Abstract
Ice cores drilled from glaciers and ice sheets provide a critical natural archive of current and past evidence of climate and environmental change, and subglacial rock holds evidence of past glacial extent. Current climate change is causing the demise of glaciers around the world; the scientific need to recover ice cores from mid-latitude glaciers is urgent before ice core records are lost to melt. Logistical access to uncertain ice sheet conditions is challenging. Retrieval of subglacial rock cores is needed for cosmogenic dating evidence of past sea level. This paper describes recent engineering advances in scientific drilling of ice and subglacial rock cores under conditions of current climate change. The successful efforts of the U.S. Ice Drilling Program to retrieve a surface-to-bedrock ice core from Quelccaya Ice Cap, Peru is described, along with the successful subglacial rock coring that retrieved the first meter-length bedrock cores underlying 509 meters of the Greenland Ice Sheet.
Keywords
- ice core
- bedrock core
- subglacial
- scientific drilling
- glaciers
- ice sheets
1. Introduction
The world’s glaciers and ice sheets are huge ice masses that have important effects on sea level, water supply, weather and climate; in addition, they serve as natural archives of evidence of past environment and climate due to the depositional nature of their formation. In high altitude and high latitude regions of the Earth, existing glaciers and ice sheets began their formation many thousands to several millions of years ago as snow in places so cold that snow melt rarely occurred. Over many years of snow accumulation, the weight of additional snow and metamorphic processes compacted the underlying snow into firn (old snow). Over time the firn further compacted and transformed into solid glacial ice. Due to glacial flow, the basal area of the glacier may have entrained sand or till. The bed of the glacier may be sediment or till that rests on bedrock of the landform. A schematic of a cross-section of a glacier is shown in Figure 1.

Figure 1.
Schematic illustration of a glacier or ice sheet cross section. Recent snow on the surface accumulates on older snow (firn) that becomes compressed into glacial ice. Basal ice may be mixed with sediment or till, and there may be wet or dry sediment, till, or rocks on the bedrock. Subglacial lakes are not depicted here, and the schematic is not to scale. (credit: IDP).
In addition to many individual alpine glaciers that exist in high-altitude sites worldwide, Earth has two enormous polar ice sheets that each cover millions of square kilometers of land: the Greenland Ice Sheet, which is now more than 3 km thick in the center, and the Antarctic Ice Sheet, that is as much as 6.4 km thick in some places. Both ice sheets have many named glaciers that slowly drain ice toward the coast. Scientific discoveries based on evidence of past climate that is embedded in glaciers and ice sheets have revolutionized the field of climate science and enabled understanding of current climate conditions in the context of recent and distant past climate.
Science and engineering are inextricably linked in glacial endeavors. Retrieval of ice cores from glaciers and ice sheets requires highly specialized ice drilling engineering. While many nations have institutions that conduct ice drilling, the U.S. Ice Drilling Program (IDP) is funded by the National Science Foundation to lead integrated planning for ice drilling and coring and to provide the expertise and drilling technology needed to advance ice core and subglacial science. This chapter briefly describes the nature of climate evidence held within and beneath glaciers, scientific drivers of several current societally-relevant projects, and engineering endeavors by IDP that enable discoveries even as the world’s glaciers and ice sheets are changing under current climate warming.
2. Glaciers: an archive of evidence about environment and climate
In cold, high-latitude and high-altitude regions where snow does not melt, annual snowfall can accumulate over hundreds of thousands of years on the polar ice sheets. The snow crystals contain water isotopes and chemical impurities that hold clues to atmospheric conditions of their origin. As recent snow accumulates, the underlying snow from years and decades in the past, called firn, becomes compressed and undergoes post-depositional metamorphism over depths that range from 10 to 120 meters. In so doing, the interstitial space between the snow crystals becomes constricted until the remaining air finally becomes trapped in bubbles in solid ice; the air in the bubbles contains direct evidence of atmospheric composition. Over many thousands of years of accumulation, the glacial ice can become multiple kilometers thick. The ice and trapped gases near the bottom hold the oldest records while the snow at the top holds the youngest.
The accumulation process that formed glaciers and ice sheets also archives a record of environmental conditions. For example, stable isotopes of oxygen and hydrogen (oxygen-18 and deuterium) in an ice core were initially deposited as snow, and are indicators of past temperature variations. Moisture in the atmosphere has an isotopic composition related to the vapor source and transport pathways. As winds transport the moisture to an ice sheet, temperature-dependent isotopic fractionation occurs where heavier isotopes preferentially fall out as precipitation. The moisture that is deposited onto the ice sheet as snow eventually becomes archived as glacial ice, and the isotopes provide clues to the transport process as well as the temperature when the snow fell. In addition, chemical composition in snowfall provides a wealth of evidence including changes in atmospheric circulation, biological activity, volcanic eruptions, fire, and air pollution for example; such evidence becomes chronologically archived in the ice. The composition of the air in the bubbles provides direct evidence of past atmospheric composition, for example levels of carbon dioxide, methane, and other greenhouse gases. A description of stable water isotopic fractionation with examples of an ice core climate record from the Vostok ice core in Antarctica are provided in [1] and an overview of common chemical measurements for ice core records is provided in [2].
In consistently very cold locations that have high snow accumulation rates, glacial ice contains high-resolution annual records of past environment and climate that provide a critical past context for comparison with present conditions along with an understanding of Earth systems, including behavior of major atmospheric circulation patterns. For example, records from ice cores drilled at a high-altitude site on Mount Logan, the highest mountain in Canada, show evidence of the regional Hadley and Walker circulations in the Pacific [3], and stable isotopes indicate that shifts between modern and zonal atmospheric flow regimes occurred abruptly within a few years, with some coinciding with the end of the Little Ice Age and the beginning of the European Warm Period [4]. Mount Logan ice core records of soluble sodium, a proxy for the strength of the wintertime Aleutian Low, displays variability that shows strong similarities to tropical proxy records for the El Nino-Southern Oscillation [5].
In locations where snow melt occurs and the meltwater flows through the firn and ice, the chemical and isotopic evidence becomes mixed and blurred, and the natural archive can be destroyed. Scientists have documented surface area decrease in many glaciers, including four glaciers in Peru, Bolivia, equatorial east Africa, equatorial Papua, Indonesia, and the western Tibetan Plateau [6]. In that study the ice core records based on oxygen isotopic ratios (δ18O) show substantial impact of seasonal melt in the firn layers in ice cores from elevations below ~6000 m, however δ18O records recovered from higher altitude sites still contain well-preserved seasonal variations to the surface. Unfortunately, as climate warming continues, higher elevation glaciers will eventually also be degraded.
2.1 Drill before it’s gone: ice cores in a warming world
The polar ice sheets and alpine glaciers around the world are experiencing dramatic changes as they melt under current climate warming. According to the IPCC, in the two decades leading up to 2020 Greenland lost approximately 4890 Gt of ice and it is virtually certain that the Greenland Ice Sheet will continue to lose mass over the twenty-first century [7].
Because the high-resolution chemical and isotopic records archived in alpine glaciers provide patterns of regional climate variability over the many centuries to millennia, the demise of glaciers in temperate regions is removing irreplaceable clues to paleo regional climate patterns in the midlatitude regions of the planet. Originally formed during the most recent ice age and receding during the current interglacial cycle, many midlatitude glaciers have now stopped accumulating mass; on those glaciers the snow accumulated during the winter disappears that summer due to melt. Temperatures in the frozen depths of most midlatitude glaciers are gradually warming, with some now approaching the pressure melting point.
Glaciers in the central Andes Mountains of South America archive evidence of mid-latitude climate patterns that have a large impact on weather and human activity in the equatorial region and beyond. The Quelccaya Ice Cap in Peru, the largest remaining tropical ice cap in the western hemisphere, is a remnant of the large ice sheet that covered South America during the Pleistocene era. Quelccaya has had multiple retreats and advances since the Last Glacial Maximum about 20,000 years ago [8]. Near the summit of Quelccaya, ice cores were drilled to bedrock 2003; analysis of a core revealed precipitation patterns, volcanic activity, and climate variability at annual resolution over the past 1800 years along with shifts in the Intertropical Convergence Zone over this region of the Andes [9]. Ice drill engineering enabled a lighter weight drill with low fuel requirements for this remote location [10]. From shallow cores drilled in 2018, it has become evident that warming is causing meltwater percolation through the firn, washing out some climate evidence in the firn and ice near the firn-ice transition; however particle-based trace element records (e.g., Fe, Mg, K) retained well-preserved signals, yielding hope that another deep ice core from Quelccaya may offer additional discoveries [11]. The Quelccaya Ice Cap, which covered an area of approximately 50.2 km2 in 2018, may completely lose its accumulation zone before the end of the twenty-first century or sooner as the ice cap continues on a trajectory to disappearance [12]. Retrieval of a new surface-to-bedrock ice core from the summit region of Quelccaya before the glacier suffers further demise will retrieve glacial ice that can be preserved and used with new high-resolution chemical sampling techniques to enable additional climate discoveries.
Drilling ice cores in warm ice that is ice close to its pressure melting point is challenging. Commonly used cable-suspended electromechanical ice coring drills, which use a rotating cutting head with steel blades that cut an annulus around the core, can become stuck as ice that is close to the pressure melting point around the sonde melts and refreezes. At sites such as Quelccaya, melting an annulus around the core is the preferred coring method. Electrothermal drills are cable-suspended drills that have an electric powered heat ring at the drill head to melt through the ice. Photos of the drill head of the IDP Electrothermal Drill are shown in Figure 2a and the drill with an ice core in Figure 2b.

Figure 2.
(a) The heat ring serves as the drill head of the IDP Electrothermal drill (photo credit: IDP). (b) an ice core drilled with the Electrothermal drill during the 2023 field work at combatant col, Mt. Waddington, British Columbia, fieldwork (photo credit: Peter Neff).
Variants of electrothermal drill types have been used by ice coring programs in a number of nations; a summary of the applications can be found in [13]. The current IDP Electrothermal Drill is an upgrade of a thermal drill from the former Polar Ice Coring Office. The drill currently consists of the sonde with heated drill head, which is used in conjunction with the cable, winch and control system of the IDP 4-Inch Drill. An 1800 W (180 V) heat ring is located at the end of the sonde, as well as three spring-loaded blades called core dogs that are recessed in the core barrel wall and engaged at core break to hold the core in the barrel until it is unloaded at the surface. The system is used to collect two-meter length, 86 mm diameter, ice cores using power supplied by a 5 kW generator. To remove an ice core from the barrel, the drill is laid horizontally and the core dogs are retracted using three magnets. Disengaging the core dogs allows the core to be pushed out through the heat ring. Two prototype tools have recently been designed by IDP and deployed to assist with difficult drilling conditions. Any debris encountered in the borehole can limit or prevent heat transfer and penetration. A debris vacuum assembly consists of a vacuum head with internal one-way valves attached to a tube containing a spring-loaded piston. A trigger mechanism in the vacuum head releases the piston when the tool is set on the bottom of the borehole, sucking any debris smaller than approximately 3 mm in diameter up into the tube. In conditions where the risk of borehole refreezing is present, an ethanol deployment system consisting of a tube containing a long plastic bag filled with ethanol may be deployed. A sharp arrowhead on the end of a plunger arm is used to puncture the bag and deploy the ethanol at the bottom of the borehole. Successful ice core drilling requires many adaptations that are specific to the nature of the ice. The IDP Electrothermal Drill was used in 2022 by IDP to successfully retrieve an ice core from the surface down to bedrock at 128 m depth at the Quelccaya Ice Cap. Figure 3 shows the Electrothermal drill with its tent, and Figure 4 shows drill operations at Quelccaya.

Figure 3.
The IDP Electrothermal drill at the drill camp on Quelccaya ice cap (photo credit: Mariusz Potocki).

Figure 4.
(a) The Electrothermal drill sonde hangs on the drill tower and is lowered into the borehole (photo credit: Mariusz Potocki). (b) IDP engineer Elliot Moravec operates the drill on Quelccaya ice cap (photo credit: Mariusz Potocki). (c) the IDP Electrothermal drill operations are conducted in a Tentipi Safir tent (photo credit: Mariusz Potocki).
In addition to challenges with the drilling, there are significant logistical constraints on accessing the remote Quelccaya ice cap and retrieving ice cores. Commercial transportation is available to the city of Cusco, Peru. From there, one must contract with local logistics personnel for transport of the field team and equipment to the field site. Car transport moves gear and personnel from Cusco to Phinaya (16,600 ft) at the base of the ice cap. Starting in Phinaya, cargo is loaded onto horses for transport up the ice cap. A basecamp was established at 17,000 ft., at which point porters load and carry cargo to the summit at 18,600 ft., as depicted in Figure 5. The slow ascent to the summit of the ice cap requires multiple days of acclimatization along the way to adapt to the high altitude.

Figure 5.
Porters carry drill cargo, ice core boxes and field camp gear through fields of Penitentes ice formations up to Quelccaya ice cap (photo credit: Elliot Moravec).
Drilling operations at the Quelccaya site must be light and agile by necessity at this remote, high altitude site. The total camp is shown in Figure 6. Smaller tents are used for berthing while a larger tent is adapted to house the drill.

Figure 6.
Drilling camp on Quelccaya ice cap in 2022 (photo credit: Mariusz Potocki).
With scant resources at the site, the team measures, weighs and packs the core as it is drilled at the site, in preparation for transporting the core down the glacier. Figure 7 depicts scientists working with the core at the drill site.

Figure 7.
The science team measures and processes an ice core drilled on Quelccaya (photo credit: Mariusz Potocki).
The ingenuity, experience, and hardiness of engineers in the Ice Drilling Program and the Quelccaya field team has achieved the successful retrieval of an irreplaceable ice core from Peru. Chemical clues in the ice cores that may reveal environmental conditions and climate shifts in the midlatitudes pre-dating current anthropogenic climate warming are now stored at laboratories in the U.S., safely archived for science even as the glacier melts away.
3. Paleo sea level: evidence of glacial extent embedded in subglacial rock
Over long periods of time, a balance of water on Earth shifts between oceans and ice sheets on land. In cold glacial periods, ice sheets are huge and sea level is low, while in warm interglacial periods glacial extent is low, and sea level is high. Earth is now in an interglacial period with the Greenland Ice Sheet the only remaining part of the large Laurentide ice sheet that once covered Greenland plus what is now Canada and the northern tier of the U.S. in the previous glacial period. Current anthropogenic climate warming is causing faster melting of glaciers and ice sheets with associated sea level rise. With more than two-thirds of the world’s fresh water currently locked up as ice in ice sheets and glaciers, knowledge of past glacial extent can help to understand the future rise in sea level that we are facing.
Rock formations on lands that are now covered by glaciers but that were not covered during the previous interglacial period hold isotopic evidence of local glacial extent in the past, through phenomena associated with their exposure to the sun. Explosions on stars in outer space and on our sun emit cosmic rays, which are high energy particles that flow through space, some of which are constantly hitting the surface of the Earth. Cosmic rays cannot penetrate into a glacier however they can penetrate into the first meter of an exposed rock to create isotopes called cosmogenic nuclides. These radionuclides are produced at a known rate and they undergo radioactive decay at a known rate, so measuring the abundance of the nuclide in a rock sample can indicate how long the rock was exposed to the sun.
3.1 Subglacial geology and past ice extent: recent endeavors in Greenland
The formidable engineering challenges involved in retrieving subglacial bedrock cores have only been overcome very recently. While glaciers are composed of ice for the majority of the hundreds to thousands of meters of their thickness, the basal meters of glaciers are often complex in geometry and composition, sometimes being a mix of glacial ice, sediment, loose rock. There may or may not be cracks and cavities in that mixed region. Below the glacier, the ground may be wet or dry and may have sediment and surface rubble that overlie the bedrock. Retrieving sub-ice bedrock cores is complex and it requires a means of drilling down through the glacier, through mixed ice/rock media near the bottom of the glacier, and then into solid bedrock. A major challenge with drilling through glaciers and into the bedrock is the possibility of hydrofracturing the drilled hole if drilling fluid circulation pressure exceeds the strength of the ice. Drill fluid is circulated during subglacial coring to cool the drill bit and to remove cuttings from the borehole. If hydrofracture occurs, then the drilling fluid drains into the cracks in the ice, drill fluid circulation is lost, and drilling is stopped. Ice is inherently a brittle material, and cracks propagate quickly. Hydrofracture occurs naturally near the surface of ice sheets as surface meltwater streams enter cracks [14]. In a laboratory study, Chen and others [15] found that there is a relationship between the overburden pressure at the depth of fracture and the pressure required to cause fracture, and that small fissures significantly weaken the borehole in phenomena that are temperature dependent. Successful drilling in complex glaciers relies on innovations in engineering.
There have been past efforts by multiple nations to gather subglacial cores through a variety of means; Talalay [16] provides a history of efforts to retrieve samples from the sub-ice environment. When the sub-ice environment is till or soft sediment, ice core drills or hot water drills can be used to create an access hole through the ice, after which metal tubes can be driven into the till or soft sediment to retrieve a core, for example using gravity corers and piston corers. Gravity and piston corers have been successful in till and sediment, however they are unable to retrieve a core from bedrock. On land that is not glaciated, the minerals industry has long drilled rock cores using heavy rotary drilling with a pipe string. Based on that technology, the Rapid Access Ice Drill (RAID) system was designed in order to rapidly drill through thousands of meters on ice sheets [17, 18]. This system will be appropriate for drilling through kilometers of ice as part of a heavy traverse of an ice sheet, however the components are too large to be transported to remote sites by small or midrange aircraft.
Scientific interest in determining past ice extent and sea level often focuses on locations near bedrock outcrops and glacial margins on polar sites where the environment is severe and the logistics are scarce. Sites with limited logistics adds the additional constraint that the drilling system must be agile and portable. Recently there have been engineering breakthroughs in agile ice drilling [19]. Drilling meters of bedrock from beneath many meters of glacial ice using agile, aircraft-deployable drill systems has been pioneered by the U.S. Ice Drilling Program, who developed the ice-enabled Winkie drill [20] and the Agile Sub-Ice Geological Drill [21]. These unique, fully operational drills have been successful in drilling subglacial rock cores from remote sites in Antarctica and also recently in Greenland.
The ice-enabled Winkie drill is an adaptation of the Minex commercial Winkie rock drill, which is a lightweight, compact drill used by the minerals exploration industry to drill small rock cores on unglaciated lands. IDP upgraded various parts of the drill, including replacing the gasoline engine with an electric motor, and also adding ice augering and ice coring capability. The ice-enabled Winkie is capable of drilling through ice, rock, till, and mixed ice/rock systems at locations where there are not cavities or cracks in the ice, nor presence of liquid water. The drill requires pressurized drilling fluid circulation; if there are cracks or cavities in the ice, there would be a loss of fluid in the borehole which would end the drilling. The system can retrieve cores either 33.4 mm or 71.7 mm in diameter and 1.5 m long, and it is able to drill to 120 m depth with the smaller diameter configuration. When there is a significant depth of snow and firn above the glacial ice, a lightweight ice coring drill is used to create a pilot hole through the firn. The system is transportable by helicopter or light aircraft and it can be set up in 3 hours. A schematic of the drill is shown in Figure 8. A mixed-media core composed of ice, silt, and rock core that was produced by the ice-enabled Winkie Drill in Ong Valley, Antarctica is depicted in Figure 9.
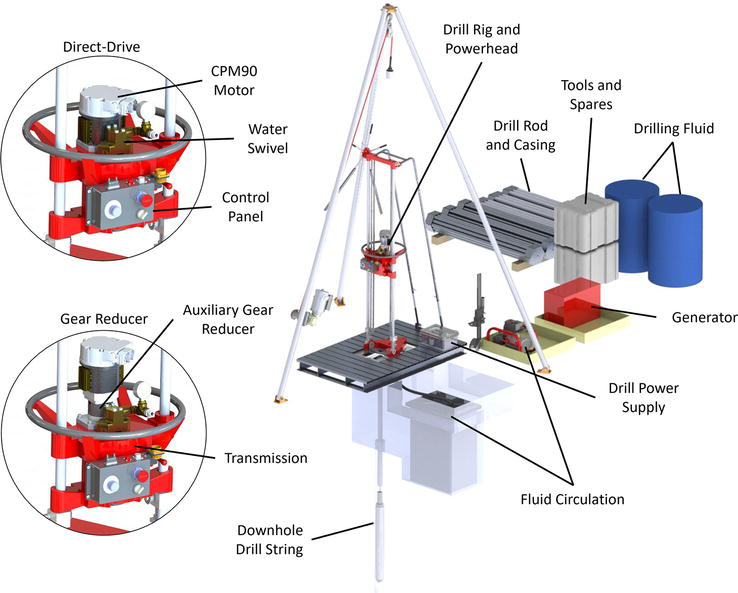
Figure 8.
Schematic of the ice-enabled Winkie drill. (credit: IDP).

Figure 9.
A mixed media core showing ice, silt, and rock that was drilled using the ice-enabled Winkie drill (photo credit: Grant Boeckmann).
The small footprint and relative agility of the ice-enabled Winki drill facilitates its use in drilling through several hundred meters of ice to retrieve meters of subglacial rock core. Figure 10 shows the drill in operation in northwest Greenland in 2023.

Figure 10.
The ice-enabled Winkie drill site at the 2023 GreenDrill project in NW Greenland (photo credit: Elliot Moravec).
IDP used the ice-enabled Winkie to drill in 2023 in northwest Greenland at a site that is 25 m north of the divide at Prudhoe Dome as part of the GreenDrill project. There the drill performed well through 96 m of ice to retrieve 2 m of frozen subglacial sediment. Twenty days were spent at the Winkie site including setup, takedown, 5.5 days of coring operations, four inactive days due to severe weather, and time spent drilling through the challenging subglacial environment. In total, more than 2500 m of drill rod was tripped in and out of the hole in working to clear and change the bits. A new full face ice bit was used to rapidly penetrate through the glacier ice and access the bed. A new slip foot clamp was designed by IDP and deployed as well as a capstan winch to assist with rod tripping. A new full face drag bit was utilized to quickly penetrate through dirty ice. As the system is operated out in the elements, considerable time was spent digging out the drill site each day and removing snow from the capstan winch, mud pumps and fluid filtration system. Tents to shield components and operations from blowing snow are now being implemented for the next field season. Drilling in severe conditions with cold temperatures and blowing snow, along with scarce logistics requires taking a variety of tools and equipment that may be needed for rapidly changing environmental conditions. Figure 11 shows a rock core drilled using the ice-enabled Winkie at the site.

Figure 11.
Core from the ice-enabled Winkie drilling near Prudhoe dome (photo credit: Jason Briner).
While the ice-enabled Winkie drill can be used at shallow sites, drilling through thicker ice with bedrock coring is accomplished using the IDP Agile Sub-Ice Geological Drill (ASIG), which is capable of drilling through 700 m of glacial ice and mixed media to retrieve up to 10 m of bedrock core that is 39 mm in diameter. The IDP ASIG drill is the first proven system in the world with this capability. The development of the ASIG Drill is described in [21]. A schematic of the layout for the ASIG drill is shown in Figure 12.
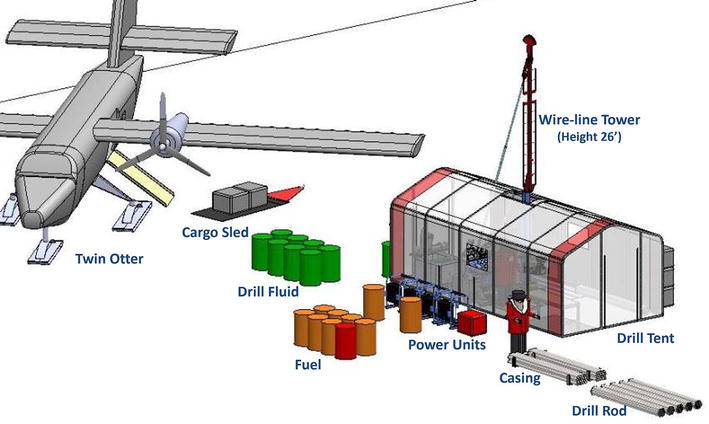
Figure 12.
Layout of the ASIG drill (credit: IDP).
The ASIG Drill is based on a customized Discovery MP1000 Man Portable Diamond Core Drill made by Multi-Power Products Ltd. in Kelowna, BC, Canada. The system uses four Kubota diesel engines, Sandvik WL56 thin-kerf drill rod and Sandvik TK56 wireline coring components. An inflatable packer is used to create a seal between the casing and the ice wall of the borehole, preventing drilling fluid from flowing up into the permeable firn above the packer. An isoparaffinic Naphtha fluid called Isopar K is used as a drilling fluid to transport cuttings to a filtration system on the surface. Polycrystalline diamond compact (PDC) full face bits are used for access drilling. An electronic pressure relief valve (PRV) was implemented by IDP to safeguard against over pressurization of the system and reduce the risk of hydrofracture of the ice. Despite such safeguards, hydrofracture events have occurred during use of the drill in both Antarctica and Greenland. In Antarctica, the drill was moved and a new hole initiated and completed. In Greenland, IDP engineers were able to remove the casing and packer and deepen the pilot hole several meters using an ice coring drill and then resume drilling.
Both the IDP ASIG and the ice-enabled Winkie drills have been successfully used to retrieve subglacial sediment and rock cores. Briner and others [22] conducted investigations to identify regions of the Greenland Ice Sheet where the ice sheet is less than 700 m thick and the bed of the ice sheet is likely to be frozen with underlying quartz-bearing rock lithologies that are amenable to cosmogenic nuclide analysis. They identified regions in northern, northwestern, and northeastern Greenland that meet the criteria and thus are likely to hold evidence of whether the ice sheet disappeared in past interglacial times 420,000 and 120,000 years ago, along with the rate of melt.
In 2023, IDP used the ASIG Drill in the Prudhoe Dome region of Greenland. Sixty thousand pounds of drilling equipment, drilling fluid, fuel, camping gear and a 10-person team were flown by Basler aircraft and helicopter from Pituffik Space Base in NW Greenland to the field site. Fifty-one days were spent onsite, including 34 days of drilling activity, and 12 days of poor weather that limited drilling, along with several days spent troubleshooting. A hydrofracture occurred just below the packer. The casing and packer were then reset and drilling resumed, resulting in the collection of 3.5 m of subglacial sediment and 4.5 m of gneiss bedrock core from under 509 m of ice. Normal versus reverse fluid circulation reduced borehole pressure at the packer and will likely be used during future ASIG drilling campaigns. The ASIG drill tent is seen at the 2023 Prudhoe Dome drill camp in Figure 13.

Figure 13.
ASIG camp at Prudhoe dome Greenland in 2023 (photo credit: Tanner Kuhl).
The tent designed for use with the ASIG drill is relatively lightweight yet provides protection from blowing snow; this facilitates continued drilling during bad weather. The ASIG drill in operation is shown in Figure 14. A tray of subglacial rock core retrieved by IDP engineers using the ASIG drill at Prudhoe Dome is shown in Figure 15.

Figure 14.
ASIG drill operations in NW Greenland in 2023 (photo credit: Elliot Moravec).

Figure 15.
Subglacial rock core from the ASIG drilling at Prudhoe dome (photo credit: Allie Balter-Kennedy).
4. Conclusions
Glaciers and ice sheets are unique natural archives of current and past evidence of climate and environmental change. Analysis of the evidence in ice core has led to many important discoveries, for example that the climate can change abruptly in less than 10 years [23], and the realization that direct evidence from samples of ancient air in ice cores indicates that levels of carbon dioxide now in our atmosphere are higher than they have been in at least 800,000 years [24, 25]. Retrieval of ice cores from remote locations requires highly specialized engineering, both for development of appropriate drills and for expert use of the drills in a medium as complex as a glacier and the subglacial environment. As current climate change is causing the demise of glaciers around the world and increasing sea level, the need to retrieve scientific evidence from within and beneath glaciers and ice sheets is urgent. The U.S. Ice Drilling Program (IDP) has recently succeeded in ice drilling achievements that are enabling cutting-edge science. Drilling the Quelccaya, Peru ice core from surface to bedrock using the IDP Electrothermal Drill on this remote, high-altitude glacier successfully preserved an ice core that is a natural archive of mid-latitude environment and climate over the past several thousand years, even as the glacier itself is on a trajectory of demise due to melt. In addition, IDP pioneered development of the first proven drilling systems to retrieve rock and sediment cores from beneath hundreds of meters of glacial ice, the Agile Sub-Ice Geological (ASIG) Drill, and the smaller ice-enabled Winkie drill. In 2023, IDP engineers successfully recovered the first meters of rock cores from beneath 509 m of the Greenland Ice Sheet, cores that scientists will analyze to improve understanding of sea level during past interglacial times. The changing climate is creating urgency for new knowledge; engineering efforts of IDP are rising to meet the need, as evidenced by the successful IDP retrieval of the Quelccaya 2023 ice core and the first retrieval of meters of bedrock core from beneath 509 m of the Greenland Ice Sheet.
Acknowledgments
The work of the U.S. Ice Drilling Program (IDP) is supported by the National Science Foundation under Cooperative Agreement No. 1836328 to Dartmouth and sub-awards to the University of Wisconsin and the University of New Hampshire. The authors thank anonymous reviewers for their constructive comments.
References
- 1.
Souchez R, Lorrain R, Tison J-L. Stable water isotopes and the physical environment. Belgeo. 2002; 2 :133-164. DOI: 10.4000/belgeo.16199 - 2.
Brook EJ. Ice core methods: Overview. In: Scott AE, editor. Encyclopedia of Quaternary Science. Vol. 2. Oxford: Elsevier; 2013. p. 278. ISBN: 9780444536426 - 3.
Moore GWK, Alverson K, Holdsworth G. Mount Logan ice core evidence for changes in the Hadley and Walker circulations following the end of the little ice age. In: Diaz HF, Bradley RS, editors. The Hadley Circulation: Present, Past and Future. Advances in Global Change Research, v 21. Dordrecht: Springer; 2004. DOI: 10.1007/978-1-4020-2944-8 14 - 4.
Fisher DA, Wake C, Kreutz K, Yalcin K, Steig E, Mayewski P, et al. Stable isotope records from Mount Logan, eclipse ice cores and nearby jellybean Lake. Water cycle of the North Pacific over 2000 years and over five vertical Kilometres: Sudden shifts and tropical connections. Géographie Physique et Quaternaire. 2004; 58 (2-3):337-352. DOI: 10.7202/013147ar - 5.
Osterberg E, Mayewski PA, Fisher DA, Kreutz KJ, Maasch KA, Sneed SB, et al. Mount Logan ice core record of tropical and solar influences on Aleutian low variability: 500-1998 A.D. Journal of Geophysical Research – Atmospheres. 2014; 119 :11,189-11,204. DOI: 10.1002/2014JD021847 - 6.
Thompson LG, Davis ME, Mosley-Thompson E, Porter SE, Corrales GV, Shuman CA, et al. The impacts of warming on rapidly retreating high-altitude, low-latitude glaciers and ice core-derived climate records. Global and Planetary Change. 2021; 203 :103538. DOI: 10.1016/j.gloplacha.2021.103538 - 7.
IPCC, 2021: Climate Change 2021: The Physical Science Basis. Contribution of Working Group I to the Sixth Assessment Report of the Intergovernmental Panel on Climate Change [Masson-Delmotte V, Zhai P, Pirani A, Connors SL, Péan C, Berger S, Caud N, Chen Y, Goldfarb L, Gomis MI, Huang M, Leitzell K, Lonnoy E, Matthews JBR, Maycock TK, Waterfield T, Yelekçi O, Yu R, and Zhou B (eds.)]. Cambridge, United Kingdom and New York, NY, USA: Cambridge University Press; 2021. pp. 33−144. DOI: 10.1017/9781009157896.002 - 8.
Kelly MA, Lowell TV, Applegate PJ, Smith CA, Phillips FM, Hudson AM. Late glacial fluctuations of Quelccaya ice cap, southeastern Peru. Geology. 2012; 40 :991-994. DOI: 10.1130/G33430.1 - 9.
Thompson LG, Mosley-Thompson E, Davis ME, Zagorodnov VS, Howat IM, Mikhalenko VN, et al. Annually resolved ice core records of tropical climate variability over the past ∼1800 years. Science. 2013; 340 :945-950. DOI: 10.1126/science.1234210 - 10.
Zagorodnov VS, Thompson LG. Thermla electric ice-core drills: History and new design optins for intermediate-depth drilling. Annals of Glaciology. 2014; 55 (68):322-330. DOI: 10.3189/2014AoG68A012 - 11.
Clifford HM, Potocki M, Rodda C, Dixon D, Birkel S, Handley M, et al. Prefacing unexplored archives from central Andean surface-to-bedrock ice cores through a multifaceted investigation of regional firn and ice core glaciochemistry. Journal of Glaciology. 2023; 69 (276):693-707. DOI: 10.1017/jog.2022.91 - 12.
Yarleque C, Vuille M, Hardy DR, Timm OE, De la Cruz J, Ramos H, et al. Projections of the future disappearance of the Quelccaya ice cap in the Central Andes. Nature. 2018; 18 (8):15564. DOI: 10.1038/s41598-018-33698-z - 13.
Bentley CR, Koci B, Augustin LJM, Bolsey RJ, Green JA, Kyne JD, et al. Ice drilling and coring. In: Bar-Cohen Y, Zacny K, editors. Drilling in Extreme Environments. Weinheim, Germany: Wiley-VCH; 2009. ISBN:978-3-527-40852-8 - 14.
Chandler DM, Hubbard A. Widespread partial-depth hydrofractures in ice sheets driven by supraglacial streams. Nature Geoscience. 2023; 16 :605-611. DOI: 10.1038/s41561-023-01208-0 - 15.
Chen C, Zhang H, Liu S, Jin C, Chen Y, Zhang N, et al. Hyrdraulic fracturing in ice boreholes: Theory and tests. Polar Science. 2019; 19 :40-48. DOI: 10.1016/j.polar.2018.10.003 - 16.
Talalay PG. Sublgacial till and bedrock coring. Cold Regions Science and Technology. 2013; 86 :142-166. DOI: 10.1016/j.coldregions.2012.08.009 - 17.
Goodge JW, Severinghaus JP. Rapid access ice drill: A new tool for exploration of the deep Antarctic ice sheets and sublacial geology. Journal of Glaciology. 2016; 62 (236):1049-1064. DOI: 10.1017/jog.2016.97 - 18.
Goodge JW, Severinghaus JP, Johnson J, Tosi D, Bay R. Deep ice drilling, bedrock coring and dust logging with the rapid access ice drill (RAID) at Minna Bluff, Antarctica. Annals of Glaciology. 2021; 62 (85-86):324-339. DOI: 10.1017/aog.2021.13 - 19.
Albert MR, Slawny KR, Boeckmann GV, Gibson CJ, Johnson JA, Makinson RKJ. Recent innovations in drilling in ice. In: Bar-Cohen Y, Zacny K, editors. Advances in Terrestrial Drilling: Ground, Ice and Underwater. 1st ed. Boca Raton, FL: CRC Press; 2021. pp. 157-220. ISBN: 9780367674861 - 20.
Boeckmann GV, Gibson CJ, Kuhl TW, Moravec E, Johnson JA, Meulemans Z, et al. Adaptation of the Winkie drill for subglacial bedrock sampling. Annals of Glaciology. 2020; 62 (84):109-117. DOI: 10.1017/aog.2020.73 - 21.
Kuhl T, Gibson C, Johnson J, Boeckmann G, Moravec E, Kristina SK. Agile sub-ice geological (ASIG) drill development and Pirrit Hills field project. Annals of Glaciology. 2021; 62 (84):53-56. DOI: 10.1017/aog.2020.59 - 22.
Briner JP, Walcott CK, Schaefer JM, Young NE, MacGregor JA, Poinar K, et al. Drill-site selection for cosmogenic-nuclide exposure dating of the bed of the Greenland ice sheet. The Cryosphere. 2022; 16 :3933-3948. DOI: 10.5194/tc-16-3933-2022 - 23.
Alley RB. Ice core evidence of abrupt climate changes. PNAS. 2000; 97 (4):1331-1334. DOI: 10.1073/pnas.97.4.1331 - 24.
Lüthi D, Le Floch M, Bereiter B, Blunier T, Barnola JM, Siegenthaler U, et al. High-resolution carbon dioxide concentration record 650,000-800,000 years before present. Nature. 2008; 453 :379-382. DOI: 10.1038/nature06949 - 25.
Brook E. Windows on the greenhouse. Nature. 2008; 453 :291-292. DOI: 10.1038/453291a