Abstract
Linked color imaging (LCI), distinct from the traditional red emphasis in endoscopic imaging, utilizes narrow-band light and red signal processing to enhance contrast in mucosal color. Redness and erosion triggered by inflammation are recognized as typical signs of Helicobacter pylori (HP) infection. Notably, these visual cues are readily identifiable through the LCI mode. Leveraging the LCI observation mode significantly improves the accuracy of diagnosing gastritis associated with HP, providing accurate diagnostic performance and precise localization. LCI demonstrates superior diagnostic accuracy in detecting HP infections, including their precise localization, when compared to traditional white light endoscopy. It also holds promise for enhancing the precision of sampling for HP cultures and determining antibiotic susceptibility. Integrating LCI with the Kyoto Classification of Gastritis may enhance the endoscopic diagnosis and confirmation of HP infection eradication, subsequently augmenting the capacity of gastrointestinal endoscopists and general practitioners to identify HP infections through endoscopic imagery.
Keywords
- linked color imaging
- image-enhancing endoscopy
- Helicobacter pylori
- accuracy
- targeted biopsies
- artificial intelligence
- education
1. Introduction
In recent times, progress in image-enhancing endoscopy (IEE) has significantly enhanced the capacity to identify and diagnose gastric cancer in its early stages. Methods encompassed by IEE, such as narrow band imaging (NBI), blue laser imaging (BLI), and linked color imaging (LCI) [1], are instrumental in advancing this field. Particularly, LCI, as a distinct mode within the blue-laser endoscopy framework, intensifies the contrast of red hues relative to the surrounding area by adjusting the light components’ intensity and mix [2]. In LCI, post-processing boosts brightness and color contrast, making it well-suited for distant views. This enhancement allows red areas to appear more vividly red and white areas to appear whiter, facilitating the identification of suspicious lesions by endoscopists. A key feature of LCI is its ability to provide images with enhanced color differences (CD), aiding in the differentiation of suspicious lesions from the surrounding mucosa based on color. Studies have reported that the demarcation of lesions in LCI mode is clearer than that in white light imaging (WLI) mode [3].
LCI effectively distinguishes early gastric cancer (EGC) as orange-red and intestinal metaplasia as purple, regardless of
Real-time endoscopy is crucial for diagnosing HP infection and facilitating targeted biopsy [5]. In clinical practice, real-time endoscopy in conjunction with conventional WLI and IEE techniques, such as NBI, LCI, and BLI, plays a significant role in identifying HP-infected status [6]. Compared to conventional WLI, LCI has been demonstrated to aid in determining HP infection status, a factor closely linked to the risk of developing gastric cancer. Additionally, LCI offers enhanced detection of gastric cancer, owing to its superior color differentiation when compared to adjacent tissues. This improvement enhances lesion detection in distant views and facilitates the more accurate diagnosis of gastric neoplasms.
2. LCI character of HP state
The presence of HP is commonly associated with increased susceptibility to gastric cancer, a condition often preceded by chronic gastritis, marked atrophy of the gastric mucosa, and intestinal metaplasia [7]. During endoscopic stomach screenings, it is vital to categorize gastritis into three phases: never-infected, currently-infected, and previously-infected, which also includes an after-eradication phase. Retrospective studies conducted in Japan have shown that LCI is superior to WLI in accurately identifying HP-positive mucosa, boasting a sensitivity of 93.3% and a specificity of 78.3% [8]. Therefore, LCI appears to play a crucial role in determining the status of HP infections.
2.1 HP-uninfected mucosa
HP-negative mucosa after eradication is distinctly observed as apricot in color (Figure 1A and B). The adjacent mucosal tissue uniformly presented a pale orange hue, reminiscent of a white-apricot blend, in all areas containing fundic glands. It’s noteworthy that the color characteristics of gastric lesions may be affected by the absorption of violet light at 410 nm within the superficial layer of the mucosa [8].
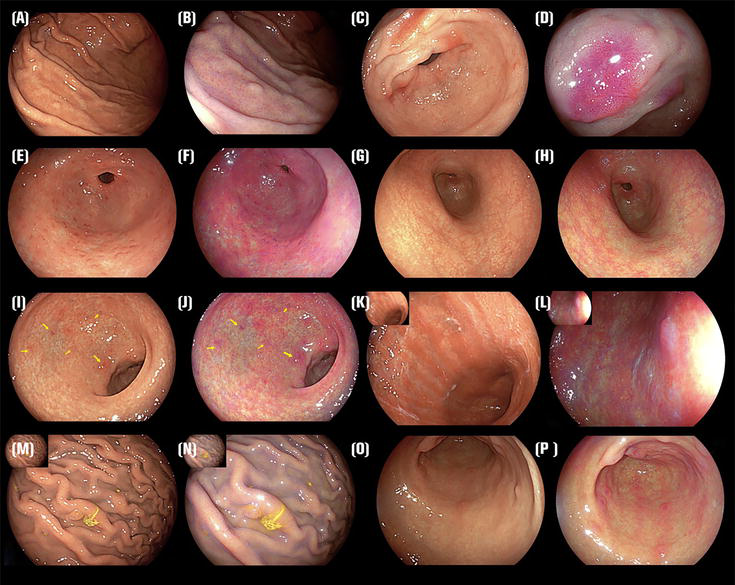
Figure 1.
Endoscopic schematic diagram: (A) WLI of RAC (+) with HP uninfected, (B) LCI of RAC (+) with HP uninfected, (C) WLI of diffuse redness, (D) LCI of diffuse redness, (E) WLI of map-like redness, (F) LCI of map-like redness, (G) WLI of nodular gastritis, (H) LCI of nodular gastritis, (I) WLI of atrophic gastritis (type C) and intestinal metaplasia (arrowheads), (J) LCI of atrophic gastritis (type C) and intestinal metaplasia (arrowheads), (K) WLI of atrophic gastritis (type O), (L) LCI of atrophic gastritis (type O), (M) WLI of rugal hypertrophy and fundie gland polyps, (N) LCI of rugal hypertrophy and fundic gland polyps, (O) WLI of red streak, (P) LCI of red streak.
2.2 HP-infected mucosa
In LCI imaging, the widespread redness seen in the gastric mucosa of the gastric body, a hallmark sign of present HP infection, appears distinctly as a deep crimson color (Figure 1C–P) [9], while intestinal metaplasia appears clearly as lavender (Figure 1I and J arrowheads). Regarding LCI visuals, the indication of HP infection is identified by any of these criteria: (1) widespread reddening; (2) absence of a regular arrangement of collecting venules (RAC); and (3) adherence of mucus [10].
A study conducted a correlation analysis to assess the indicators associated with HP infection. Based on the findings of skilled endoscopists, there was a notable association between HP infection and several indicators: WLI and LCI scores, the reddening and purpleness of the fundic gland mucosa, and the cloudiness of the mucus lake [11]. In contrast, granular erosion, erythema, and loss of collecting venules were moderately correlated with HP. According to Dohi et al., LCI enhanced the endoscopic diagnosis of active HP infections, demonstrating improvements of 10–15% in accuracy, sensitivity, and specificity compared to WLI [8]. The rate of diagnosis using the LCI method for HP infection surpassed that of other methods.
LCI may serve as an effective preliminary diagnostic instrument for HP infections in real-time applications, offering a notable level of diagnostic precision. Shida et al. concluded that LED endoscopy is equivalent to laser endoscopy for assessing HP infection status [12].
In a prospective study, the distinction in hue at the degenerated margins was markedly more pronounced using LCI than WLI, irrespective of the HP infection status. LCI exhibited a notable enhancement in diagnostic precision for subjects with either a current or historical HP infection, as opposed to WLI. Conversely, in prospective diagnostic research focusing on HP infection, the diagnostic precision, sensitivity, and specificity metrics for WLI, LCI, and magnified endoscopy using WLI, when evaluating the presence of RAC or general erythema, did not present considerable variances [13].
2.3 HP-eradicated mucosa
After eradication, HP-negative mucosa is distinctly observed as apricot in color. However, a considerable amount of red depression is evident extensively throughout the gastric mucosa, spanning from the gastric body to the antrum. It has been observed that through the application of LCI technology, the mucosa of the fundic gland exhibits widespread redness (resembling a deep shade of crimson) in cases of active gastritis, whereas it manifests a pale orange hue (akin to the shade of white apricots) in stomachs where HP has been successfully eradicated [14]. Majima et al. found that LCI detects the positive risk indicator of map-like redness (MR) (Figure 1E and F). The negative risk indicator of a regular arrangement of collecting venules for early gastric cancer (EGC) detection after HP eradication is more frequent than WLI [15]. LCI was more efficient for evaluating MR than WLI because LCI could identify MR more frequently than WLI. Hence, it might be tentatively inferred that the influence of external variables on the LCI mode was relatively minimal regarding the diagnosis of HP. The study revealed that when reviewing recorded endoscopies, both expert and trainee endoscopists had a notably reduced miss rate for EGC post-eradication when using LCI compared to WLI. Concerning the objective analyses, it evaluated the CD of each lesion on WLI and LCI images [16].
2.4 The meta-analysis and limitation
To thoroughly assess the diagnostic efficacy of LCI and WLI in detecting HP infection, we conducted a meta-analysis. It employs a meticulously crafted framework of study inclusion criteria, ensuring a high standard of selection precision. In conducting this analysis, we rigorously adhered to a set of carefully pre-established criteria for selecting relevant studies. Included studies were either randomized controlled trials or observational studies, aimed at comparing the sensitivity and specificity of LCI versus WLI in detecting HP infection. The investigation entailed systematic searches within the PubMed and CNKI databases, utilizing key phrases including “((Linked Color Imaging) AND (White Light Imaging)) AND (
We employed the QUADAS-2 framework for evaluating the quality of the studies we included, focusing on the bias risk and relevance in aspects such as patient selection, index tests, reference standards, flow, and timing. Based on these parameters, each study was classified as a “low,” “high,” or “unclear” risk. In our meta-analysis, most studies were deemed low risk in patient selection and index tests, but some showed moderate risk in flow and timing (Figure 2).
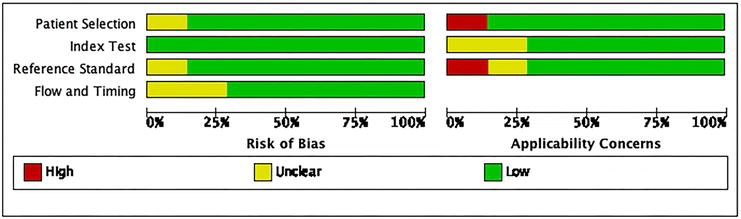
Figure 2.
Assessment of study quality using QUADAS-2 tool presents bar graphs depicting the risk of bias and applicability concerns in various studies. The majority of included randomized controlled trials (RCTs) demonstrated a low risk in patient selection and index testing (axes: horizontal axis: proportion (%) ranging from 0% to 100%, vertical axis: aspects of study quality assessment: patient selection, index tests, reference standards, flow and timing, color legend: red: high risk, yellow: unclear risk, green: low risk).
The meta-analysis results, synthesized through a random-effects model, demonstrated LCI’s superior sensitivity (91%) and specificity (88%) in detecting HP infection. This contrasts with WLI’s sensitivity and specificity, which stood at 82% and 80%, respectively. Our heterogeneity assessment revealed I2 values of 45% for sensitivity and 50% for specificity, indicating moderate variability across studies. Nonetheless, the observed
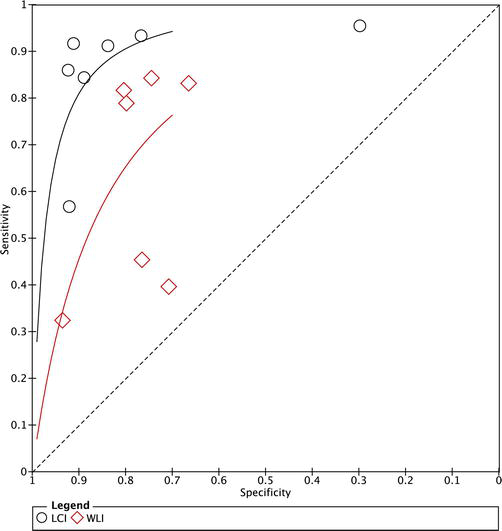
Figure 3.
SROC curve comparison: LCI vs. WLI. LCI demonstrates superior efficacy over WLI in HP detection. This figure compares the receiver operating characteristic (ROC) curves of LCI and WLI for detecting HP infection. The x-axis is “1-specificity” (false-positive rate), and the y-axis is sensitivity (true-positive rate). LCI data points are represented by hollow circles and WLI data points by hollow diamonds. The AUC reflects test accuracy, with higher values indicating better sensitivity and specificity. LCI demonstrates superior efficacy over WLI in HP detection.
In instances of pronounced atrophy, the hue alterations post-HP infection tend to be inconspicuous, resulting in a lack of widespread redness when observed using LCI technology. The negative predictive value (NPV) of RAC exhibits optimal performance, in contrast, the positive predictive value (PPV) is notably inadequate. Furthermore, the morphological attributes exhibit variations throughout the different phases of atrophic evolution [17]. Since various endoscopic characteristics must be comprehensively assessed for an accurate endoscopic diagnosis, inter-observer bias exists in the studies.
In summary, LCI demonstrates a clear advantage in diagnosing HP infection. Its high sensitivity and specificity, coupled with superior overall diagnostic efficacy, render LCI a more valuable diagnostic tool. Despite some heterogeneity among studies, LCI’s overall performance stands out, offering robust technological support for clinical diagnosis.
3. LCI for screening early gastric cancer
Moreover, the diverse coloration present in the surrounding mucosal tissue raises debates regarding whether color intensification aids in identifying EGC. In the context of WLI, the endoscopic visuals of these observations closely mimic cancerous appearances, making the differentiation between non-malignant and malignant growths challenging. In contrast, gastric cancer is recognized as orange or magenta, while surrounding lesions are observed in lavender color in LCI, allowing for clearer observation compared to WLI due to enhanced color contrast [16]. The presence of intestinal metaplasia is characterized by a distinct lavender coloration. Because of these prominent color disparities, research indicates that LCI has a markedly superior detection rate for intestinal metaplasia compared to WLI [18]. Additionally, a study conducted by Ono et al. further supported that the lavender hue observed in LCI facilitates the non-invasive identification of gastrointestinal metaplasia [19]. MR was identified more frequently using LCI than WLI, and the absence of RAC was associated with the detection of EGC after the eradication of HP [15]. LCI had the highest visibility among findings of gastric mucosa and EGC after HP eradication. A recent meta-analysis demonstrated that LCI exhibits strong diagnostic efficacy for gastrointestinal metaplasia [20]. Additionally, when examined using LCI, the vascular permeation of the atrophic border and the atrophic region becomes more discernible compared to observations made with WLI [21]. It has also been documented that endoscopic procedures utilizing LED for LCI are proficient in the early identification of EGC [22]. The color difference value with LCI was significantly higher, being more than twice that of WLI, and this difference appeared to be similar in cases of accompanying HP infection or intestinal metaplasia. However, no statistically significant finding was present in signet ring cell carcinoma or mixed-type histology [23].
3.1 EGC strategy
3.1.1 HP-related gastritis
The LCI score has been shown to exhibit notable sensitivity and specificity in distinguishing HP-associated gastritis. This underscores its efficacy as a method for detecting HP presence in gastric mucosal tissue [24]. In an investigation, both erythema and non-visible RAC were incorporated into the regression analysis, proving beneficial for distinguishing HP-induced gastritis. Conversely, features like erosions and nodularity were excluded from this model [11]. Mizukami et al.’s research indicated that LCI enhanced the definition of the atrophic border through objective analysis [21]. In a multicenter prospective study conducted by Ono et al., which compared the accuracy of WLI and LCI for the endoscopic diagnosis of HP gastritis, LCI exhibited notably superior performance over WLI, particularly in patients with a history of past infections [25]. LED-LCI was found to be more effective than WLI for evaluating HP-related gastritis. However, another study suggested no significant differences in accuracy, sensitivity, or specificity for diagnosing metaplastic gastritis between LCI and WLI [26].
3.1.2 MESDA-G
The Magnifying Endoscopy Simple Diagnostic Algorithm for Early Gastric Cancer (MESDA-G) provides an extensive framework for the early detection of gastric cancer. It evaluates the boundary differentiation between abnormal lesions and normal gastric tissue, as well as identifies anomalies in the microvascular structure and surface patterns of the stomach lining [27]. Given that most gastric cancers are enveloped by intestinal metaplasia, LCI proves valuable in depicting this metaplasia in pale purple. The majority of the surrounding mucosa is also represented in purple, creating a high color contrast. In the LCI view, early-stage gastric cancers typically appear orange-red, orange, or orange-white [1]. It becomes crucial to identify gastric cancer (depicted in orange) amidst intestinal epithelial metaplasia (depicted in purple) using LCI. Subsequent clarification of the diagnosis can be achieved through magnifying BLI. LCI has proven to be effective in the early detection of flat gastric cancers, manifesting as distinct reddish lesions against the surrounding mucosa [28]. In the LCI view, undifferentiated gastric cancer exhibits a faded tone, characteristic of mucosa in HP-uninfected cases, offering clear color differentiation [2]. When LCI is followed by magnified BLI, it confidently identifies EGC in an MR post-successful HP eradication, outperforming WLI in clinical practice [29].
Further research is essential to assess the effectiveness of LCI in the screening of gastric cancer among the general populace, particularly emphasizing the need for studies centered around EGC. Recent research conducted by Khurelbaatar et al. revealed that LCI, when compared to conventional WLI, notably improves the visibility of early gastric cancer (EGC), regardless of variations in lesion morphology, histology, location, depth of invasion, and HP status. This observation could potentially lead to the reported enhancements in detection rates and reductions in miss rates [30]. In contrast to BLI, LCI exhibits enhanced visibility of EGC, irrespective of the endoscopist’s experience [31].
3.1.3 Kyoto classification
The Kyoto classification system for gastritis was formulated primarily to highlight the endoscopic characteristics linked with HP-related gastritis. This system is instrumental in the precise diagnosis of HP infections and in pinpointing the risk factors for gastric carcinoma. In the process of gastroduodenoscopy, it’s advised to assess various factors, including nodularity, mucosal atrophy, intestinal metaplasia, enlarged folds, and widespread redness (Figure 1G–P). The cumulative score of these elements is used as an index to gauge the risk of gastric cancer. According to this classification, a score of 0 suggests gastritis that is not associated with HP, whereas a score of 2 or higher is indicative of HP-induced gastritis, with a reported diagnostic accuracy of 90% [32].
Notably, LCI has demonstrated utility in evaluating gastritis based on the Kyoto classification [33]. LCI excels in diagnosing mucosal atrophy, intestinal metaplasia, diffuse redness, RAC, spotty redness, and MR from a distant view compared to WLI. Utilizing LCI significantly enhances the detectability of gastroscopic observations in line with the Kyoto Gastritis Classification, presenting a marked improvement over WLI [34]. Moreover, the diagnostic value of the LCI combined score surpasses that of the WLI combined score and each observation index of WLI. These findings suggest that LCI may offer distinct advantages in diagnosing the Kyoto classification of gastritis compared to other IEEs, emphasizing the need for prospective assessments to further validate these potential benefits.
3.1.4 Targeted biopsies
Numerous clinical studies have emphasized the potential of IEEs in identifying mucosal changes, enabling precisely targeted biopsies [11]. In individuals never infected with HP, it was observed that LCI exhibited a greater color difference at the fundic and pyloric mucosal borders compared to WLI. The enhanced detection of atrophic borders with LCI makes it a recommended choice for trans-nasal endoscopy in health check-ups, particularly for identifying individuals at a high risk of gastric carcinoma [5].
4. Mechanism
LCI emerges as an innovative technique in IEEs, utilizing a combination of short-wavelength narrow-band and white-light laser illumination in tandem with traditional WLI. This integration of dual-light modalities enables LCI to gather detailed insights into mucosal surface textures and vascular patterns [35]. In comparison to white light imaging, LCI employs advanced post-processing steps to enhance subtle differences in color tone, with a higher emission intensity of 410 nm influencing the mucosal color of various lesions [34]. Functioning as a cutting-edge IEE technology, LCI excels at highlighting even the slightest differences in mucosal color, making it a powerful tool for the endoscopic diagnosis of gastrointestinal inflammation [1]. The capability of LCI to enhance color differences is particularly beneficial in scenarios of active mucosal inflammation, where hyperemia manifests as noticeable redness. Conversely, mild or inactive inflammation may not exhibit obvious signs during traditional endoscopic examination. LCI, being highly sensitive to color changes, emerges as a convenient and effective tool for detecting these nuanced differences and facilitating a more accurate and differential diagnosis [18].
LCI’s unique characteristic of heightened sensitivity to color differences is employed to vividly depict mucosal surface blood vessels and illustrate distinctions in mucosal surface tissue structure, including surface blood vessels [11]. The ELUXEO system was developed with a specific focus on precisely regulating the emission intensity ratio of LED illumination across four colors: blue-violet, blue, green, and red. This setup enables the generation of both white light and short-wavelength narrow-band light. The objective behind this design is to generate high-quality images akin to those produced by LASEREO. With LED-LCI, it is anticipated to achieve diagnostic performance comparable to conventional laser-induced LCI [1].
The enhanced brightness of LCI images is anticipated to increase their utility in detecting subtle irregularities on the gastric surface in patients. Significantly, it has been observed that the orderly pattern of collecting venules, mainly notable in the lesser curvature area, is linked with gastric mucosa not affected by HP and a decreased likelihood of developing gastric cancer [12]. Bright LCI, by highlighting slight irregularities in the surface epithelium even from middle to distant views, significantly improves the ability to detect EGC post-eradication [32]. LCI exhibited the most significant odds ratio compared to factors like elevated morphology and submucosal invasion. Several studies have highlighted the substantial color contrast observed between EGCs and the adjacent mucosa when utilizing LCI. This underscores the crucial role of high color contrast in improving the detectability of EGCs [16, 18].
Fine-tuning color enhancement settings prove instrumental in enhancing the visibility of malignant lesions within the stomach, fostering a clearer color contrast between these lesions and the surrounding mucosa. It is significant to observe that the average scores for visibility, when enhanced with C2 for LCI, were markedly superior in comparison to the results obtained with C1 enhancement [30]. However, it’s worth noting that this enhancement, to some extent, compromises the consistency between examiners. To enhance diagnostic accuracy and align with clinical requirements for consistency between examiners, it becomes imperative to improve the consistency of individual observation indices and minimize subjectivity in the evaluation process [36]. Striking the right balance in color enhancement settings is pivotal, ensuring optimal visibility without sacrificing reliability and agreement among different examiners.
5. Artificial intelligence
The integration of artificial intelligence (AI) technology with IEEs is emerging as a potent and innovative diagnostic approach in the evolving landscape of medical imaging. In a pioneering research effort by Sun et al. in China, MATLAB software was applied for the analysis of endoscopic imagery in the LCI mode, specifically targeting the antral region. This research emphasized the extraction and utilization of specific data pertaining to brightness and hue, applying these metrics to the identification and diagnosis of a range of gastric ailments. These include HP infections, gastric atrophy, intestinal metaplasia, and the early stages of gastric cancer [11]. The incorporation of AI, notably through convolutional neural network-based computer-aided diagnosis (CAD) systems, has made significant advancements in gastrointestinal endoscopy. This technology is becoming more widely available and utilized for the detection of HP infection status and gastric cancer [37].
Numerous studies highlight the remarkable capabilities of CAD when integrated with LCI or BLI-bright in discerning the HP infection status [38]. In the study, Nakashima and colleagues demonstrated the effective diagnostic performance of LCI-CAD in categorizing the status of HP infection, effectively differentiating among cases where HP was present, absent, or had been successfully eradicated [39]. The application of AI in diagnostic imaging is expected to alleviate the burden of double-checking, effectively identifying patients in need of follow-up endoscopy while providing crucial support for non-expert endoscopists [40]. In comparison to experienced endoscopists, the study’s results revealed that AI exhibits greater sensitivity in diagnosing HP infection, boasting a sensitivity and specificity of 81.9/83.4% and 79/83.2%, respectively. The integration of AI in HP infection diagnosis aims to mitigate interobserver disagreement and reduce time consumption in the diagnostic process. The evolving landscape of AI in conjunction with IEE holds immense promise for advancing the accuracy, efficiency, and accessibility of gastrointestinal diagnostics.
Owing to its elevated rate of HP infections and the extensive need for gastric carcinoma screenings, Japan has emerged as a leading nation in conducting research into the integration of AI within the realm of endoscopic techniques [41]. A prospective pilot study conducted in Japan compared AI-assisted BLI with bright light (AI-BLI-bright), LCI, and WLI in diagnosing HP infection. The performance metrics, specifically the AUC, for both AI-BLI-bright and AI-LCI were impressively elevated, registering at 0.96 and 0.95, respectively. In contrast, AI-WLI demonstrated a lower efficacy with an AUC score of 0.66 [42].
The challenge in the endoscopic diagnosis of HP infection lies in the lack of objective indicators, leading to variations in diagnostic abilities among doctors. Consequently, it becomes crucial to develop methods with universal interpretability, like CAD systems, for the endoscopic assessment of HP infection. The goals of a recent study were to (i) develop an interpretable automated diagnostic system utilizing a support vector machine (SVM) for detecting HP infection, (ii) assess and contrast the diagnostic performance of the AI system with that of endoscopists. The findings indicated that the artificial intelligence system attained levels of accuracy, sensitivity, and specificity, as well as positive and negative predictive values, which were recorded at 87.6%, 90.4%, 85.7%, 80.9%, and 93.1%, respectively. This signifies that the AI system can diagnose HP infection with significant accuracy [39]. The integration of AI systems in endoscopic practices holds great promise for enhancing diagnostic capabilities, standardizing assessments, and contributing to more effective and efficient medical care.
Numerous studies have delved into the CAD of HP infection through deep learning (DL) algorithms. The computer-aided diagnosis system, developed using the GoogLeNet architecture of convolutional neural networks, has shown remarkable efficiency in diagnosing HP infections, thereby illustrating the significant capabilities of deep learning algorithms in the field of gastroenterology [43]. Another CAD, also based on DL algorithms, exhibited significantly higher accuracy and shorter diagnostic times compared to endoscopists in diagnosing HP infection [44].
Concurrently, CAD using IEE images has been actively explored. A computer-aided diagnosis system, powered by deep learning algorithms, demonstrated significantly enhanced precision in detecting anomalies using BLI-bright and LCI, in contrast to the results obtained with traditional WLI [45]. Similarly, a CAD system based on a conventional support vector machine, utilizing LCI, demonstrated diagnostic yields on part with those of expert endoscopists [39].
In broadening its application, CAD encompasses the use of the Kyoto criteria for gastritis in the endoscopic identification of HP infections. A predictive model, employing a machine learning approach and incorporating endoscopic features derived from the Kyoto classification of gastritis, demonstrated a high level of diagnostic accuracy for determining HP infection status. This accuracy was found to be comparable to evaluations made by experienced endoscopists [43]. These findings underscore the growing potential of CAD, both with DL algorithms and traditional methods, in revolutionizing the accuracy, efficiency, and objectivity of diagnosing HP infection and related gastric conditions.
6. Education
Fukuda et al. brought attention to a noteworthy challenge in lesion recognition, reporting that malignant lesions appearing redder than the surrounding mucosa on WLI may manifest as purple on LCI. This color shift could potentially lead to the oversight of malignant lesions, even in the presence of surface irregularities. Therefore, it has become imperative to enhance educational efforts to heighten recognition of the importance attached to the purple hue observed in LCI [46]. Despite the complexities involved, assessing HP infection via indicators such as widespread erythema, loss of the typical collecting venule pattern, and mucus adhesion when using LCI proves to be a dependable method. These indicators are deemed attainable skills for gastrointestinal endoscopists, covering a range of expertise from novices to seasoned professionals. Nonetheless, it is underscored that additional research, particularly with a focus on practitioners of varying experience levels, is crucial to fully comprehending the benefits of LCI in the educational spectrum of endoscopy. Analyzing results before and after systematic training will contribute to a comprehensive assessment of LCI’s efficacy [18].
In an extensive research project, clarity levels and interclass correlation coefficients (ICCs) were utilized to evaluate the visibility and consistency among different raters for both LCI and BLI-bright, relative to traditional WLI. This assessment was conducted based on the findings observed through endoscopy, which were in line with the criteria outlined in the Kyoto Classification of Gastritis. Notably, statistically significant differences in visibility scores for LCI were observed between trainees and experts, particularly in categories such as spotty, patchy, and MR. Trainees exhibited significantly higher visibility scores than experts for spotty redness observed through LCI, suggesting that LCI may enhance diagnostic performance in the assessment of spotty redness by non-experts [10].
Building on this insight, our team embarked on an initiative to integrate LCI with the Kyoto Classification of Gastritis, forming a training model tailored for general practitioners (GPs). Expert endoscopists’ analysis of the Kyoto Classification of Gastritis revealed that gastroscopic mucosal manifestations such as mucosal atrophy, hematin deposition, mucosal swelling, and the presence of sticky mucus influenced their assessment of HP infection. Subsequent to the training program, there was a notable enhancement in the overall scores achieved by GPs in the questionnaire. Additionally, the time taken to complete the questionnaire was comparable between the GPs and established gastroenterology specialists. Following training, general practitioners’ questionnaire scores improved based on images observed in both WLI and LCI modes. Notably, the score achieved with the LCI mode was superior to that obtained with the WLI mode. The conclusion of the study is that the Kyoto Classification of Gastritis combined with LCI improves the ability of GPs to diagnose HP infection through endoscopic images.
7. Conclusion
LCI observation mode can enhance the diagnosis rate of HP infection-related gastritis, providing accurate diagnostic performance and precise localization. LCI demonstrates a high level of diagnostic accuracy in identifying HP infections, including their precise locations, when contrasted with traditional white-light endoscopic methods. Additionally, LCI shows promise in enhancing the precision of sample collection for HP culture and its antibiotic sensitivity analysis. Integrating LCI with the Kyoto Classification of Gastritis could potentially elevate the effectiveness of diagnosing HP infections or confirming their eradication during endoscopic procedures. This advancement might significantly bolster the capabilities of gastrointestinal endoscopists and general practitioners in diagnosing HP infections through endoscopic imaging.
Acknowledgments
This study was supported by the Guangdong Basic and Applied Basic Research Foundation (2022B1515130004). Thanks to Lanfeng Xue, Yujia Guo, and Haiyun Zhuang who performed the experiments and meta-analysis, as well as the figures collection.
References
- 1.
Osawa H, Miura Y, Takezawa T, et al. Linked color imaging and blue laser imaging for gastrointestinal screening. Clinical Endoscopy. 2018; 51 :513-526. DOI: 10.5946/ce.2018.132 - 2.
Kanzaki H, Takenaka R, Kawahara Y, Kawai D, Obayashi Y, Baba Y, et al. Linked color imaging (LCI), a novel image-enhanced endoscopy technology, emphasizes the color of early gastric cancer. Endoscopy International Open. 2017; 5 :E1005-E1013. DOI: 10.1055/s-0043-117881 - 3.
Fukuda H, Miura Y, Hayashi Y, et al. Linked color imaging technology facilitates the early detection of fat gastric cancers. Clinical Journal of Gastroenterology. 2015; 8 :385-389. DOI: 10.1007/s12328-015-0612-9 - 4.
Yashima K, Onoyama T, Kurumi H, et al. Current status and future perspective of linked color imaging for gastric cancer screening: A literature review. Journal of Gastroenterology. Jan 2023; 58 (1):1-13. DOI: 10.1007/s00535-022-01934-z. Epub 2022 Oct 26. Erratum in: J Gastroenterol. 2023 May;58(5):514. PMID: 36287268; PMCID: PMC9825522 - 5.
Chatrangsun B, Vilaichone RK. Endoscopic diagnosis for H. pylori infection: White light imaging (WLI) vs. image-enhanced endoscopy (IEE). Asian Pacific Journal of Cancer Prevention. 2021;22 (9):3031-3038. DOI: 10.31557/APJCP.2021.22.9.3031 - 6.
Malfertheiner P, Megraud F, O’Morain C, et al. Current concepts in the management of Helicobacter pylori infection: The Maastricht III Consensus Report. Gut. 2007;56 :772-781. DOI: 10.1136/gut.2006.101634 - 7.
Uemura N, Okamoto S, Yamamoto S, et al. Helicobacter pylori infection and the development of gastric cancer. The New England Journal of Medicine. 2001;345 :784-789. DOI: 10.1056/NEJMoa001999 - 8.
Dohi O, Yagi N, Onozawa Y, et al. Linked color imaging improves endoscopic diagnosis of active Helicobacter pylori infection. Endoscopy International Open. 2016;4 :E800-E805. DOI: 10.1055/s-0042-109049 - 9.
Hiraoka Y, Miura Y, Osawa H, et al. Linked color imaging demonstrates characteristic findings in semi-pedunculated gastric adenocarcinoma in Helicobacter pylori -negative normal mucosa. Clinical Endoscopy. Jan 2021;54 (1):136-138. DOI: 10.5946/ce.2020.059 - 10.
Wang L, Lin XC, Li HL, Yang XS, Zhang L, Li X, et al. Clinical significance and influencing factors of linked color imaging technique in real-time diagnosis of active Helicobacter pylori infection. Chinese Medical Journal. 2019;132 (20):2395-2401. DOI: 10.1097/CM9.0000000000000486 - 11.
Xiu JZ, Bing N, Juan NJ, Yu HP. Comparison between the linked color and white light imaging combined score in the evaluation of high-risk population of gastric cancer. The Turkish Journal of Gastroenterology. 2021; 32 (10):859-869. DOI: 10.5152/tjg.2021.20799 - 12.
Ishida T, Dohi O, Yoshida N, et al. Enhanced visibility in evaluating gastric cancer 1 and Helicobacter pylori -associated gastritis using linked color imaging with a light-emitting diode light source. Digestive Diseases and Sciences. 2022;67 :2367-2371. DOI: 10.1007/s10620-021-07234-5 - 13.
Chen TH, Hsu CM, Cheng HT, et al. Linked color imaging can help gastric Helicobacter pylori infection diagnosis during endoscopy. Journal of the Chinese Medical Association. 2018;81 :1033-1037. DOI: 10.1016/j.jcma.2018.03.006 - 14.
Iwamuro M, Sakae H, Kanzaki H, Okada H. Diffuse redness in linked color imaging is useful for diagnosing current Helicobacter pylori infection in the stomach. Journal of General and Family Medicine. 2018;19 (5):176-177. DOI: 10.1002/jgf2.195 PMID: 30186732 - 15.
Majima A, Dohi O, Takayama S, et al. Linked color imaging identifies important risk factors associated with gastric cancer after the successful eradication of Helicobacter pylori . Gastrointestinal Endoscopy. 2019;90 :763-769. DOI: 10.1016/j.gie.2019.06.043 - 16.
Kitagawa Y, Suzuki T, Nankinzan R, Ishigaki A, Furukawa K, Sugita O, et al. Comparison of endoscopic visibility and miss rate for early gastric cancers after Helicobacter pylori eradication with white-light imaging versus linked color imaging. Digestive Endoscopy. 2020;32 (5):769-777. DOI: 10.1111/den.13585 - 17.
Nishikawa Y, Ikeda Y, Murakami H, Hori SI, Hino K, Sasaki C, et al. Classification of atrophic mucosal patterns on blue Laser imaging for endoscopic diagnosis of Helicobacter pylori -related gastritis: A retrospective, observational study. PLoS ONE. 2018;13 :e0193197. DOI: 10.1371/journal.pone.0193197 - 18.
Fukuda H, Miura Y, Osawa H, et al. Linked color imaging can enhance the recognition of early gastric cancer by high color contrast with the surrounding gastric intestinal metaplasia. Journal of Gastroenterology. 2019; 54 :396-406. DOI: 10.1007/s00535-018-1515-6 - 19.
Ono S, Dohi O, Yagi N, et al. Accuracies of endoscopic diagnosis of Helicobacter pylori -gastritis: A multicenter prospective study using white light imaging and linked color imaging. Digestion. 2020;101 :624-630. DOI: 10.1159/000501634 - 20.
Shu X, Wu G, Zhang Y, et al. Diagnostic value of linked color imaging based on endoscopy for gastric intestinal metaplasia: A systematic review and meta-analysis. Annals of Translational Medicine. 2021; 9 :506. DOI: 10.21037/atm-21-1051 - 21.
Mizukami K, Ogawa R, Okamoto K, et al. Objective endoscopic analysis with linked color imaging for gastric mucosal atrophy: A pilot study. Gastroenterology Research and Practice. 2017; 2017 :5054237. DOI: 10.1155/2017/5054237 - 22.
Esaki M, Minoda Y, Ihara E. In living color: Linked color imaging for the detection of early gastric cancer. Digestive Diseases and Sciences. 2022; 67 :1922-1924. DOI: 10.1007/s10620-021-07235-4 - 23.
Kyung Yoo I, Lee H, Il Kim Y, Young Cho J, Sik LW. Clinical usefulness of linked color imaging for detection and characterization of undifferentiated type early gastric cancer. The Turkish Journal of Gastroenterology. 2023; 34 (4):356-363. DOI: 10.5152/tjg.2023.22684 - 24.
Jiang ZX, Nong B, Liang LX, Yan YD, Zhang G. Differential diagnosis of Helicobacter pylori -associated gastritis with the linked-color imaging score. Digestive and Liver Disease 2019;51 (12):1665-1670. DOI: 10.1016/j.dld.2019.06.024 - 25.
Hatta W, Gotoda T, Oyama T, et al. A scoring system to stratify curability after endoscopic submucosal dissection for early gastric cancer: “eCura system”. The American Journal of Gastroenterology. 2017; 112 :874-881. DOI: 10.1038/ajg.2017.95 - 26.
Lee SP, Lee J, Kae SH, Jang HJ, Koh DH, Jung JH, et al. The role of linked color imaging in endoscopic diagnosis of Helicobacter pylori associated gastritis. Scandinavian Journal of Gastroenterology. 2020;55 (9):1114-1120. DOI: 10.1080/00365521.2020.1794025 - 27.
Muto M, Yao K, Kaise M, et al. Magnifying endoscopy simple diagnostic algorithm for early gastric cancer (MESDA-G). Digestive Endoscopy. 2016; 28 :379-393. DOI: 10.1111/den.12638 - 28.
Ono S, Abiko S, Kato M. Linked color imaging enhances gastric cancer in gastric intestinal metaplasia. Digestive Endoscopy. 2017; 29 (2):230-231. DOI: 10.1111/den.12757 - 29.
Dohi O, Ishida T, Yoshida N. Linked color imaging followed by magnifying blue laser imaging identifies early gastric cancer in map-like redness after successful Helicobacter pylori eradication. Digestive Endoscopy. 2020;32 (5):e109-e111. DOI: 10.1111/den.13708 - 30.
Khurelbaatar T, Miura Y, Osawa H, et al. Usefulness of linked color imaging for the detection of obscure early gastric cancer: Multivariate analysis of 508 lesions. Digestive Endoscopy. 2022; 34 :1012-1020. DOI: 10.1111/den.14221 - 31.
Matsumura S, Dohi O, Yamada N, et al. Improved visibility of early gastric cancer after successful Helicobacter pylori eradication with image-enhanced endoscopy: A multi-institutional study using video clips. Journal of Clinical Medicine. 2021;10 :3649 - 32.
Dohi O, Majima A, Naito Y, Yoshida T, Ishida T, Azuma Y, et al. Can image-enhanced endoscopy improve the diagnosis of Kyoto classification of gastritis in the clinical setting? Digestive Endoscopy. 2020; 32 (2):191-203. DOI: 10.1111/den.13540 - 33.
Toyoshima O, Nishizawa T, Koike K. Endoscopic Kyoto classification of Helicobacter pylori infection and gastric cancer risk diagnosis. World Journal of Gastroenterology. 2020;26 :466-477. DOI: 10.3748/wjg.v26.i5.466 - 34.
Takeda T, Asaoka D, Nojiri S, et al. Linked color imaging and Kyoto classification of gastritis: Evaluation of visibility and inter-rater reliability. Digestion. 2020; 101 :598-607. DOI: 10.1159/000501534 - 35.
Kawai Y, Sugimoto M, Hamada M, Iwata E, Niikura R, Nagata N, et al. Linked color imaging effectively detects the endoscopic atrophic border in transnasal endoscopy. Journal of Clinical Biochemistry and Nutrition. 2022; 70 (3):290-296. DOI: 10.3164/jcbn.21-145 - 36.
Hiraoka Y, Miura Y, Osawa H, Nomoto Y, Takahashi H, Tsunoda M, et al. Appropriate color enhancement settings for blue laser imaging facilitates the diagnosis of early gastric cancer with high color contrast. Journal of Gastric Cancer. 2021; 21 (2):142-154. DOI: 10.5230/jgc.2021.21.e13. Epub 2021 Jun 17 - 37.
Sun X, Bi Y, Dong T, Min M, Shen W, Xu Y, et al. Linked color imaging benefits the endoscopic diagnosis of distal gastric diseases. Scientific Reports. 2017; 7 :5638. DOI: 10.1038/s41598-017-05847-3 - 38.
Kanesaka T, Lee TC, Uedo N, Lin KP, et al. Computer-aided diagnosis for identifying and delineating early gastric cancers in magnifying narrow-band imaging. Gastrointestinal Endoscopy. May 2018; 87 (5):1339-1344. DOI: 10.1016/j.gie.2017.11.029 - 39.
Nakashima H, Kawahira H, Kawachi H, et al. Artificial intelligence diagnosis of Helicobacter pylori infection using blue laser imaging-bright and linked color imaging: A singlecenter prospective study. Annals of Gastroenterology. 2018;31 :462-468. DOI: 10.20524/aog.2018.0269 - 40.
Nakashima H, Kawahira H, Kawachi H, et al. Endoscopic threecategorical diagnosis of Helicobacter pylori infection using linked color imaging and deep learning: A single-center prospective study (with video). Gastric Cancer. 2020;23 :1033-1040. DOI: 10.1007/s10120-020-01077-1 - 41.
Abe S, Oda I. How can endoscopists adapt and collaborate with artificial intelligence for early gastric cancer detection? Digestive Endoscopy. 2021; 33 :98-99. DOI: 10.1111/den.13751 - 42.
Bang CS, Lee JJ, Baik GH. Artificial intelligence for the prediction of Helicobacter pylori infection in endoscopic images: Systematic review and meta-analysis of diagnostic test accuracy. Journal of Medical Internet Research. 2020;22 :e21983. DOI: 10.2196/21983 - 43.
Yasuda T, Hiroyasu T, Hiwa S, Okada Y, Hayashi S, Nakahata Y, et al. Potential of automatic diagnosis system with linked color imaging for diagnosis of Helicobacter pylori infection. Digestive Endoscopy. 2020;32 (3):373-381. DOI: 10.1111/den.13509 - 44.
Itoh T, Kawahira H, Nakashima H, et al. Deep learning analyzes Helicobacter pylori infection by upper gastrointestinal endoscopy images. Endoscopy International Open. 2018;6 :E139-E144. DOI: 10.1055/s-0043-120830 - 45.
Shichijo S, Nomura S, Aoyama K, et al. Application of convolutional neural networks in the diagnosis of Helicobacter pylori infection based on endoscopic images. EBioMedicine. 2017;25 :106-111. DOI: 10.1016/j.ebiom.2017.10.014 - 46.
Yoshii S, Mabe K, Watano K, et al. Validity of endoscopic features for the diagnosis of Helicobacter pylori infection status based on the Kyoto classification of gastritis. Digestive Endoscopy. Jan 2020;32 (1):74-83. DOI: 10.1111/den.13486