, Chen Y, Liu J. Toxicity of biosynthesized silver nanoparticles to aquatic organisms of different trophic levels. Chemosphere. 2020; 258 :127346. DOI: 10.1016/j.chemosphere.2020.127346 - 30.
Rahimi HR, Doostmohammadi M. Nanoparticle synthesis, applications, and toxicity. In: Applications of Nanobiotechnology. London, UK: IntechOpen; 2020. DOI: 10.5772/intechopen.87973 - 31.
Jamkhande PG, Ghule NW, Bamer AH, Kalaskar MG. Metal nanoparticles synthesis: An overview on methods of preparation, advantages and disadvantages, and applications. Journal of Drug Delivery Science and Technology. 2019; 53 :101174. DOI: 10.1016/j.jddst.2019.101174 - 32.
Ying S, Guan Z, Ofoegbu PC, Clubb P, Rico C, He F, et al. Green synthesis of nanoparticles: Current developments and limitations. Environmental Technology and Innovation. 2022; 26 :102336. DOI: 10.1016/j.eti.2022.102336 - 33.
Landge S, Ghosh D, Aiken K. Solvent-Free Synthesis of Nanoparticles, Green Chemistry. Amsterdam, Netherlands: Elsevier; 2018. pp. 609-646. DOI: 10.1016/B978-0-12-809270-5.00022-4 - 34.
Kim D, Shin K, Kwon SG, Hyeon T. Synthesis and biomedical applications of multifunctional nanoparticles. Advanced Materials. 2018; 30 (49):1802309. DOI: 10.1002/adma.201802309 - 35.
Halamoda-Kenzaoui B, Vandebriel RJ, Howarth A, Siccardi M, David CAW, Liptrott NJ, et al. Methodological needs in the quality and safety characterisation of nanotechnology-based health products: Priorities for method development and standardisation. Journal of Controlled Release. 2021; 336 :192-206. DOI: 10.1016/j.jconrel.2021.06.016 - 36.
Anastas PT, Warner JC. Green Chemistry: Theory and Practice. Oxford [England], New York: Oxford University Press; 1998. p. 135 - 37.
Singh J, Dutta T, Kim KH, Rawat M, Samddar P, Kumar P. ‘Green’ synthesis of metals and their oxide nanoparticles: Applications for environmental remediation. Journal of Nanbiotechnology. 2018; 16 (1):84. DOI: 10.1186/s12951-018-0408-4 - 38.
Singh A, Gautam PK, Verma A, Singh V, Shivapriya PM, Shivalkar S, et al. Green synthesis of metallic nanoparticles as effective alternatives to treat antibiotics resistant bacterial infections: A review. Biotechnology Reports. 2020; 25 :e00427. DOI: 10.1016/j.btre.2020.e00427 - 39.
Makarov VV, Love AJ, Sinitsyna OV, Makarova SS, Yaminsky IV, Taliansky ME, et al. “Green” nanotechnologies: Synthesis of metal nanoparticles using plants. Acta Naturae. 2014; 6 (1):35-44. DOI: 10.32607/20758251-2014-6-1-35-44 - 40.
Hebbalalu D, Lalley J, Nadagouda MN, Varma RS. Greener techniques for the synthesis of silver nanoparticles using plant extracts, enzymes, bacteria, biodegradable polymers, and microwaves. ACS Sustainable Chemistry & Engineering. 2013; 1 (7):703-712. DOI: 10.1021/sc4000362 - 41.
Agarwal H, Venkat Kumar S, Rajeshkumar S. A review on green synthesis of zinc oxide nanoparticles – An eco-friendly approach. Resource-Efficient Technologies. 2017; 3 (4):406-413. DOI: 10.1016/j.reffit.2017.03.002 - 42.
Velusamy P, Kumar GV, Jeyanthi V, Das J, Pachaiappan R. Bio-inspired green nanoparticles: Synthesis, mechanism, and antibacterial application. Toxicological Research. 2016; 32 (2):95-102. DOI: 10.5487/TR.2016.32.2.095 - 43.
Vetchinkina E, Loshchinina E, Kupryashina M, Burov A, Pylaev T, Nikitina V. Green synthesis of nanoparticles with extracellular and intracellular extracts of basidiomycetes. PeerJ. 2018; 6 :e5237. DOI: 10.7717/peerj.5237 - 44.
Das VL, Thomas R, Varghese RT, Soniya EV, Mathew J, Radhakrishnan EK. Extracellular synthesis of silver nanoparticles by the Bacillus strain CS 11 isolated from industrialized area. 3 Biotechnology. 2014;4 (2):121-126. DOI: 10.1007/s13205-013-0130-8 - 45.
Singh P, Kim YJ, Zhang D, Yang DC. Biological synthesis of nanoparticles from plants and microorganisms. Trends in Biotechnology. 2016; 34 (7):588-599. DOI: 10.1016/j.tibtech.2016.02.006 - 46.
Messaoudi O, Bendahou M. Biological synthesis of nanoparticles using endophytic microorganisms: Current development. In: Nanotechnology and the Environment. London, UK: IntechOpen; 2020. DOI: 10.5772/intechopen.93734 - 47.
Mukherjee P, Ahmad A, Mandal D, Senapati S, Sainkar SR, Khan MI, et al. Fungus-mediated synthesis of silver nanoparticles and their immobilization in the mycelial matrix: A novel biological approach to nanoparticle synthesis. Nano Letters. 2001; 1 (10):515-519. DOI: 10.1021/nl0155274 - 48.
Syed A, Ahmad A. Extracellular biosynthesis of platinum nanoparticles using the fungus fusarium oxysporum . Colloids and Surfaces. B, Biointerfaces. 2012;97 :27-31. DOI: 10.1016/j.colsurfb.2012.03.026 - 49.
Riddin TL, Gericke M, Whiteley CG. Analysis of the inter- and extracellular formation of platinum nanoparticles by fusarium oxysporum f. sp.lycopersici using response surface methodology. Nanotechnology. 2006;17 (14):3482-3489. DOI: 10.1088/0957-4484/17/14/021 - 50.
Rana A, Yadav K, Jagadevan S. A comprehensive review on green synthesis of nature-inspired metal nanoparticles: Mechanism, application and toxicity. Journal of Cleaner Production. 2020; 272 :122880. DOI: 10.1016/j.jclepro.2020.122880 - 51.
Dahoumane SA, Yéprémian C, Djédiat C, Couté A, Fiévet F, Coradin T, et al. A global approach of the mechanism involved in the biosynthesis of gold colloids using micro-algae. Journal of Nanoparticle Research. 2014; 16 (10):2607. DOI: 10.1007/s11051-014-2607-8 - 52.
Carrillo-López LM, Soto-Hernández RM, Zavaleta-Mancera HA, Vilchis-Néstor AR. Study of the performance of the organic extracts of Chenopodium ambrosioides for Ag nanoparticle synthesis. Journal of Nanomaterials. 2016;2016 :1-13. DOI: 10.1155/2016/4714162 - 53.
Dauthal P, Mukhopadhyay M. Noble metal nanoparticles: Plant-mediated synthesis, mechanistic aspects of synthesis, and applications. Industrial and Engineering Chemistry Research. 2016; 55 (36):9557-9577. DOI: 10.1021/acs.iecr.6b00861 - 54.
Saim AK, Kumah FN, Oppong MN. Extracellular and intracellular synthesis of gold and silver nanoparticles by living plants: A review. Nanotechnology for Environmental Engineering. 2021; 6 (1):1. DOI: 10.1007/s41204-020-00095-9 - 55.
Naikoo GA, Mustaqeem M, Hassan IU, Awan T, Arshad F, Salim H, et al. Bioinspired and green synthesis of nanoparticles from plant extracts with antiviral and antimicrobial properties: A critical review. Journal of Saudi Chemical Society. 2021; 25 (9):101304. DOI: 10.1016/j.jscs.2021.101304 - 56.
Álvarez-Chimal R, García-Pérez VI, Álvarez-Pérez MA, Tavera-Hernández R, Reyes-Carmona L, Martínez-Hernández M, et al. Influence of the particle size on the antibacterial activity of green synthesized zinc oxide nanoparticles using Dysphania ambrosioides extract, supported by molecular docking analysis. Arabian Journal of Chemistry. 2022;15 (6):103804. DOI: 10.1016/j.arabjc.2022.103804 - 57.
Thanh NTK, Maclean N, Mahiddine S. Mechanisms of nucleation and growth of nanoparticles in solution. Chemical Reviews. 2014; 114 (15):7610-7630. DOI: 10.1021/cr400544s - 58.
Handayani W, Ningrum AS, Imawan C. The role of pH in synthesis silver nanoparticles using Pometia pinnata (Matoa) leaves extract as bioreductor. Journal of Physics: Conference Series. 2020;1428 (1):012021. DOI: 10.1088/1742-6596/1428/1/012021 - 59.
De Oliveira RC, Amoresi RAC, Marana NL, Zaghete MA, Ponce M, Chiquito AJ, et al. Influence of synthesis time on the morphology and properties of CeO2 nanoparticles: An experimental–Theoretical study. Crystal Growth and Design. 2020; 20 (8):5031-5042. DOI: 10.1021/acs.cgd.0c00165 - 60.
Kuppusamy P, Yusoff MM, Maniam GP, Govindan N. Biosynthesis of metallic nanoparticles using plant derivatives and their new avenues in pharmacological applications – An updated report. Saudi Pharmaceutical Journal. 2016; 24 (4):473-484. DOI: 10.1016/j.jsps.2014.11.013. DOI: 10.1016/j.molstruc.2016.12.069 - 61.
Tippayawat P, Phromviyo N, Boueroy P, Chompoosor A. Green synthesis of silver nanoparticles in aloe vera plant extract prepared by a hydrothermal method and their synergistic antibacterial activity. PeerJ. 2016;4 . DOI: 10.7717/peerj.2589 - 62.
Amina M, Al Musayeib NM, Alarfaj NA, El-Tohamy MF, Oraby HF, Al Hamoud GA, et al. Biogenic green synthesis of MgO nanoparticles using Saussurea costus biomasses for a comprehensive detection of their antimicrobial, cytotoxicity against MCF-7 breast cancer cells and photocatalysis potentials. PLoS One. 2020;15 (8). DOI: 10.1371/journal.pone.0237567 - 63.
Ghorbani HR. Extracellular synthesis of copper nanoparticles using culture supernatants of salmonella typhimurium . Oriental Journal of Chemistry. 2015;31 (1):527-529. DOI: 10.13005/ojc/310165 - 64.
Singh AK, Tiwari R, Kumar V, Singh P, Riyazat Khadim SK, Tiwari A, et al. Photo-induced biosynthesis of silver nanoparticles from aqueous extract of Dunaliella salina and their anticancer potential. Journal of Photochemistry and Photobiology, B: Biology. 2017;166 :202-211. DOI: 10.1016/j.jphotobiol.2016.11.020 - 65.
Nava OJ, Luque PA, Gómez-Gutiérrez CM, Vilchis-Nestor AR, Castro-Beltrán A, Mota-González ML, et al. Influence of Camellia sinensis extract on zinc oxide nanoparticle green synthesis. Journal of Molecular Structure. 2017;1134 :121-125 - 66.
Duan H, Wang D, Li Y. Green chemistry for nanoparticle synthesis. Chemical Society Reviews. 2015; 44 (16):5778-5792. DOI: 10.1039/C4CS00363B - 67.
Razavi M, Salahinejad E, Fahmy M, Yazdimamaghani M, Vashaee D, Tayebi L. Green Chemical and Biological Synthesis of Nanoparticles and their Biomedical Applications. Green Processes for Nanotechnology. Cham: Springer International Publishing; 2015. pp. 207-235. DOI: 10.1007/978-3-319-15461-9_7 - 68.
Badria F, AbouHabieb M, Bar FA. Synthesis of Nanoparticles Using Green Chemistry Green Synthesis. 1. Auflage ed. München: Grin Verlag; 2019 - 69.
Chopra H, Bibi S, Singh I, Hasan MM, Khan MS, Yousafi Q , et al. Green metallic nanoparticles: Biosynthesis to applications. Frontiers in Bioengineering and Biotechnology. 2022; 10 :874742. DOI: 10.3389/fbioe.2022.874742 - 70.
Michael A, Singh A, Roy A, Islam MR. Fungal- and algal-derived synthesis of various nanoparticles and their applications. Bioinorganic Chemistry and Applications. 2022; 2022 :1-14. DOI: 10.1155/2022/3142674 - 71.
Raj S, Trivedi R, Soni V. Biogenic synthesis of silver nanoparticles, characterization and their applications—A review. Surfaces. 2021; 5 (1):67-90. DOI: 10.3390/surfaces5010003 - 72.
Kebede MA, Wubieneh TA, Yohannes YB, Shah KJ. Green synthesis of zinc oxide from aqueous fruit extract of Dovyalis abyssinica (Koshem) and application for water purification. Ethiopian Journal of Science and Technology. 2023;16 (1):1-12. DOI: 10.4314/ejst.v16i1.1