Compositions of blended fuels.
Abstract
The step-down of petroleum fuels has forced researchers to identify alternative fuels in the industrial and transportation sectors to satisfy energy demands. The most frequently used fuel alternative in compression ignition engines is methyl esters derived from vegetable oils. The present work aims to examine the performance, combustion, and emission characteristics of corn oil blends in a variable-compression-ratio engine. The Corn bio-diesel blends B20, B40, B60, B80, and B100 were compared with diesel for compression ratios of 13:1 and 14:1. The same blends were used in the experiments. The results of various parameters, such as brake thermal efficiency, specific fuel consumption, and emissions, showed that B100 had better outcomes than diesel. The average cylinder pressure and heat release rate compared with those of different blends and diesel at a compression ratio of 14 were also used in this study.
Keywords
- corn oil
- VCR CI engine
- combustion
- emission
- diesel engine
1. Introduction
Corn’s abundance and relatively simple conversion to ethyl alcohol (ethanol) make it a preferred feedstock for ethanol production in the United States. Although ethanol has been produced from corn and other high-starch cereals for thousands of years, the usage of these grains as fuel has increased significantly in the last century. Grinding, heating with enzymes, fermenting with yeast, and distilling to extract the water are all steps in the conversion process.
Two more processes are involved in fuel ethanol production: denaturing the ethanol to render it unfit for human consumption and utilizing a molecular sieve to extract the remaining water. After trans-esterification, corn oil can be utilized as biodiesel in compression ignition (CI) engines. Impact of residual gas trapping on the emissions and part-load efficiency of a wet ethanol-fueled spark-ignition direct-injection engine [1]. Experimental research and simulation were conducted on the impacts of blending diesel with waste-cooked oil methyl ester. Mohamed et al. [2] have published on the performance and emission characteristics of diesel engines. Researchers examined the efficiency and burning properties of diesel engines running on mixtures of waste-cooked biodiesel and grape seed [3], and the effects of changing the WCO/LDO blending ratio on exhaust emissions, combustion efficiency, and flame characteristics were studied. The range of the blending ratio was 0 to 100%. The equivalency ratio for each blend ranged from 0.6 to 1.05. The studies were carried out inside a cylindrical combustor that was cooled by water and equipped with a waste-oil burner that was positioned coaxially [4]. It has been documented that the combustion and emission properties of various generations of biodiesel can affect the performance of a compression ignition engine [5]. Using an electronic fuel fumigation approach, researchers have experimentally studied the performance and emission characteristics of compression ignition engines [6]. A single-cylinder compression-ignition (CI) engine was used for experimental research on pure diesel fuel and fuel oil-diesel fuel combinations. The engine’s performance, combustion, and exhaust emission characteristics were all studied [7]. Various diesel-biodiesel mixes and their glycerin emulsions have been examined for performance and emissions [8]. With a unit capacity of close to or above 200,000 tons/year, the most economical method of producing green diesel in a petroleum refinery looks to be catalytic hydroprocessing. Under supercritical circumstances, the profitability of conventional ester biodiesel and noncatalytic ester biodiesel processes is lower at a given capacity. Ten years into the project lifetime, the unit capacities of the examined processes—less than 100,000 metric tons annually—are probably going to produce negative net present values [9]. It has been shown that using renewable diesel fuels can assist address issues with urban air quality while also reducing particulate matter and climate-warming emissions from transportation [10]. Using Taguchi techniques and varying operating conditions, the performance of a tiny Kirloskar diesel in an internal combustion engine was investigated. The test engine’s NOx emissions were monitored with an AVL gas analyzer. Ramesh Babu et al. [11] examined how a thermal barrier-coated piston affected the operation and emissions of a diesel engine running on diesel fuel. Vidyasagar Reddya et al. [12] investigated the novel and distinctive acacia biodiesel’s engine performance and emission properties [13].
Examined how a biodiesel derived from palm oil and an antioxidant ingredient performed in engines and what kind of emissions it produced [14]. Measured and compared with plain diesel emissions were the emissions of carbon monoxide (CO), nitric oxide (NO), nitrogen dioxide (NO2), nitrogen oxides (NOx), hydrocarbons (HC), acetaldehyde, and formaldehyde from various mixtures. According to the test results, B20S2 has the lowest cloud point (34.8°C) of all the gasoline blends. Additionally, it was discovered that synergy could alter the biodiesel-diesel blend B20’s crystal structure and size [15]. This study examined the production, efficiency, combustion, and emission properties of diesel and corn oil combined in a VCR engine at various load levels.
2. Experimental setup and methodology
2.1 Corn biodiesel production and characterization
A nearby merchant in Hyderabad provided the corn oil. The free fatty acid (FFA) content of the maize oil was 4.75%, which was more than the permitted 2% level. A snapshot of the biodiesel production facility is displayed in Figure 1. Methanol and catalyst concentration (NaOH) were used in a transesterification process that lasted 60 minutes at 65°C. Bulk corn oil was made in accordance with these parameters.
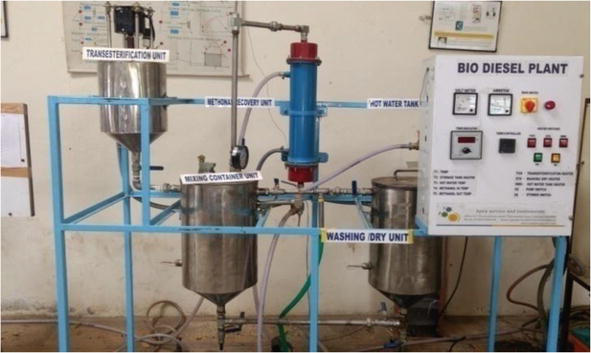
Figure 1.
Experimental setup of a bio-diesel producing plant.
To reach the required FFA content, more stirring was done for 20 minutes. After the required amount of biodiesel from maize oil was obtained, the glycerol, which can be used for further purposes, was separated from the biodiesel by gravity for a duration of 12 hours. In addition, the obtained biodiesel contained contaminants like NaOH and methanol. The elimination of these contaminants by hot distilled water washing of the sample is demonstrated in Figure 2. Once more, heating was done for 25 minutes at 100°C. Finally, a translucent, pale-yellow liquid was created that was based on corn for biodiesel. The diesel was mixed with various amounts of oil to test its miscibility. After fifteen days of observation, there were no indications of separation.
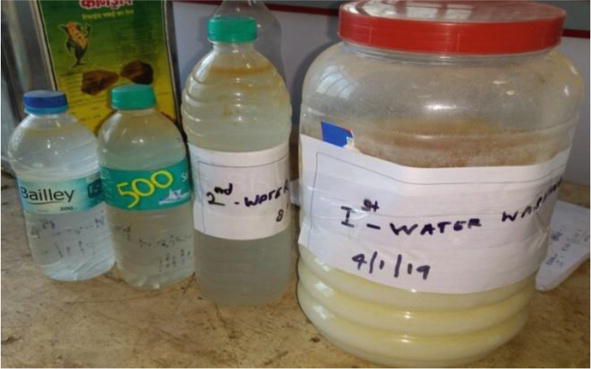
Figure 2.
The samples used to remove traces of methanol and NaOH.
The test samples (a total of 5) with a 1000 ml fuel each were named B20, B40, B60, B80, and B100 according to the reference diesel fuel (by volume) and their blending proportions of 20, 40, 60, 80, and 100%, as indicated in Figure 3. Tables 1 and 2 list the attributes of the combined maize oil and diesel, as well as the compositions of the blended fuels.
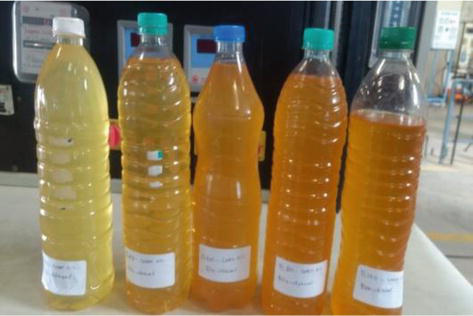
Figure 3.
The blended fuels B20, B40, B60, B80, and B100 used.
Blended fuels | Compositions |
---|---|
B20 | 20% Corn Oil +80% Diesel |
B40 | 40% Corn Oil +60% Diesel |
B60 | 60% Corn Oil +40% Diesel |
B80 | 80% Corn Oil +20% Diesel |
B100 | 100% Corn Oil |
Base Fuel | Diesel |
Table 1.
Properties | Diesel | Blended corn oil | Test protocol | ||||
---|---|---|---|---|---|---|---|
B20 | B40 | B60 | B80 | B100 | |||
Density (kg/m3) | 840 | 830 | 845 | 816 | 862 | 879 | IS 1448 (P-16), 1990 |
Calorific Value (MJ/kg) | 44.8 | 39.87 | 37.06 | 37.31 | 36.20 | 35.20 | IS 1448 (P-6), 1984 |
Flash Point (°C) | 50 | 56 | 58 | 60 | 61 | 60 | IS 1448 (P-21), 1992 |
Fire Point (°C) | 56 | 61 | 63 | 63 | 65 | 64 | IS 1448 (P-20), 1998 |
Kinematic viscosity (mm2/s) | 2 | 4.68 | 5.28 | 6.10 | 6.24 | 7.26 | IS 1448 (P-25), 1976 |
Pour Point (°C) | 6 | 3 | 4 | 4 | 4 | 5 | IS 1448 (P-10), 2012 |
Cetane Number | 50 | 59 | 63 | 57 | 69 | 63 | IS 1448 (P-19), 1989 |
Table 2.
Properties of fuel and test protocol.
2.2 Experimental setup and operating procedure
Figure 4 shows the experimental configuration employed in this investigation. An engine with a single cylinder and four strokes with a variable compression ratio was employed for the experiment. The cylinder’s clearance capacity was changed by pulling a lever, which alternated the compression ratio. A heat exchanger that served as a calorimeter was subjected to the exhaust gas. An exhaust gas analyzer called the AVL DI 444 was used to measure the emission parameters. An encoder fixed with a timing gear was used to monitor the crank angle, and a piezoelectric pressure sensor was installed on the cylinder head to measure the combustion pressure. Using a computer with a high-speed data gathering system, the parameters, including cylinder pressure, temperature, heat release rate, and so forth, were recorded at all the significant places. The experimental setup was preconditioned by running the VCR on diesel for fifteen minutes. Cooling water was supplied to the setup at a steady 100 LPH rate. As is customary, the engine was run for seven to ten minutes to stabilize it following any fuel blend changes. By mechanical injection, the fuel was injected at a temperature of 23° prior to the TDC and at a pressure of 200 psi. Brake power (BP), brake thermal efficiency (BTE), specific fuel consumption (SFC), and mass of fuel consumption were all calculated. Brake power (BP), brake thermal efficiency (BTE), specific fuel consumption (SFC), and mass of fuel consumption were all calculated. For every operating condition, measurements were made of the exhaust emission levels, combustion characteristics, and performance. Table 3 provides a list of the engine, pressure sensor, and exhaust gas analyzer specifications employed in the experimental inquiry.
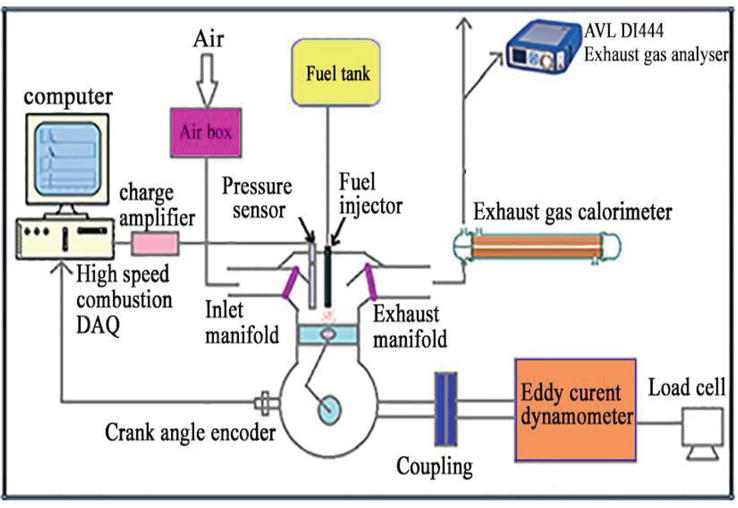
Figure 4.
Schematic of experimental setup [
Description | Specification |
---|---|
Variable compression ratio engine | Model: Kirloskar TV1, VCR, Single cylinder, 4-Stroke, water-cooled, stroke 110 mm, bore 87.5 mm |
Power | 3.5 kW |
Speed | 1500 rpm |
Injection Point variation | 0 to 25° BTDC |
Maximum load | 10 kg |
Injection pressure | 200 bar |
Compression ratio range | 12:1 to 18:1 |
Exhaust gas calorimeter | Shell and Tube, K-type thermocouple, water cooling |
Exhaust gas analyzer | AVL DIGAS 444 N |
Pressure sensor | Piezoelectric |
Table 3.
Specifications of the engine parameters.
2.3 Error and uncertainty analysis
The experimental uncertainty, instrumental error, environmental conditions, and parallax error were considered during the analysis. Various parameters, such as specific fuel consumption, brake thermal efficiency, and carbon monoxide, were calculated using the percentage uncertainty of the respective instruments. The total uncertainty of the experiment is calculated using the following equation:
Total percentage of the uncertainty = [(BP)2 + (BTE)2 + (CO)2 + (HC)2 + (O2)2+ (NOx)2 + (crank angle encoder)2 + (Load)2+ (pressure sensor)2 + (Speed)2 + (SFC)2]1/2. The obtained value of total uncertainty lies in the acceptable range of ±1.4%.
3. Results and discussion
3.1 Experimental results
Blends of corn biodiesel were tested. Diesel was contrasted with the outcomes for each of the five blends’ parameters. Blends B20, B40, B60, B80, and B100 have generally better emissions, specific fuel consumption, and brake thermal efficiency than diesel. The ensuing sections provide more details on the performance, combustion, and emission features.
3.2 Brake thermal efficiency
Brake thermal efficiency can be used to assess how well the fuel’s ignition quality is performing. Figure 5 displays the BTEs of the fuels that were evaluated at various loads and compression ratios. The graphic illustrates how the BTE is greatly increased by the decrease in heat loss and the rise in brake power. The break thermal efficiency for diesel at full load at a compression ratio of 14 was roughly 22.98%, which was little less than that of the B20, B40, B60, B80, and B100. When using blends of biodiesel instead of diesel, a notable rise in BTE was noted. Diesel evaporated quickly and was easily mixed with the blends to create a homogenous mixture, which contributed to the blends’ greater BTE. Diesel evaporated quickly and was easily mixed with the blends to create a homogenous mixture, which contributed to the blends’ greater BTE. The blends’ combustion characteristics are enhanced by the presence of oxygen. Diesel has a lower brake thermal efficiency (22.10%) than other mixes because of its low volatility, high viscosity, and low calorific value. All of these cause the fuel to burn poorly. The picture illustrates how the BTE of various blends of diesel grew when the CR (13 and 14) increased. One explanation for this could be that the tested fuels burn more efficiently at higher CRs due to greater compressed air temperatures.
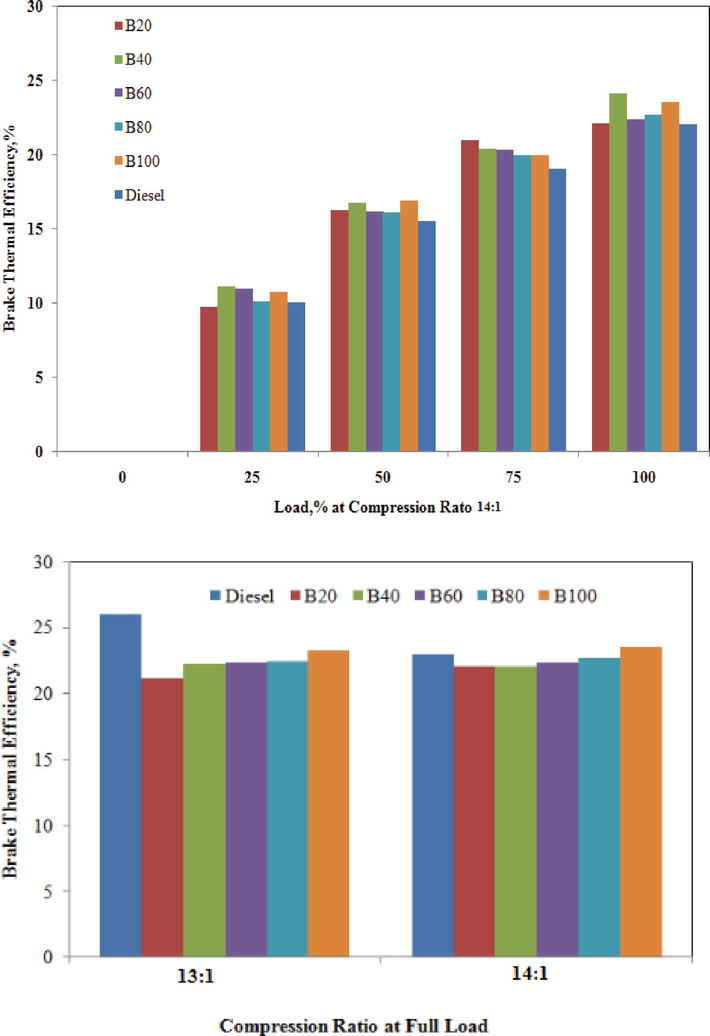
Figure 5.
Variations of brake thermal efficiency with load and compression ratio.
3.3 Specific fuel consumption
Fuel economy is determined by specific fuel consumption. Figure 6 displays the precise fuel consumptions of the tested fuels at various loads and compression ratios. For all fuels, as the braking power increased, the brake SFC dropped with increasing load. Relative to diesel, a smaller percentage of biodiesel blends (B20, B40, and B60) had no effect on the break of SFC. Diesel produced lower SFC than B80 and B100. By adding more oxygen and hydrocarbons to the blends, the rate of combustion was accelerated.
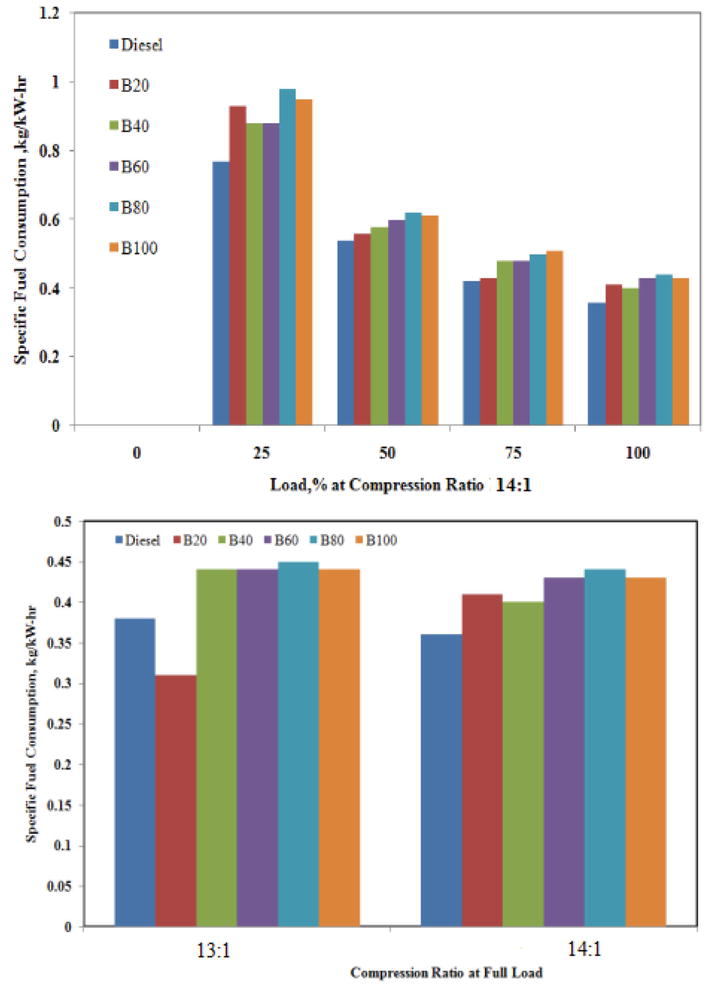
Figure 6.
Variations of brake SFC with load and compression ratio.
In comparison to other studied fuels, diesel’s high density and poor volatility led to lower SFC. The air-fuel combination becomes lean beyond the stoichiometric area, which leads to a progressive fall in SFC as CR increases. In a compression ignition engine, the mass of fuel used will decrease up to a 75% load.
Table 4 shows the comparison of brake thermal efficiency values and specific fuel consumption values of the fuels being tested. The brake thermal efficiency of the fuels is in descending order and vice versa for SFC.
Fuel | Brake thermal efficiency % | Specific fuel consumption kg/kW-hr | ||
---|---|---|---|---|
CR13 | CR14 | CR13 | CR14 | |
B20 | 21.12 | 22.1 | 0.31 | 0.41 |
B40 | 22.14 | 24.05 | 0.44 | 0.40 |
B60 | 22.17 | 22.38 | 0.44 | 0.43 |
B80 | 21.85 | 22.69 | 0.45 | 0.44 |
B100 | 21.95 | 22.54 | 0.44 | 0.41 |
Diesel | 22.23 | 22.98 | 0.38 | 0.34 |
Table 4.
Comparison of BTE and SFC at full load with variable compression ratio.
3.4 Carbon monoxide
Carbon monoxide is released when combustion is not completed. The tested fuels emit less CO at lighter loads and more CO at heavier loads as a result of the air-fuel ration decreasing with increasing load. The changes in CO emissions of the diesel and various blends operating at varied loads and compression ratios are displayed in Figure 7.
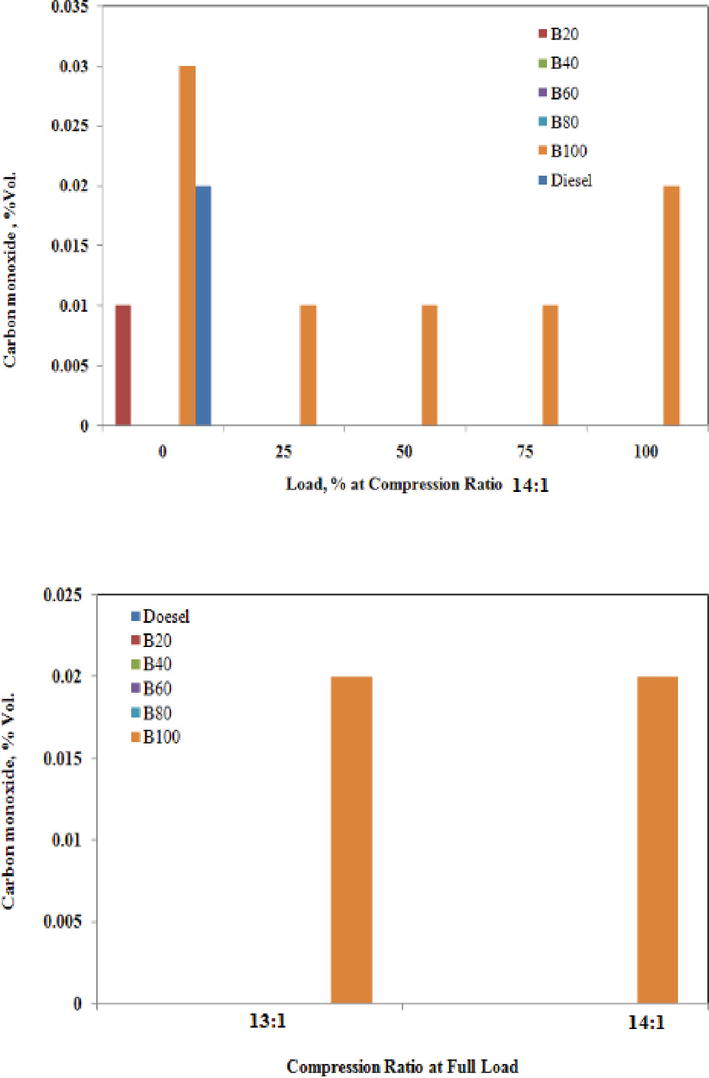
Figure 7.
Variations of carbon monoxide with load and compression ratio.
According to the figure, the engine produced greater CO emissions for B100 than for diesel and less for diesel. When compared to diesel (0.00% vol.) at full load, the CO emissions of B20 (0.01% vol.), B40 (0.00% vol.), B60 (0.00% vol.), B80 (0.00% vol.), and B100 (0.02% vol.) were shown to be significantly lower. Because to the poor atomization, increased viscosity, and low volatility in B100, the CO emission is higher (0.02% vol.). Because of increased pressure and compressed air temperature, a higher compression ratio results in a homogenous mixing of fuels, which lowers CO emissions.
3.5 Unburned hydrocarbons
The release of unburned hydrocarbons from an engine can be used to determine its performance. Low combustion temperatures, a weak flame, and fuel escaping from the flame zone are the main causes of HC emissions. The changes in HC emission with load and compression ratios are displayed in Figure 8.
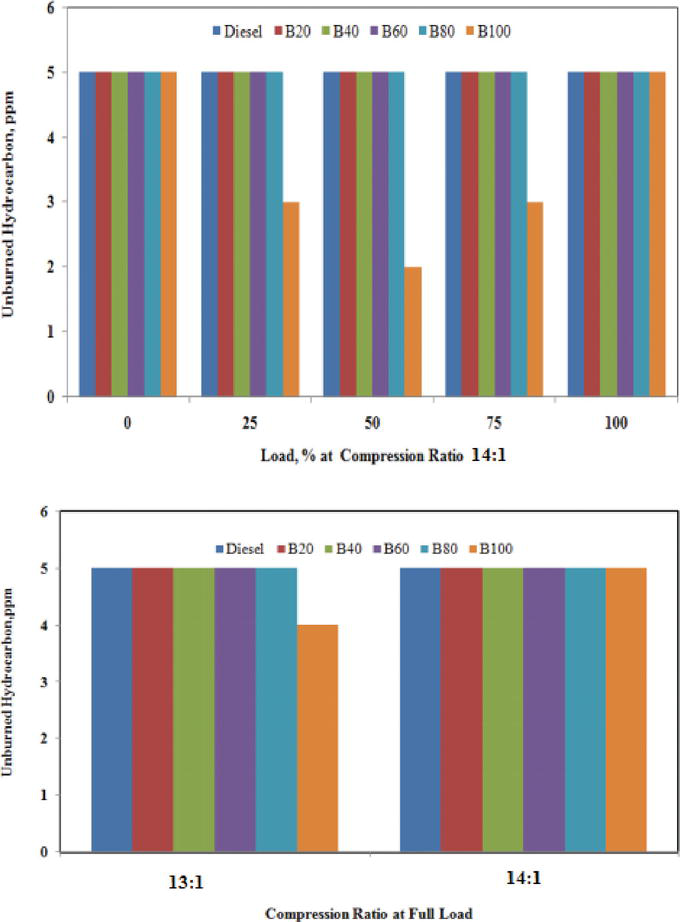
Figure 8.
Variations of unburned hydrocarbon with load and compression ratio.
In comparison to diesel fuel, it is seen that the HC emissions for blends (B20, B40, B60, B80, and B100) exhibit higher and lower values, respectively, at all loads. Increased density, atomization, and longer ignition delays cause fuel to build up in the combustion chamber, which could be the cause of higher-than-average HC emissions. The mixes B20, B40, B60, B80, and B100 increase combustion rates and enrich oxygen to lower greenhouse gas emissions. Because the HC emission for the tested fuels at full load shows a decreasing trend with regard to compression ratios, it is noticed that combustion is better at higher ratios.
3.6 Oxides of nitrogen
Nitrogen and oxygen react at high temperatures during fuel combustion to produce nitrogen oxide and nitric oxide, which are known as NOx emissions. Figure 9 displays the changes in NOx emissions with load and compression ratios for the different tested fuels. The NOx emissions rise as the load and compression ratio rise for each of the fuels under test.
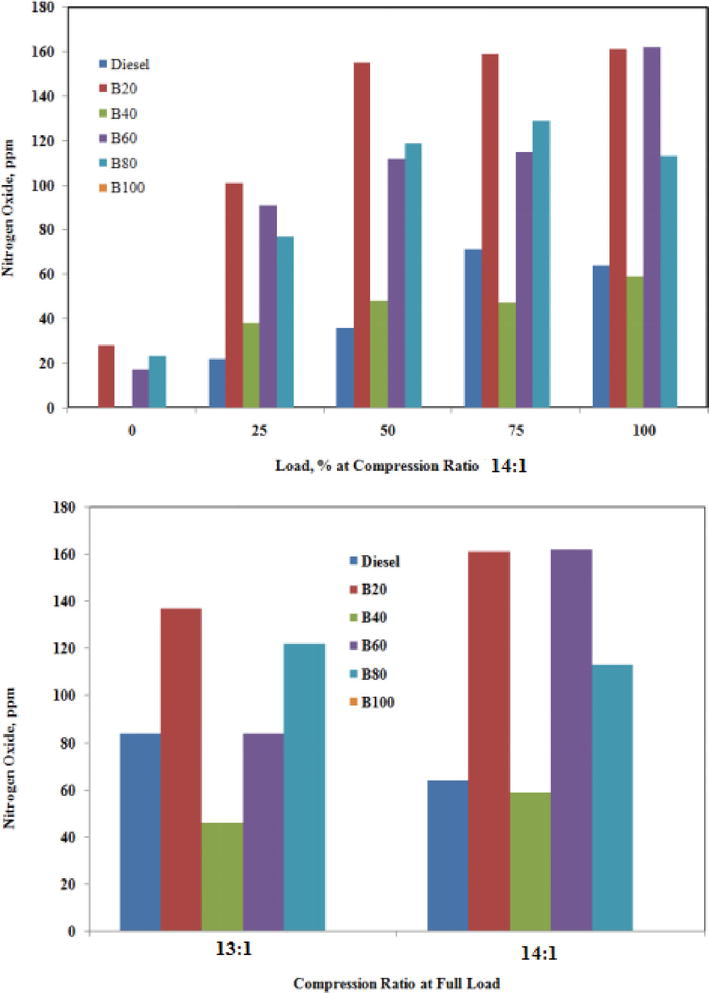
Figure 9.
Variations of nitrogen oxide with load and compression ratio.
Diesel and the blends (B40, B60, B80, and B100) were found to have lower NOx emissions as compared to B20. One possible explanation for the decreased amounts of NOx emissions could be the drop in flame temperature. Because of inadequate atomization and vaporization, incomplete combustion is the reason it is high for B20 fuel. Because to their increased oxygen concentration, which caused complete combustion, B20 blends emitted more NOx into the atmosphere than diesel did. Because of partial fuel combustion at a lower compression ratio, the NOx level is lower. Because of the increased pressure and temperature of the compressed air during the combustion process, which ensures complete combustion, NOx emissions are higher at higher compression ratios.
3.7 Combustion characteristics
The fuels’ volatility, viscosity, and rate of combustion all affect how high the cylinder pressure can get. Figure 10 displays the changes in mean cylinder pressure and heat release rate with crank angle. The graphic shows that the max cylinder pressure is lower at first and rises throughout the subsequent strokes. For the B20, B40, B60, B80, and B100, there is a little deviation in the mean cylinder pressure and heat release rate compared to diesel.
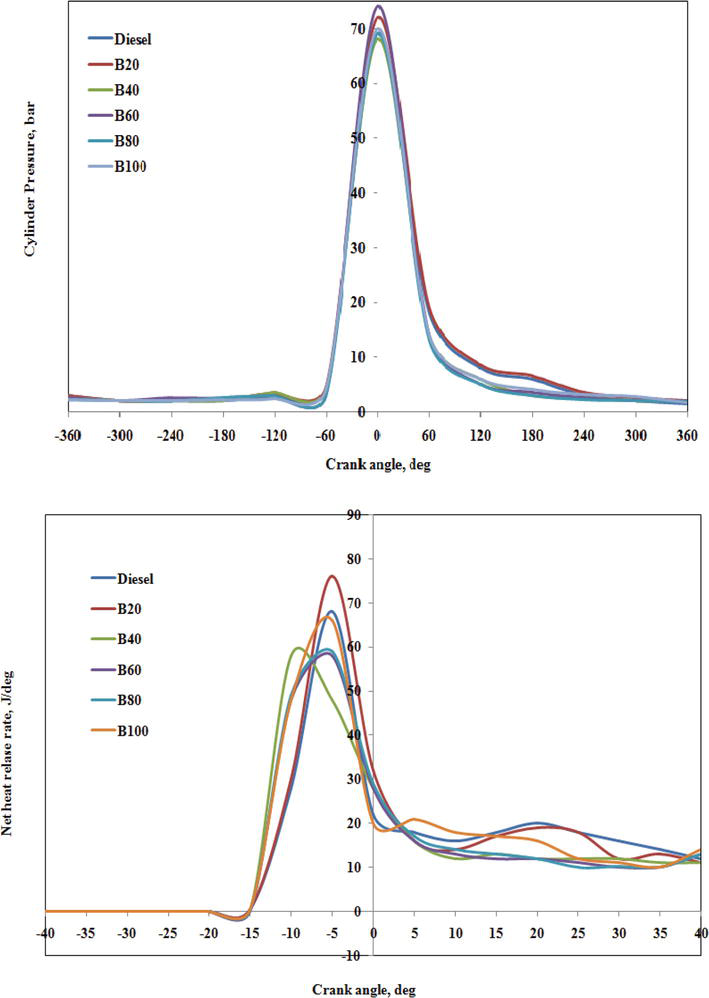
Figure 10.
Cylinder pressure and heat release rate with crank angle at CR of 14.
The fuel’s energy content has a direct impact on how quickly heat is released. The maximal heat release rate of corn biodiesel lowers as its ingredient content rises. Figure 10 shows that the heat release rate of diesel is higher (67.85 J/deg) than that of pure corn oil (65.08 J/deg) as a result of the lower flame temperature. Additionally, Figure 10 shows that B100 releases heat at a slower pace than the other fuels that were evaluated. B100’s increased viscosity causes the rates of fuel-air mixing to decrease.
4. Conclusions
In an experiment, different combinations of diesel and corn-based diesel (B20, B40, B60, B80, and B100) are utilized, and the compression ratio is adjusted. Blend B100 reaches a maximum BTE of 22.54%, which is higher than diesel, at full load and a compression ratio of 14:1. Furthermore, under full load, the B100’s SFC (0.41 kg/kWh) is higher than diesel’s (0.34 kg/kWh). With the lower blends, far less CO is being released. Biodiesel emits 0.02% more CO into the atmosphere than diesel, which emits 0.00% vol. Diesel and corn biodiesel have constant HC emissions across all loads, in contrast to mixed fuels. Compared to B100, diesel generates greater NOx emissions. Diesel releases heat at a faster rate (67.85 J/deg) than pure corn biodiesel (65.08 J/kg) because to its controlled combustion stage. Mixtures of methyl ester and corn biodiesel produce less emissions and better.
References
- 1.
Lanzanova TDM, Dalla Nora M, Martins MES, Machado PRM, Pedrozo VB, Zhao H. The effects of residual gas trapping on part load performance and emissions of a spark ignition direct injection engine fuelled with wet ethanol. Applied Energy. 2019; 253 (1):113-508 - 2.
Al-Dawody MF, Jazie AA, Abbas HA. Experimental and simulation study for the effect of waste cooking oil methyl ester blended with diesel fuel on the performance and emissions of diesel engine. Alexandria Engineering Journal. 2019; 58 :9-17 - 3.
Azad K, Rasul M. Performance and combustion analysis of diesel engine fueled with grape seed and waste cooking Biodesel. Energy Procedia. 2019; 160 :340-347 - 4.
Gad AMMS, El Fatih A, Emara A. Comparative study of combustion characteristics and exhaust emissions of waste cooking-diesel oil blends. Ain Shams Engineering Journal. 2018; 9 (4):3123-3134 - 5.
Rajak U, Verma TN. Influence of combustion and emission characteristics on a compression ignition engine from a different generation of biodiesel. Engineering Science and Technology an International Journal. 2020; 23 (1):10-20 - 6.
Vijayakumar M, Kumar PCM. Performance and emission characteristics of compression ignition engine handling biodiesel blends with electronic fumigation. Heliyon. 2019; 5 (4):e01480 - 7.
Akcay M, Ozer S. Experimental investigation on performance and emissioncharacteristics of a CI diesel engine fueled with fusel oil/diesel fuel blends. Energy Sources, Part A: Recovery, Utilization, and Environmental Effects. 2019. DOI: 10.1080/15567036.2019.1689317 - 8.
Sidhu MS, Roy MM, Wang W. Glycerine emulsions of diesel-biodiesel blends and their performance and emissions in a diesel engine. Applied Energy. 2018; 230 :148-159 - 9.
Glisic SB, Pajnik JM, Orlovic AM. Process and techno-economic analysis of green diesel production from waste vegetable oil and the comparison with ester type biodiesel production. Applied Energy. 2016; 170 :176-185 - 10.
Pirjola L, Kuuluvainen H, Timonen H, Saarikoski S, Teinila K, Salo L, et al. Potential of renewable fuel to reduce diesel exhaust particle emissions. Applied Energy. 2019; 254 :113-636 - 11.
Ramesh Bapu BR, Kumar JS, Shinde YN, Skaria DA. Emission investigation of VCR diesel engine by TiO2 nanocatalyst and Jamun biodiesel blended with diesel. International Journal of Ambient Energy. 2020; 43 (1):2673-2680 - 12.
Vidyasagar Reddya G, Rasua NG, Prasad TGH. Analysis of performance and emission characteristics of TBC coated low heat rejection engine. International Journal of Ambient Energy. 2021; 42 (7):2162-8246 - 13.
Babu JM, Sarath Chandra M, Ganesh PRC, Jayaprakash P, Kumar KS, Nagappan M. Experimental evaluation of direct injection diesel engine performance and emissions with acacia biodiesel. International Journal of Ambient Energy. 2022; 43 (1):7038-7045 - 14.
Harigaran A, Balu P. An experimental study on the performance and emission characteristics of a single-cylinder diesel engine running on biodiesel made from palm oil and antioxidant additive. International Journal of Ambient Energy. 2023; 44 (1):1076-1080 - 15.
Roy MM, Calder J, Wang W, Mangad A, Diniz FCM. Emission analysis of a modern tier 4 DI diesel engine fueled by biodiesel-diesel blends with a cold flow improver (Wintron synergy) at multiple idling conditions. Applied Energy. 2016; 179 :45-54 - 16.
Rajendran S, Govindasamy M. Effect of isopropyl alcohol on the performance, combustion and emission characteristics variable compression ratio engine using rubber seed oil blends. Energy Sources, Part A: Recovery, Utilization, and Environmental Effects. 2021. DOI: 10.1080/15567036.2021.1887408