Some commonly used deep eutectic solvent types.
Abstract
Deep eutectic solvents (DESs) are recently developed green solvents displaying characteristics, including simple production, reliability, chemical stability, low volatility, and biocompatibility that has caught the eye of researchers recently. This book chapter makes an in-depth review of the recent study on deep eutectic solvents, including its classifications, preparation methods, physicochemical characteristics, factors affecting DES pretreatment, and their applications. Based on the type of DES, molar ratio, type of biomass, reaction time, and reaction temperature, DESs can modify lignin characteristics and satisfy certain criteria. In addition, in this review, an investigation of the function of DESs in lignocellulose pretreatment and lignin isolation was also included. DESs dissolve lignin and provide extracted lignin with characteristics that make it suitable for further processing. This book chapter also discusses recent improvements in lignin extraction via biomass fractionation employing DESs and discusses DES-lignin valorization routes, depolymerization and functionalization.
Keywords
- deep eutectic solvent
- preparation techniques
- physicochemical characteristics
- lignin isolation
- valorizations of DES lignin
1. Introduction
Lignin is an abundant lignocellulosic biomass next to cellulose, which makes up about 30% of organic carbon on earth [1]. Despite the availability and properties, the recalcitrant nature of the lignin structure makes it the most underutilized lignocellulose [2]. Lignin can be used for the production of various chemicals, bioenergy, biomaterials, polymers, and additives, to name a few [3]. However, a significant amount of lignin is left as waste or burned to generate heat [4]. Thus, from the biorefinery concept with a view to enhance the profitability, valorization of lignin with an efficient fractionation technique is the key to maximize biomass valorization and minimize waste [5].
The isolation of lignin from lignocellulosic biomass can be performed using solvents like dilute acid, alkali solvent, and organic solvents. However, these solvents are not suitable for the fractionation of lignin since they produce condensed lignin, despite the costs and environmental impacts [6]. Recently, researchers have investigated DES as the most efficient solvent for lignin isolation.
Deep eutectic solvents are green solvents prepared from binary or ternary combinations of different organic or inorganic chemicals, which are Lewis or Bronsted acids and bases, with anionic and cationic species through hydrogen bonding [7, 8], as shown in Figure 1. Because of its distinct and tailorable physicochemical qualities, such as low volatility, high solubility, biodegradability, cheap cost, environmental friendliness, and structural feasibility, deep eutectic solvent (DES) is considered as a new generation of green solvents and so on, and promoted their widespread usage in the effective delignification of various biomass feedstocks [9], as indicated in Figure 2.

Figure 1.
Some commonly used organic or inorganic chemicals as hydrogen bond donors (HBDs), hydrogen bond acceptors (HBAs), and as metallic salts for DES synthesis.

Figure 2.
Researches in the areas of deep eutectic solvent for lignin isolation.
Deep eutectic solvents are generally classified into four types: type (I, II, III), and type (IV), depending on the source of the complexing agent used.
The combinations of deep eutectic solvent type can be represented by the general equation
The preparation of DES can be carried out by heating, grinding, evaporation, freeze-drying, microwave, and ultrasound-assisted methods. Heating and grinding methods are more commonly used than recently developed methods to overcome time and energy consumption, such as microwave-assisted and ultrasonic-assisted synthesis methods [11, 12]. However, ultrasound-assisted preparation of DES is the simplest and fastest preparation method, which is also chosen for the isolation of lignin from natural lignocellulosic materials in short time [13].
Earlier, processing of lignocellulose lignin was seen as complex and difficult due to lack of effective lignin extraction techniques. Thus, the valorization of lignocellulosic biomass typically, lignin became a problem. To overcome these problems, researchers found DESs are efficient and green methods for the extraction of lignocellulosic biomass.
The aim of this book chapter is to assess the most recent works on deep eutectic solvent, including its description, properties, synthesis and characterization, its role in lignocellulosic biomass isolation, factors affecting the isolation of lignin using DES, and the valorization routes of DES lignin.
2. Deep eutectic solvents
2.1 Deep eutectic solvent classification
Deep eutectic solvents (DESs) can be categorized, based on their nature of complexing agents as (type I, II, III, IV, and type V), pH (acidic, basic, and neutral), and miscibility in water (hydrophobic and hydrophilic) [14], which help to differentiate one DES from others. The majority of DESs are categorized under the nature of the complexing agent, which can be represented by the general formula
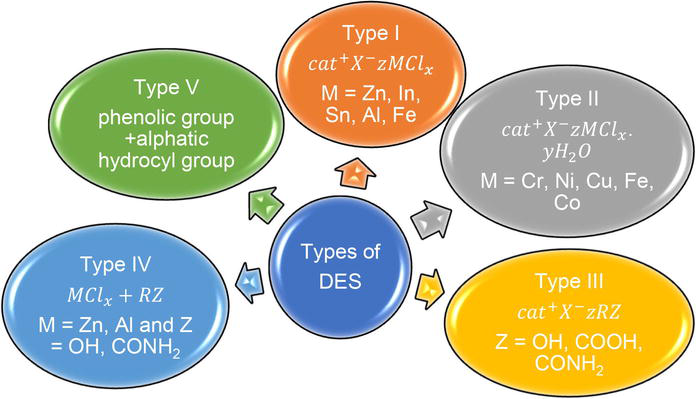
Figure 3.
Types and general chemical formula expression of DES.
Type of DES | Examples |
---|---|
Type I | ChCl: FeCl3, ChCl: CoCl2, & … |
Type II | ChCl: CrCl3. 6H2O, ChCl: CoCl2. 6H2O, & … |
Type III | ChCl: H2NCONH2, ChCl: CH3CONH2, & … |
NADES | ChCl: LAa, ChCl: Glyb, ChCl: CAc: H2O, & … |
Type IV | ZnCl2: CH3CONH2, ZnCl2: H2NCONH2, & … |
Type V | Phenanthroline: thymol, & … |
Table 1.
Lactic acid.
Glycerol.
Citric acid.
Type I DESs are combination of quaternary ammonium salt and metal chloride. This type of DES has high melting points due to non-hydrated metal halides, making Type I DESs difficult for the isolation of lignin. Whereas, Type II DESs are combination of quaternary ammonium salt and metal chloride hydrate. The differences between Type I DESs and Type II DESs are the type of metal halide, non-hydrated and hydrated metal halides, respectively. Thus, in biomass processing, Type I DESs have limited applications because of high melting points of non-hydrated metal halides, but Type II DESs are more practical for industrial operations due to the hydrated metal halides [8].
Type III DESs are combination of quaternary ammonium salt and hydrogen bond donor (HBD). The HBDs can be various types of carboxylic acids and this type of DES is mostly chosen for biomass pretreatment. Type III eutectics are the most studied types of DESs, which are typically synthesized from choline chloride (ChCl) and different hydrogen bond donors [15]. Choline chloride (ChCl), a quaternary ammonium salt, and amides, alcohols, and carboxylic acids are common hydrogen bond acceptors because of their low cost, high biodegradability, low toxicity, and bioavailability [11]. The DES could also be synthesized from a molecule that is typically safe and accessible, such as urea, organic carboxylic acids, and polyols [11]. With an ever-growing library of constituents estimated at 106–108 possible binary combinations of Type III DESs have been organized into subcategories based on their behavior or nature, which include hydrophobic DES [16], metal-free DES [17], and natural DESs [18].
Type IV DESs are combination of metal chloride and HBD such as urea and ethylene glycol [11, 12]. Lately, new classes of DESs made entirely of non-ionic, molecular hydrogen bond acceptors and hydrogen bond donors have been proposed as a novel DES type, which is Type V DES with the distinctive low melting point caused by strong hydrogen bonding [19]. In addition, natural deep eutectic solvent (NADES) type of DES was also developed, which was most likely similar to two type (III) DESs, the only difference being the solvents in NADES are synthesized from natural biomass (e.g., glycerol).
2.2 Potentials of deep eutectic solvent (DES)
One of the primary reasons for the growing research interest in deep eutectic solvents is their physicochemical properties, such as; low volatility, nonflammability, low vapor pressure, easy to prepare, biodegradability, and stability [7]. In addition, DESs are so tunable, which can be designed to form a number of different types of DESs. Understanding the fundamental properties of deep eutectic solvents is the crucial thing to prepare deep eutectic solvents and to use for different applications. Thus, the properties of DES are solvatochromic, physicochemical, electrochemical, and biological properties. The common characteristics of deep eutectic solvents are freezing point, melting point, density, viscosity, conductivity, surface tension, molar heat capacity, compensability, refractive index, polarity, and dielectric conductivity which are described in this book chapter [20]. Whereas some properties of DES such as toxicity, sustainability, and biodegradability were not considered since they were considered chemically green solvents [21].
2.2.1 Solvatochromic properties
Solvatochromic properties are the measure of DES effectiveness for fractionation of lignin using empirical parameter known as Kamlet-Taft (K-T) [4]. Kamlet-Taft parametric model is the quantitative tool that determines the properties of DES based on the parameters, such as hydrogen bond donating ability, hydrogen bond accepting ability, and bipolarity of DES; represented by α, β, and π*, respectively. The properties of these parameters depend on the nature of hydrogen bond donor (HBD) and hydrogen bond acceptor (HBA) [3]. Thus, to better understand the lignin solubility in DES, all the Kamlet-Taft parameters (α, β, and π*) have to be determined carefully. The higher values of α and β mean high hydrogen bond donating ability and high lignin fractionation ability [22]. Similarly, high values of β and π* dissolve high amount of lignin [23]. In addition, the solubility of lignin in DES also depends on bipolarity value (π*) and type of lignin [24].
In addition, Hildebrand and Hansen solubility parameters are also used to determine the solubility of lignin in DES using molecular dynamics (MD) simulation [25]. It is computed based on the projected infinite dissolution activity coefficient by molecular dynamics simulation, which assists in the selection of suitable DES for lignin isolation. Nowadays, the interaction between lignin and DESs, regulated by specific characteristics, is still underexplored. However, theories suggest that DESs can be modified by altering the variety of eutectic solvents. This could lead to a more efficient and desirable DES pretreatment system, allowing for the fractionation of lignin with desired qualities for subsequent valorization.
2.2.2 Physical, chemical, electrical, and biological properties of deep eutectic solvent
2.2.2.1 Freezing point
Freezing point, a temperature at which the liquid becomes solid at atmospheric pressure, is a determining parameter of DES. The freezing point of DES lies between −79 and 149°C [7]. Though, at the freezing point below 50°C solvents are more attractive, since it’s safe to use them and they are also inexpensive [26]. Factors, such as the type of ionic symmetry [8], alkaline chain length, strength of hydrogen bond [27], reaction temperature [28], molar ratio of hydrogen bond acceptor to hydrogen bond donor [29], the nature of hydrogen bond acceptor, the nature of hydrogen bond donor, and the nature of organic salt and its anion, affect the values of freezing point of the mixture [26]. In general, to prepare DES solutions for different applications, DESs with low freezing points are preferred. Low freezing points broaden the operating temperature range and facilitate the transmission of intermediary substances during separation processes [30]. For example, DESs with low freezing points are preferred for biomass processing applications, as they can remain as liquids for better mixing and mass transfer efficiency [31]. The freezing points of HBA and HBD are essential reference properties when designing DESs, as indicated in Table 2. The lower the freezing points of HBA and HBD of DES components, the deeper the decrease in the prepared DES.
HBA | Mp(°C) | HBD | mp(°C) | Molar ratio | DES Tf(°C) | Ref. |
---|---|---|---|---|---|---|
ChCl | 303 | Urea | 134 | 1:2 | 12 | [32] |
ChCl | 303 | Thiourea | 175 | 1:2 | 69 | [29] |
ChCl | 303 | 1,3-dimethyl urea | 93 | 1:2 | 29 | [29] |
ChCl | 303 | Citric acid | 149 | 1:1 | 69 | [32] |
ChCl | 303 | Adipic acid | 153 | 1:1 | 95 | [32] |
ChCl | 303 | Malonic acid | 134 | 1:1 | 10 | [32] |
ChCl | 303 | Oxalic acid | 190 | 1:1 | 34 | [32] |
ZnCl2 | 293 | Urea | 134 | — | 9 | [12] |
ZnCl2 | 293 | Acetamide | 81 | — | −16 | [12] |
Table 2.
A comparison of freezing point values of different deep eutectic solvents.
2.2.2.2 Density
Density is likely the most crucial property to identify the molecular mobility and activity of DES, which varies with the type of eutectic mixture. Most research studies showed that the density of deep eutectic solvents is greater than that of water, which is in the range of 1.0–1.3 g.cm−3 at atmospheric temperature. Whereas the densities of DESs obtained from metallic salts like zinc chloride (ZnCl2) are between 1.3 and 1.6 g.cm−3 [26]. In general, type I-IV DESs have higher density than water, whereas the density of type V DES is lower than that of water [9]. In addition, the density of hydrophobic deep eutectic solvent is lower than that of water changing between 0.889 and 0.93 based on the components [14].
The density of DES depends on the choice of the hydrogen bond donor and the molar ratio [33]. In addition, the density of deep eutectic solvent is temperature-dependent, which decreases linearly with increasing temperature [33]. Alomar et al. investigated the density of DES from glycerol. In the study, six different DESs were prepared taking glycerol as a HBD and six different salts, i.e., methyltriphenylphosphonium bromide (MTPB); benzyltriphenylphosphonium chloride (BTPB); allyl-triphenyl-phosphonium bromide (ATPB); choline chloride (ChCl); N, N diethylethanolammonium chloride (DAC); and tetra-n-butylammonium bromide (TBAB).
The measured density in the temperature ranges of 298–363 K showed the highest density 1.2965 g/cm−3 at 298 K [34]. Whereas, Hayyan et al. characterized physiochemical properties such as density, viscosity, refractive index, surface tension, and pH of glucose-based deep eutectic solvents. The results revealed that DES densities are above 1.20 g/cm3. In general, we can say the density of DES decreases as the temperature increases, due to the increased activity and molecular mobility [35]. However, the density of DES may also vary with the type of DES component and molar ratio, as shown in Table 3. As a result, the density of DES should be optimized to fit lignin fractionation.
DES (HBA: HBD) | Molar ratio | T(K) | Density (g/cm3) | Ref. |
---|---|---|---|---|
ChCl: urea | 1:2 | 313.15 | 1.24 | [36] |
298.25 | 1.212 | [36] | ||
MTPB: GLY | 1:3 | 363 | 1.2513 | [34] |
BTPC: GLY | 1:16 | 363 | 1.1968 | [34] |
ATBP: GLY | 1:14 | 363 | 1.2201 | [34] |
ChCl: GLY | 1:2 | 363 | 1.1548 | [34] |
DAC: GLY | 1:2 | 363 | 1.1342 | [34] |
TABB: GLY | 1:4 | 363 | 1.1064 | [34] |
ChCl: D-glu | 1:1 | 358 | 1.2678 | [35] |
Table 3.
Densities of different deep eutectic solvents.
2.2.2.3 Viscosity
One of the essential and well-studied characteristics of DES is viscosity. A number of researchers have described that the viscosity of DES is being very viscous at room temperature (> 100 mPa.s.). The reason behind the high viscosity of DES can be due to the hydrogen bond network that exists between the components of DES, as indicated in Table 4. However, deep eutectic solvents may present a broad viscosity range from low viscosity to very high viscosity. For instance, choline chloride: ethylene glycol has low viscosity, whereas sugar-based DESs have very high viscosity (12,730 mPa.s for 1:1 ChCl: Sr. (sorbitol) at 30°C and 34,400 mPa.s for 1:1 ChCl: glu (glucose) at 50°C), and metal salts-based deep eutectic solvents have even higher viscosities (85,000 mPa.s for 1:2 choline chloride: zinc chloride at 25°C) [26].
DES (HBA: HBD) | Molar ratios | T(K) | Viscosity (mpa s) | Ref. |
---|---|---|---|---|
ChCl: urea | 1:2 | 303.15 | 152,449 | [29, 37] |
ChCl: GLY | 1:2 | 303.15 | 190,246.79 | [38, 39] |
ChCl: EG | 1:2 | 303.15 | 35 | [40] |
ChCl: EG | 1:2 | 298.15 | 37 | [41] |
ChCl: MA | 1:1 | 303.15 | 982.9,464,608 | [42, 43] |
ChCl: OA | 1:1 | 313.15 | 1881,2142,202 | [42, 43] |
ChCl: LV | 1:2 | 303.15 | 164.5 | [42] |
Table 4.
Different types of deep eutectic solvents with their viscosities.
Factors, such as electrostatic interactions, chemical composition of the DES component, reaction temperature, and amount of water, all this affect the viscosity of deep eutectic solvent [7]. In addition, the experimental methods, sample preparation, and the impurities also affect the viscosity of deep eutectic solvent [42]. DES with high viscosity is not favorable for lignin fractionation. Thus, the development of DESs with low viscosities is particularly sought for lignin isolation. Using the hole theory model, it’s possible to prepare low viscosity deep eutectic solvents, in addition to using fluorinated HBD and small cations.
2.2.2.4 Conductivity
Conductivity is the measure of DES strength for conducting electricity. The value of DES conductivity depends on the viscosity of DES and other properties of DES. The hydrogen bond acceptor to hydrogen bond donor molar ratio, the type of both the organic salt and the hydrogen bond donor, as well as the anion of the salt [36], and the imbibition of water are the factors that affect conductivity of deep eutectic solvents [44]. In addition, the quantity of charged compounds and their mobilities influence DES conductivity. For instance, the conductivity of a deep eutectic solvent is inversely proportional to the viscosity of DES. Since deep eutectic solvents are mostly viscous, it implies low ionic conductivity, which is a problem for electrochemical applications [45]. As a result, raising the temperature reduces viscosity while increasing conductivity [45]. Generally, DESs display lower conductivity that is much lower than 2 ms cm−1.
Thus, microwave irradiation has recently been widely used to enhance lignin fractionation. When microwave heating is used, DESs with high electrical conductivity and dielectric constant effectively delignify lignocellulosic biomass by molecular collisions. Furthermore, for electrochemical transformation of lignin in adequate DES conductivity is required. The electrical conductivity of DESs can be tailored by optimizing the DES constituents and their molar ratios.
2.2.2.5 Surface tension
Surface tension is a measure of the cohesive forces in a liquid on its surface and a measure of the inherent energy between liquid molecules. It is a critical physical quality for the determination of a variety of applications, such as wettability, mass transfer capacity for liquid-liquid or gas-liquid extraction, and multiphase homogeneous catalysis. Surface tension is greatly influenced by the intensity of intermolecular interactions between the HBDs and the appropriate salt. For most of the examined systems, the surface tension of DES is exceptionally high. The values relative to the reported deep eutectic solvents generally vary between 35 and 75 mN/m at 25°C [36].
The surface tension of deep eutectic solvents is affected by the extra hydroxyl group or a longer alkyl chain in the quaternary ammonium salt, the salt mole fraction, cation type, and temperature [31]. The surface tension increases linearly with decrease in temperature [46] and it also decreases with increasing salt mole fraction due to the weakening of the hydrogen bond donor bonding network during mixing [36]. To understand further the variation of surface tension with different types of DESs, molar ratios at different temperatures are indicated in Table 5 below.
2.2.2.6 Polarity
Polarity is an important property for determining the solubility of a deep eutectic solvent measured by solvatochromic parameters [50]. The most commonly used polarity scales of Reichardt and Dimroth’s are normalized ET scale [50] and the multiparameter scale of Kamlet-Taft (α, β, and π*) [51, 52]. However, Abbott and colleagues reported the first attempts to investigate the solvation processes in deep eutectic solvents for eutectic mixes of choline chloride and glycerol.
Abbott et al. characterized the solvent polarities of ChCl and glycerol at different molar ratios using the indicated methods. The measured polarity of DES by ET [29] polarity scale showed that ChCl and glycerol DESs are polar fluids. These polarity scale ET [29] values of ChCl and glycerol are comparable to those of known polar HBD solvents. The reported result showed the change in polarity scale ET [29] values with increasing ChCl from 57 kcal/mol for pure glycerol to 59 kcal/mol for pure ChCl (extrapolated) with an almost linear trend [53]. Though, it’s understood that to compare polarity parameters obtained by different solvatochromic probes is so difficult as the polarity scales are not universal and are probe dependent [54, 55, 56].
3. Synthesis and characterization of deep eutectic solvent
See Figure 4.

Figure 4.
Chemical reaction followed for the synthesis of DES from commonly used type 3, HBA and HBD as an example.
3.1 Deep eutectic solvent synthesis
Deep eutectic solvent (DES) is synthesized by simply reacting hydrogen bond donor (HBD) and hydrogen bond acceptor (HBA) at specified molar ratios, as shown in Figure 4. During the preparation of DES, factors such as the water content and purity of individual components of HBA and HBD, as well as the storage and drying time of the prepared DES, should be consistently considered. The presence of these factors leads to variations in the properties of DES and preparation. Since most components of HBA and HBD absorb moisture in the atmosphere and disrupt the physiochemical properties of DES, furthermore, it is important to note that reagents and DES should be stored on moisture-free surfaces [57].
Several methods were developed for the preparation of DES, as shown in Table 4, to ensure their reproducibility and constant property. The evaporation and grinding are the most commonly employed extraction methods [7, 42]. However, recently advanced extraction methods, including microwave-assisted approach [58], ultrasound-assisted, and freeze-drying synthesis methods, were developed considering extraction time and energy consumption during extraction process [18, 20].
The preparation of DES by heating and stirring methods is carried out by mixing and heating the compounds at a temperature of 50 to 100°C under constant stirring until a homogeneous liquid is formed, as shown in Figure 5. Following the preparation, the colorless eutectic solvent will be formed, which is in this case DES, and finally it will be dried to remove extra moisture [42]. This method is the simplest and most common one. However, there is a limitation, irrespective of the production process: a high temperature may result in the deterioration of the DES due to the esterification reaction of solvents based on choline chloride and carboxylic acids [59]. In addition, uncontrolled stirring speed and heating temperature make the eutectic mixture crystallize. Thus, optimizing the appropriate value for reaction time and heating temperature is required to use the heating and stirring methods.
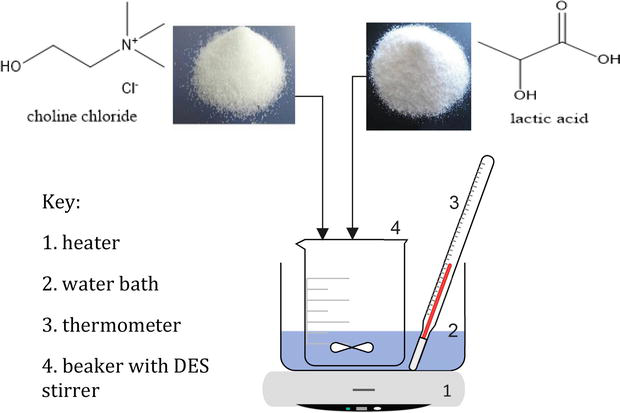
Figure 5.
Schematic diagram for the synthesis of DES by the heating method.
The evaporation method is another way of deep eutectic solvents’ preparation conducted by dissolving DES components in water, followed by evaporation at 50°C and then dropping the solution in a desiccator [60]. The addition of water is performed to enhance the mixing of highly viscose sugar-based DES. Compared to the heating and stirring methods, the evaporation method consumes less power and is mostly suitable for the preparation of NADES, which commonly consist of amino acids, organic acids, and sugars [60].
The grinding method is also the commonly used deep eutectic solvent preparation method, executed by mixing the eutectic solvents at room temperature, then grinding them together using mortar and pestle, until a clear liquid is seen [42]. Due to its hygroscopic nature, the deep eutectic solvent absorbs water, so it has to be stored in a vacuum for hours. In comparison to the heating and evaporation methods, grinding may be done at a lower temperature. However, in order to use the evaporation process, HBA and HBD must be water-soluble. Furthermore, total water removal might be time-consuming [61].
Freeze-drying preparation of deep eutectic solvent is carried out by adding a stoichiometric proportion of HBA and HBD, and then diluting with distilled water to obtain a 5% aqueous solution. To create a clear, viscous liquid, the aqueous solution is frozen at a very low temperature (253 K) and then freeze-dried at a lower temperature [62]. Gutiérrez et al. examined the freeze-drying approach for DES production. The researcher noticed that high-purity DESs may be synthesized and that they are especially ideal for the creation of DESs containing unstable thermal chemicals [63]. However, in terms of energy cost, time cost, and economic sustainability, it is not suited for large-scale manufacture. Thus, searching for advanced methodologies that fill the limitations is crucial for the reliability of DES applications.
Microwave-assisted DES preparation is one of the fastest, cheapest, easiest, and eco-friendly methods [58]. The principle behind microwave-assisted DES preparation is executed by mixing eutectic solvents and placing in irradiation for 20 s after enclosing the solvents in a 20-ml cylinder. Kohli et al. studied microwave-assisted DES preparation, which was used to improve the fractionation of lignocellulosic biomass to yield lignin for biochemical synthesis [21].
Ultrasound-assisted preparation is another recent development for deep eutectic solvent preparation. The process is similar to microwave-assisted preparation of DES, which is performed by mixing HBA and HBD in a glass cylinder stoichiometrically [64]. Then put the mixture in an ultrasonic bath for 1 to 5 h. The ultrasonic heating temperature can be increased from room temperature to 60°C, depending upon the DESs constituents. Finally, stable and non-crystallizable DES can be obtained, after keeping the solution for 24 h in the same container under ambient conditions to ensure the formation of a homogeneous mixture [64].
Espino et al. reviewed different preparation techniques of DES. All the above synthesis techniques and others have their own pros and cons; the thing is, it’s critical to know the approaches of DES preparation after utilizing for different applications. Since, DESs are prone to degradation and the formation of byproducts is based on the synthesis conditions. For instance, DES degrades when synthesized at high temperatures (Table 6) [62].
Methods | Compositions | Findings | Ref. |
---|---|---|---|
Heating and stirring at 80°C | ChCl: LA (1:2) 20% dilution | Phenolic extraction from | [62] |
Heating and stirring at 100°C | ChCl: LA (1:2) 20 v/v% dilution | Saponin extraction from | [62] |
Evaporation method at 50°C | Preparation of DES evaporation methods operated at low-energy heating method and it is mostly suitable for NADES preparation | [65] | |
Ultrasonication (1 to 5 h at 60°C) | 75 v/v% dilution Different alcohol-based DES | Extraction of gingerols from ginger. Extraction T > 50 °C induced degradation and loss of phenolic compounds. DESs are stable and not prone to crystallization | [64] |
Heating and stirring at 60°C, 200 rpm Microwave for enhancement. | ChCl as HBA with six different carboxylic acids as HBD | Delignification of lignocellulosic biomass | [21] |
Heating and stirring methods at 100°C Grinding method at room temperature | ChCl as HBA with carboxylic acid | To assess the properties of DES (densities, viscosities, and refractive index). The case of viscosity grinding methods preparation is preferred than heating method. | [42] |
Microwave-assisted method. For 200 W to 800 W and 5 to 30 s. | Three different NADES prepared | To develop. Microwave-assisted method is an effective NADES preparation method. Since it helps to prepare NADES in short period of time and reduces conventional preparation time from tens of minutes (30–90min) to 20 sec, and the energy consumption, 650 times less. 200 W for 20 sec is the best value to reach eutectic point. | [58] |
Table 6.
A summary table of deep eutectic solvent synthesis methods.
3.2 Characterization of DES physicochemical properties
The physical and chemical properties of deep eutectic solvent have to be determined, prior to using deep eutectic solvents for different applications, including the isolation of lignin from lignocellulosic biomass [66]. Thus, physical and chemical properties that affect the DES properties and are to be characterized include phase behavior, density, viscosity, ionic conductivity, surface tension, and polarity.
Characterization tools, such as ultraviolet-visible (UV-vis) spectroscopy, Raman, infrared (IR), and fluorescence correlation spectroscopy *(FCS) and others, can be used to characterize and understand the molecular dynamics of DESs [67]. In addition, thermogravimetric analysis (TGA) for determining thermal properties, Fourier transform infrared spectroscopy (FTIR) for determining the functional groups of DES, pycnometer for performing density analysis, and viscometer for conducting viscosity analysis [44, 59], as shown in Figure 6.

Figure 6.
Characterizing tools for determining the properties of deep eutectic solvents.
For instance, Rodriguez Rodriguez et al. characterized biodegradability of choline chloride and carboxylic acid-based deep eutectic solvents prepared by the heating method. The researcher studied the degradation of DES using TGA and nuclear magnetic resonance (NMR) techniques. The reported result showed that the DES of this family is prone to degradation between 125 and 235°C due to esterification reaction and from the 1H NMR (proton nuclear magnetic resonance) analysis, it was also confirmed that the DESs had esterified to a certain extent [59].
Dai et al. characterized the physiochemical properties such as viscosity, density, conductivity, and polarity of NADES prepared from different chemicals using the heating method with stirring at 70°C, until a clear liquid was formed. The researcher used FTIR (Bruker FT-IR spectrometer at 25°C), NMR (40°C on a 500 MHz Bruker DMX-500 spectrometer), viscometer, coulometer (Metrohm), and density meter (DMA 5000) for characterizing prepared NADES. Furthermore, a polarity test was performed with Nile Red (NR) as a solvatochromatic probe to estimate the polarity of NADES in the form of molar transition energy [44].
Cui et al. prepared deep eutectic solvents by mixing choline chloride with different organic acids as hydrogen bond donors using different methods such as stirring, grinding, and stirring with grinding methods. Physical parameters (density, electrical conductivity, dynamic viscosity, and refractive index) were examined at temperatures ranging from 15.15 to 65.15°C at atmospheric pressure [33].
Alvarez-Vasco et al. characterized the lignin isolated by DES for their molecular weight and their cleavage of ether bond using GPC (gel permeation chromatography) and C NMR (Carbon-13 nuclear magnetic resonance). The NMR analysis showed the absence of the ether linkage β-O-4 after pretreatment of the milled wood. Whereas the GPC analysis showed that the molecular weight of about 890 g/mol provides evidence that DES depolymerizes native lignin by selective cleavage of ether bonds without subsequent condensation reactions [23].
4. DES mediated lignin isolation and recent advancements
Pretreatment processes are primarily concerned with the isolation of interconnected fractions of lignocellulosic contents and increasing the accessibility of each individual content, making them an essential step in a wide range of valorizations [68]. Thus, to make greater use of lignocellulosic biomass for lignin-based coproducts, a method for fractionating lignin from the feedstock with high effectiveness and low cost must be developed. Currently, physical, chemical, physiochemical, and biological methods are the major approaches developed for lignocellulosic biomass pretreatment strategies [69].
Physical pretreatment methods are commonly used to reduce biomass particle size and disintegrate lignocellulosic components. Physical pretreatments, such as milling, extrusion [70], microwave [68], and ultrasound pretreatment [71], are commonly used, and to facilitate the process, chemicals and enzymes are also used. Compared with physical pretreatments, a microwave pretreatment has several advantages, such as: short reaction times, rapid and uniform heating, low reaction activation energy, high product yield, minimal side products, cost-effectiveness, eco-friendliness, and energy efficiency [68].
Chemical pretreatment of lignocellulosic biomass, on the other hand, is performed by using either acid, alkali, ionic liquids, or combinations of one another to alter the physical and chemical structure of lignocellulosic biomass. Many chemical pretreatment techniques were studied for valorization of lignocellulosic biomass, some of which are alkali [70], acidic [72], organosolv [73], cosolvent enhanced lignocellulosic fractionation (CSELF) [74], ammonia fiber explosion (AFEX), and deep eutectic solvent (DES) pretreatment [75]. However, DES is a recently developed green solvent that is used for delignification. Properties, such as low volatility, high solubility, biodegradability, cheap cost, environmental friendliness, and structural feasibility, promoted their widespread use in the effective delignification of various biomass feedstocks [9].
For instance, Malaeke et al. solubilized lignin from wheat straw up to 50% w/w in a DES consisting of resorcinol-ChCl coupled with ultrasonic irradiation, which improves the mass transfer and dissolution process. DES is prepared by the heating method with subsequent heating at 100°C and stirring until a clear liquid is formed. During pretreatment, the solubility of cellulose in the prepared DES is very low; thus, it enhances the isolation of lignin [76].
The primary step toward lignin valorization via various techniques is effective lignin isolation from lignocellulosic biomass. Many research works on DES pretreatment have indicated easy lignin isolation/delignification using various DESs, as shown in Table 7. The HBA of most DESs used for pretreatment is choline chloride (ChCl), whereas the HBDs are more diverse, with carboxylic acids, polyols, and amides often being utilized. Several carboxylic acids generated from biomass, like formic acid (FA), lactic acid (LA), and oxalic acid (OA), have been used as HBDs in DES synthesis.
DES | Ratio | Biomass | Parameters | Lignin yield | Ref. |
---|---|---|---|---|---|
ChCl: OA | 1:1 | Corncob | 90°C, 24 h | 98.5 | [77] |
ChCl: LA | 1:2 | Corncob | 90°C, 24 h | 64.7 | [77] |
ChCl: LA | 1:15 | Corncob | 90°C, 24 h | 93.1 | [77] |
ChCl: OA | 1:1 | Poplar | Microwave, 3 min | 80 | [66] |
ChCl: LA | 1:2 | Switchgrass | Microwave,45 s | 72 | [78] |
ChCl: LA | 1:2 | Corn stover | Microwave,45 s | 79.6 | [78] |
ChCl: LA | 1:2 | Miscanthus | Microwave, 45 s | 65.2 | [78] |
ChCl: LA | 1:2 | Poplar | 145°C, 6 h | 78.5 | [23] |
ChCl: LA | 1:5 | Rice straw | 60°C, 12 h | 60 | [67] |
ChCl: LA | 1:10 | Eucalyptus | 110°C, 6 h 80 | 80 | [79] |
ChCl: LA | 1:10 | Willow | 120°C, 12 h | 91.8 | [80] |
ChCl: LA | 1:9 | Poplar | 120°C, 6 h | 98.1 | [81] |
ChCl: LA | 1:5 | Bagasse | 80°C, 12 h | 50.6 | [31] |
ChCl: FA | 1:2 | Corn stover | 130°C, 2 h | 23.8 | [82] |
ChCl: EG | 1:2 | Corncob | 90°C, 24 h | 87.6 | [77] |
ChCl: GLY | 1:2 | Corncob | 150°C, 15 h | 59.0 | [83] |
ChCl: GLY | 1:2 | Corn stover | 180°C, 2 h | −60 | [82] |
ChCl: GLY | 1:2 | Switchgrass | 110°C,1 h | 76.6 | [84] |
ChCl: GLY: AlCl3·6H2O | 1:2:0.28 | Poplar | 120°C,4 h | 95.5 | [84] |
ChCl: EG | 1:2 | Switchgrass | 130°C, 30 min | 78.9 | [85] |
GH:EG: PTSA | 1:1.94:0.06 | Switch grass | 120°C, 6 min | 82.1 | [81] |
ChCl: ME | 1:6 | Wheat straw | 90°C, 12 h | 81.0 | [85] |
ChCl: DE | 1:8 | Wheat straw | 90°C, 12 h | 73.5 | [85] |
ChCl: imidazole | 3:7 | Corncob | 115°C, 15 h | 76.7 | [83] |
ChCl: Urea | 1:2 | Corncob | 80°C, 15 h | 27.1 | [83] |
ChCl: Urea | 1:2 | Rice straw | 130°C, 8 h | 44.7 | [86] |
ChCl: PCA | 1:1 | Switchgrass | 160°C, 3 h | 60.8 | [87] |
TEBAC:LA | 1:9 | Wheat straw | 100°C, 10 h | 79.7 | [88] |
ChCl: LA | 1:5 | Oil palm empty fruit bunch | 120°C for 8 h | 88.0 | [89] |
ChCl: OA | 1:2 | Rice straw | 120°C for 1 h | 75.9 | [90] |
BTMAC:LA | 1:2 | Corncob | 140°C, 2 h | 63.4 | [91] |
Table 7.
Analysis of lignin isolation using DES from different lignocellulosic biomasses.
Deep eutectic solvents (DESs) from choline chloride as HBA and carboxylic acid, polyols, and others as HBDs were used for the isolation of lignin from lignocellulosic biomass. From others, DES from choline chloride as HBA with carboxylic acid as HBD solvent are the most effective for lignin isolation. However, the process takes long time at an operating temperature of 60 to 150°C, which needs modification. Recently, high-performance microwave-assisted DES treatment is introduced, intended to reduce time and energy [66, 77].
Deep eutectic solvent (DES) from choline chloride as HBA and polyols that include glycerol, ethylene glycol, and propylene glycol as HBD is another type of DES that was used previously for lignocellulose pretreatment. From this category, ChCl: GLY (choline chloride: glycerol) is an effective polyols-based DES-lignin isolation, which yields 60–90% [66, 83]. Likewise, it has limitations in reaction time and operating temperature as the previous one. In order to overcome these limitations, acidified polyol was introduced [84]. In addition, to further shorten time and enhance yield, ternary DES was developed [81, 92]. The schematic representation of the general mechanisms of lignin isolation using DES was illustrated in Figure 7.

Figure 7.
General schematic diagram of lignin isolation using DES.
5. Factors affecting DES-lignin pretreatment
The key process parameters that distinguish DES pretreatment of lignocellulosic biomass from others are reaction temperature, reaction time, molar ratio, and type of DES component [93]. Hong et al. categorized the parameters that affect DES pretreatment efficiency as characteristics of biomass (components of raw biomass, crystallinity of biomass, and particle size), characteristics of DES (HBA, HBD, and molar ratio), and reaction conditions (reaction time, temperature, and solid-to-liquid ratio) [10].
For instance, Okur et al. studied the effects of DES component molar ratio, pretreatment temperature, and pretreatment time on lignin removal effectiveness. The raw material paddy husks and the DES solvent (ethylene glycol) EG: CA (citric acid) were utilized in the investigation. The study reported varied lignin yields in percentage (47.10, 52.35, and 51.17%) utilizing DES of EG:CA molar ratios of 1:1,1:2, and 2:1 at 90°C. It has been found that pretreatment temperature and reaction time have a substantial impact on the production of lignin from paddy husks. Because intermolecular hydrogen bonds are decreased at high temperatures, the viscosity of the DES solution decreases, DES rapidly enters the lignocellulosic structure, and therefore the lignin removal efficiency rises. In this work, the most significant parameters that affect deep eutectic solvent pretreatment of lignocellulosic biomass, such as temperature, molar ratio, reaction time, types of biomasses, and types of DES solvents, are included.
5.1 Reaction temperature
The pretreatment temperature is a critical key parameter influencing the degree of delignification in the DES pretreatment response [93]. For instance, increasing temperature decreases viscosity of DES [21]. In addition, DES may enhance the breaking of huge quantities of ether bonding, particularly β-O-4 bond, and the resulting lignin becomes lower molecular weight lignin. Thus, the removal of lignin is highly impacted by reaction temperature [6]. In general, the commonly used DES pretreatment temperature ranges from 60 to 200°C [94, 95].
Y. Liu et al. studied the influence of temperature on lignin isolation by DES (the molar ratio of ChCl to p-coumaric acid was 2:1) for 7 h with a solid-to-liquid ratio of 1:10. When the pretreatment temperature was increased from 140 to 160°C and 180–200°C, consequently, delignification rate was increased from 8, 10, and 50 to 84%, respectively, and similarly, for the second temperature range, delignification rate was changed from 27, 36, and 66–90%, respectively. Ma et al. also performed experiments in order to improve wheat straw fractionation efficiency using a deep eutectic solvent (DES) solution comprised of chlorine and lactic acid. In the study, wheat straw pretreatment was conducted by utilizing chloride: lactic acid (1:2) and DES solution (1:20 solid-to-liquid ratio) at various temperatures (90, 120, 150, and 180°C) for 6 and 12 h. It is reported that the yields of lignin were increased from 15.26 to 81.54% and purity of 91.33% with temperature increase from 90 to 180°C. Thus, the optimal temperature obtained in the pretreatment of wheat straw for lignin isolation was 150°C for 6 h [96].
Lou et al. conducted a comprehensive analysis of lignin nanoparticle synthesis from wheat straw using ChCl: LA-based DES. In the study, process parameters, such as temperature (90 to 150°C) and reaction time (6 to 24 h), were considered. The reported result showed high-purity lignin (up to 94.8%) with a high yield (up to 81.5% from air-dried samples and 85.9% from oven-dried samples) at 150°C for 24 hours [97]. This indicates that ChCl: LA DES can isolate lignin efficiently at optimum reaction time and temperature.
C. W. Zhang et al. investigated the effects of different DES combinations, pretreatment temperature, and reaction time on the utilization of DESs in the pretreatment of lignocellulosic biomass. Analyzing the effects of pretreatment temperature on lactic acid with ChCl-treated corncob (2:1) resulted in that lignin extractability improved (18.1–95.5%) when the pretreatment temperature goes from 70 to 110°C. In the study, it was concluded that the reaction time of 24 h and temperature of 90°C were the optimum values obtained. It can be concluded that pretreatment temperature is one of the most important aspects of DES pretreatment of lignocellulosic biomass, and optimizing reaction temperature to improve lignin removal from lignocellulose biomass will be one of the research hotspots [77].
5.2 Reaction time
The pretreatment time of DES is found to be in the range of 0.5 to 24 h, based on different researches [23, 77]. As a result, optimum pretreatment time should be adequate not only to achieve a high lignin yield. Since, the solid recovery rate can be lowered by longer pretreatment time because carbohydrate components in lignocellulosic biomass could be dissolved and decomposed clearly. Thus, it is required to optimize reaction time in the pretreatment of lignocellulose to get the required yield for valorization of lignocellulosic biomass.
For instance, Liu et al. processed wheat straw at 100°C with a DES (triethyl benzyl ammonium chloride: lactic acid) molar ratio of 1:9 and lecithin: sphingomyelin (LS) ratio of 1:15. To determine the effect of time on DES pretreatment, the effects of different pretreatment times of 2, 4, 6, 8, 10, and 12 h were examined. The recovery rate of the cellulose-enriched residues rapidly dropped with increasing pretreatment duration, falling from 66% in 2 h to 48.7% in 12 h. Meanwhile, the xylan and lignin removal rates improved steadily from 43% in 2 h to 78% in 12 h and 48% in 2 h to 74% in 12 h, respectively [88]. For different molar ratios, reaction times, DES compositions, and raw materials, the increment lignin yield with reaction time was confirmed by the other researcher. Li et al. used ChCl: LA at different molar ratios of (1:2, 1:4, 1:6, 1:8, 1:10, and 1:12), temperature at (90–120°C), and reaction time (4–62 h) for delignification of willow. The result indicates that the efficiency of delignification increased significantly with increasing treatment time from 6 h to 12 h at molar ratio of (1:10). Delignification at ChCl: LA (1:10), temperature (20°C), and 12 h resulted in 91.82% lignin yield but when the treatment time extended to 42 h, the lignin yield increased to 94.2% [66]. However, as the treatment time increased, the solid residue yield decreased, indicating that the carbohydrate components dissolved and/or degraded significantly. Thus, reaction time of 12 h is the optimum time for delignification.
Zhang et al. investigated the effects of temperature (70 to 110°C) and time (1.5 to 36 h) at fixed DES molar ratio of ChCl: LA (2:1) on delignification of corn cup. The effects of pretreatment time on the corncob by lactic acid: ChCl (2:1) were studied with the elongation of pretreatment time from 1.5 to 36 h at a temperature of 70 to 110°C. The reported results showed that the lignin extractability efficiency increased significantly with time. The optimal pretreatment temperature and time were shown to be 24 h and 90°C. In general, most of the research works reported that increasing reaction time favors to increase the yield of lignin extraction. Increase the removal rate of lignin, which increased the accessible area of polysaccharides. Another significant reason was that as time goes by, better swelling of cellulose fibers could be obtained by pretreatment solvent’s penetration into biomass fiber bundles [77].
5.3 Molar ratio of HBA to HBD
The molar ratio of DESs, i.e., the ratio between hydrogen bond acceptor (HBA) and hydrogen bond donor (HBD), has effect on DES formulation. Hence, different formulations of HBA to HBD were discovered. From the different types of DES combinations, ChCl: LA formulation is the most commonly researched type. Since, this combination is effective to isolate lignin [98, 99]. For willow lignocellulosic biomass, Li et al. utilized ChCl: LA at different molar ratios (1:2, 1:4, 1:6, 1:8, 1:10, and 1:12) to investigate their effect on the isolation of lignin from willow at temperatures of 90–120°C and time at 4–6 h. It was shown that choline chloride to lactic acid with a molar ratio of 1:10 was an optimum molar ratio yielding a maximum lignin amount, 91.82% [100].
For corncob, C. W. Zhang et al. conducted experiments using an LA: ChCl DES solvent and investigated the impacts of DES molar ratio (2:1 to 15:1), pretreatment temperature (70 to 110°C), and reaction time from 1.5 to 36 hours on the delignification of corn cup. Thus, the result showed that the lignin extractability increased (64.7–93.1%), as the molar ratio of lactic acid to ChCl increased from 2:1 to 15:1 [77]. For different biomasses and DESs, Okur et al. studied the effects of molar ratio, temperature, and time. In the study, rice husks were pretreated using an EG: CA binary DES system. Pretreatment tests were carried out at 90°C for 4–24 h and at 120°C for 1–16 h with EG: CA molar ratios of 1:1, 1:2, and 2:1. The percentage of delignification efficiency for DES systems with EG: CA molar ratios of 1:1, 1:2, and 2:1 was determined to be 47.10, 52.35, and 51.17%, respectively. After 4 hours of pretreatment at 120°C, the maximum lignin removal was determined to be 57.33%, which indicates that delignification efficiency with varying molar ratios was found to be relatively similar [101]. Thus, process parameters, such as temperature and time, were considered to be more critical parameters influencing the delignification of rice husk. To confirm, a detailed analysis using different DES formulations is required.
Tan et al. synthesized five distinct DESs (ChCl: LA, ChCl: MA, ChCl: CA, ChCl: FA, and ChCl: AA) to investigate the effects of molar ratio on the delignification of lignocellulosic biomass (empty oil palm fruit bunch). The results indicated that increasing HBD concentration enhanced lignin extraction by 61% and 40% in the ChCl: LA and ChCl: AA pretreatments, respectively, whereas lignin extraction was only 6% improved in the ChCl: MA pretreatment. When the ratio of ChCl to LA was adjusted from 1:1 to 1:15, lignin production gradually increased from 33 to 61%. On the other hand, increasing the molar ratio from 1:2 to 1:5 resulted in a significant decrease in lignin output from 62 to 25%. This implies that a 1:5 ratio may not be an appropriate eutectic composition for cycloserine-cefoxitin fructose agar (CC-FA) DES and for delignification. It can be observed that high amount of lignin yield (61 and 62%) was obtained using two different DESs at optimum molar ratios; ChCl: LA at 1:15 and ChCl: FA at 1:2, respectively [98, 99]. Hence, optimizing molar ratio of DES for better isolation of lignin is worth.
5.4 Type of DES component
Acidic DES is commonly used in the pretreatment of lignocellulosic biomass because it effectively delignifies lignin and hemicellulose and significantly improves the enzymatic hydrolysis of lignocellulosic pretreatment residues [77]. The DES generated from ChCl: Urea generally fractionates lignin by cleaving the ether bond. Under alkaline circumstances, the breakdown of the β-O-4 bond in non-phenolic-type lignin model molecules can progressively generate epoxide intermediates. In the presence of alkaline DES, the phenolic hydroxyl group may produce quinone methylator-type intermediates due to deprotonation, which then undergoes depolymerization and repolymerization.
C. Li et al. investigated the effects of acidic DES on lignocellulose pretreatment. In the study, sugarcane bagasse and five DESs: ChCl: Urea, ChCl: EG, ChCl: Gly, ChCl: LA, and ChCl: OA were used. It reported that acidic DESs were more soluble than basic DESs. Typically, ChCl: OA isolated lignin successfully, but ChCl: LA isolated lignin less effectively than ChCl: OA; ChCl: Gly, ChCl: EG, and ChCl: Urea. Whereas ChCl: LA and ChCl: OA successfully enhanced the crystallinity of bagasse fibers, 62.3 and 61.7%, respectively. For different lignocellulose biomasses, rice husk and sugar cane bagasse [16], N. Kumar et al. studied the potential of five different deep eutectic solvents for delignification of rice husk and bagasse. The research work investigated that DES composed of ChCl: FA, ChCl: LA, and ChCl: LA was effective. The other two DESs (betaine: lactate and proline: lactate) had showed poor delignification due to weak acidity of DES, but DES with higher acidity (lower pKa) delignified lignin more effectively [102].
Lin et al. processed bamboo waste with various DES molar ratios of ChCl: LA. The findings revealed that increasing the ratio of choline chloride to lactic acid from 2:1 to 1:8 improved the lignin isolation capacity from 42.3 to 80.3% at temperatures of 110 and 130°C, respectively [103, 104]. Similarly, H. Xu, et al. analyzed the effects of DES components’ molar ratio using DES of ChCl: LA. The study revealed that delignification decreased with increasing lactic acid content and on the other side, an increase in the monocarboxylic acid hydrogen bonding donor, resulting in enhanced delignification and a decrease in molecular chain length [93]. Whereas, Tan et al. studied the effects of types of DES on delignification of oil palm fruit bunch employing five different acidic deep eutectic solvents; ChCl: LA, ChCl: MA, ChCl: CA, ChCl: FA, and ChCl: AA. The results demonstrated that the presence of hydroxyl groups and short alkyl chains improved lignin extraction. A 1:15 ratio of choline chloride to lactic acid (ChCl: LA) and a 1:2 ratio of choline chloride to formic acid (ChCl: FA) isolated more than 60% of lignin [98, 99].
T. Li et al. also performed experiments to investigate the type of DES and reaction temperature effects on the isolation of lignin from willow. The types of DESs were glycerol, urea, and lactic acid mixed with choline chloride at fixed molar ratio (1:2). Whereas the reaction temperature (90, 100, 110, and 120°C for 6 h) was another parameter that was considered to investigate the treatment effects on lignin yield. The final result reported that the amount of lignin isolated varied with the type of DES, as reportedly high amount of lignin was obtained from DES of ChCl: LA [75]. In addition, it was also investigated that lignin yield varied with reaction temperature and time, which increased with increasing temperature and
5.5 Type of biomass
Deep eutectic solvent (DES) pretreatment has varying effects based on the kind of lignocellulosic biomass feedstock. Alvarez-Vasco et al. investigated the effects of biomass type, such as hardwood and softwood, using DES. The result revealed that the yield of lignin varies with the type of biomass, 78% for hardwood and 58% for softwood [23].
Morán et al. pretreated sugarcane bagasse and beer bagasse using DES of ChCl: GLY. The reported result showed 80 and 15% acid-soluble lignin and Klason lignin isolation, respectively. In addition, it showed increment of saccharification yields with 60, 80, and 100% of dextran, xylan, and arabinoxylan, respectively [96].
For poplar sawdust and herbaceous manzanita, Zhou et al. conducted an experiment employing DES consisting of p-toluene sulfonic acid and choline chloride and exhibited practically full cellulose conversion, with over 90% delignification and xylan elimination [105]. However, thus studies have not determined the reason why the yields of lignin vary with biomass type.
H. Xu et al. conducted an experiment to study the synergistic impacts of various critical factors on DES pretreatment and studied the composition of lignocellulose biomass that hinders DES pretreatment. In the study, 34 different lignocellulosic biomasses were used, including wheat straw, oil palm empty fruit bunches, rice straw, maize straw, and willow jelly. The experiments with biomass feedstocks were investigated using multivariate analysis. The study established that xylan content, lignin content, and dextran content are the most significant components of biomass that influence the effect of DES pretreatment [93]. As seen, the effects of pretreatment vary with the type of biomass, which may be due to the structure of lignin. Thus, it will be a challenge since the structure of lignocellulose biomass varies based on the environment and nature from one area to another, which is also a problem for other pretreatment techniques. For the long-term goal of a renewable economy, genetically engineered biomass types should be planted.
6. Valorization of deep eutectic solvent isolated lignin
Lignin can be upgraded into a number of hydrocarbon fuels and high-value bioproducts (such as aromatic chemicals, macromolecules, advanced materials, or polymers) [106]. Thus, products from lignin can be valorized or processed in different ways depending on the properties of DES lignin. Lignin extracted by deep eutectic solvents has unique properties, such as high purity, low molecular weight, high reactivity, and more preserved β-O-4 bonds, which makes DES lignin interesting for upgrading to aromatic chemical synthesis and synthesis of various polymers. However, these properties may vary with the type of DES. For instance, from the different DES types, carboxylic acid-based DES was the most studied and explored unique feature [4].
Following the properties of extracted lignin, currently there are two categories of lignin valorization such as functionalization and depolymerization [3].
Depolymerization is one of the methods of lignin valorization, which can be carried out by biological [107], thermochemical [108], and electrochemical [109] methods. Lignin’s chemical structure may be depolymerized using high and intermediate energetic, catalytic, and biotransformation processes to yield low-value carbon sources for energy generation as well as high-value molecules, such as vanillin and other phenolic compounds.
Functionalization is another way of lignin valorization, described as the activity of improving the reactivity of specific functional groups, such as hydroxyl (OH), methoxyl, carbonyl, carboxyl, or aromatic groups, by hydroxyalkylation, esterification, alkylation, amination, and epoxidation [110]. Following functionalization, lignin can be processed into carbon nanofibers, nanoparticles, resins, polyurethanes, biocomposites, and others [108]. Zdanowicz et al. synthesized a composite thermoplastic starch plasticized by DES of urea: resorcinol: choline chloride (U: R: ChCl, 2:1:1) from potato starch and lignin using twin screw extrusion [111].
Furthermore, because of the strong reactivity
Another interesting characteristic of lignin is the degree of demethylation, which increases the reactivity of lignin toward chemical alteration. It is shown that lignin extracted from willow via ChCl: LA pretreatment showed more phenolic hydroxyl groups than the other technical lignin, suggesting high reactivity of lignin [80]. Thus, the purity and reactivity of lignin extracted using carboxylic acid-based DES make it appropriate for the production of different polymers [86]. Polyurethane (PU) and phenolic resin are of special importance due to their widespread industrial uses [86, 112]. Kalami et al. produced phenol formaldehyde from agricultural lignin by dilute acid pretreatment and hydrolysis from corn stover [112]. The characteristic properties showed that the produced lignin-phenol resin is similar to commercial phenol resin. Whereas, Xia et al. utilized lignin for the production of biodegradable bioplastic. This work is intended to fill the limitations in mechanical performance and wet stability of bioplastic produced from different biomasses [113]. As a result, the lignocellulosic bioplastic produced has great mechanical strength, excellent water stability, ultraviolet (UV)-light resistance, and increased thermal stability. Furthermore, because it can be easily recycled or biodegraded in the natural environment, lignocellulosic bioplastic has a lesser environmental effect.
7. Conclusions
This book chapter discussed recent advances in lignin fractionation and valorization using deep eutectic solvents (DESs) as a more environmentally friendly alternative to fossil-based synthesis. DESs are promising for lignin extraction due to their chemical and thermal stability, low volatility, and simplicity of synthesis. However, more theoretical and technological studies are needed to better understand the molecular interactions between DESs and lignin, to develop effective DESs recycling methods, and to create more economically feasible lignin biorefinery systems. Tunable DES systems with specific lignin properties for cost-effective valorization are expected in the near future. More research is needed to determine the most effective usage of DES, selection criteria, and operating conditions.
Acknowledgments
The authors acknowledge Helena Recic, Publishing Process Manager in INTECHOPEN for her kindness and endless support. The authors also acknowledge the academic editors and INTECHOPEN for accepting funding wavier.
References
- 1.
Ullah M, Liu P, Xie S, Sun S. Recent advancements and challenges in lignin valorization: Green routes towards sustainable bioproducts. Molecules. 2022; 27 :6055 - 2.
Nadányi R, Ház A, Lisý A, Jablonský M, Šurina I, Majová V, et al. Lignin modifications, applications, and possible market prices. Energies. 2022; 15 (18):1-16 - 3.
Zhou M, Fakayode OA, Ahmed Yagoub AEG, Ji Q, Zhou C. Lignin fractionation from lignocellulosic biomass using deep eutectic solvents and its valorization. Renewable and Sustainable Energy Reviews. Pergamon. 2022; 156 :111986 - 4.
Chen Z, Ragauskas A, Wan C. Lignin extraction and upgrading using deep eutectic solvents. Industrial Crops and Products. [Internet]. 2020; 147 (February):112241. DOI: 10.1016/j.indcrop.2020.112241 - 5.
Poveda-Giraldo JA, Solarte-Toro JC, Cardona Alzate CA. The potential use of lignin as a platform product in biorefineries: A review. [Internet]. Renewable and Sustainable Energy Reviews. 2021; 138 :110688. DOI: 10.1016/j.rser.2020.110688 - 6.
Hong S, Shen XJ, Pang B, Xue Z, Cao XF, Wen JL, et al. In-depth interpretation of the structural changes of lignin and formation of diketones during acidic deep eutectic solvent pretreatment. Green Chemistry. 2020; 22 (6):1851-1858 - 7.
El Achkar T, Greige-Gerges H, Fourmentin S. Basics and properties of deep eutectic solvents: A review. [Internet]. Environmental Chemistry Letters. 2021; 19 :3397-3408. DOI: 10.1007/s10311-021-01225-8 - 8.
Smith EL, Abbott AP, Ryder KS. Deep eutectic solvents (DESs) and their applications. Chemical Reviews. 2014; 114 (21):11060-11082 - 9.
Zhang M, Zhang X, Liu Y, Wu K, Zhu Y, Lu H, et al. Insights into the relationships between physicochemical properties, solvent performance, and applications of deep eutectic solvents. Environmental Science and Pollution Research. 2021; 28 (27):35537-35563 - 10.
Hong S, Shen XJ, Xue Z, Sun Z, Yuan TQ. Structure-function relationships of deep eutectic solvents for lignin extraction and chemical transformation. Green Chemistry. 2020; 22 (21):7219-7232 - 11.
Van ODJGP, Van SJ, Esteves ACC, Tuinier R, Vis M. Promising technological and industrial applications of deep EutecticEutectic systems. Physical Chemistry Chemical Physics [Internet]. 2021; 22 :2181-2187. DOI: 10.1039/C9CP06762K - 12.
Abbott AP, Barron JC, Ryder KS, Wilson D. Eutectic-based ionic liquids with metal-containing anions and cations. Chemistry : A European Journal. 2007; 13 (22):6495-6501 - 13.
Meraj A, Singh SP, Jawaid M, Nasef MM, Alomar TS, AlMasoud N. A review on eco-friendly isolation of lignin by natural deep eutectic solvents from agricultural wastes. [Internet]. Journal of Polymers and the Environment. 2023; 31 (8). DOI: 10.1007/s10924-023-02817-x - 14.
Florindo C, Branco LC, Marrucho IM. Quest for green-solvent design: From hydrophilic to hydrophobic (deep) eutectic solvents. ChemSusChem. 2019; 12 (8):1549-1559 - 15.
Abbott AP, Capper G, Davies DL, Rasheed RK. Ionic liquid analogues formed from hydrated metal salts. Chemistry : A European Journal. 2004; 10 (15):3769-3774 - 16.
Cao J, Su E. Hydrophobic deep eutectic solvents: The new generation of green solvents for diversified and colorful applications in green chemistry. Journal of Cleaner Production [Internet]. 2021; 314 (December 2020):127965. DOI: 10.1016/j.jclepro.2021.127965 - 17.
Gurkan B, Squire H, Pentzer E. Metal-free deep eutectic solvents: Preparation, physical properties, and significance. Journal of Physical Chemistry Letters. 2019; 10 (24):7956-7964 - 18.
Santana APR, Mora-Vargas JA, Guimarães TGS, Amaral CDB, Oliveira A, Gonzalez MH. Sustainable synthesis of natural deep eutectic solvents (NADES) by different methods. Journal of Molecular Liquids. 2019; 293 :17-20 - 19.
Abranches DO, Martins MAR, Silva LP, Schaeffer N, Pinho SP, Coutinho JAP. Phenolic hydrogen bond donors in the formation of non-ionic deep eutectic solvents: The quest for type v des. Chemical Communications. 2019; 55 (69):10253-10256 - 20.
Li P, Zhang Z, Zhang X, Li K, Jin Y, Wu W. DES: Their effect on lignin and recycling performance. RSC Advances. 2023; 13 (5):3241-3254 - 21.
Kohli K, Katuwal S, Biswas A, Sharma BK. Effective delignification of lignocellulosic biomass by microwave assisted deep eutectic solvents. Bioresource Technology. 2020; 303 :122897 - 22.
Liu Q, Zhao X, Yu D, Yu H, Zhang Y, Xue Z, et al. Novel deep eutectic solvents with different functional groups towards highly efficient dissolution of lignin. Green Chemistry. 2019; 21 (19):5291-5297 - 23.
Alvarez-Vasco C, Ma R, Quintero M, Guo M, Geleynse S, Ramasamy KK, et al. Unique low-molecular-weight lignin with high purity extracted from wood by deep eutectic solvents (DES): A source of lignin for valorization. Green Chemistry. 2016; 18 (19):5133-5141 - 24.
Liu Q, Mou H, Chen W, Zhao X, Yu H, Xue Z, et al. Highly efficient dissolution of lignin by eutectic molecular liquids. Industrial and Engineering Chemistry Research. 2019; 58 (51):23438-23444 - 25.
Salehi HS, Ramdin M, Moultos OA, Vlugt TJH. Computing solubility parameters of deep eutectic solvents from molecular dynamics simulations. Fluid Phase Equilibria [Internet]. 2019; 497 :10-18. DOI: 10.1016/j.fluid.2019.05.022 - 26.
Zhang Q, De Oliveira VK, Royer S, Jérôme F. Deep eutectic solvents: Syntheses, properties and applications. Chemical Society Reviews. 2012; 41 (21):7108-7146 - 27.
Hou YC, Yao CF, Wu WZ. Deep eutectic solvents: Green solvents for separation applications. Wuli Huaxue Xuebao/Acta Physico-Chimica Sinica. 2018; 34 (8):873-885 - 28.
Liu P, Hao JW, Mo LP, Zhang ZH. Recent advances in the application of deep eutectic solvents as sustainable media as well as catalysts in organic reactions. RSC Advances [Internet]. 2015; 5 (60):48675-48704. DOI: 10.1039/C5RA05746A - 29.
Abbott AP, Capper G, Davies DL, Rasheed RK, Tambyrajah V. Novel solvent properties of choline chloride/urea mixtures. Chemical Communications. 2003; 1 :70-71 - 30.
Makoś P, Przyjazny A, Boczkaj G. Hydrophobic deep eutectic solvents as “green” extraction media for polycyclic aromatic hydrocarbons in aqueous samples. Journal of Chromatography. A. 2018; 1570 :28-37 - 31.
Satlewal A, Agrawal R, Bhagia S, Sangoro J, Ragauskas AJ. Natural deep eutectic solvents for lignocellulosic biomass pretreatment: Recent developments, challenges and novel opportunities. Biotechnology Advances. 2018; 36 (8):2032-2050. DOI: 10.1016/j.biotechadv.2018.08.009 - 32.
Abbott AP, Boothby D, Capper G, Davies DL, Rasheed RK. Deep eutectic solvents formed between choline chloride and carboxylic acids: Versatile alternatives to ionic liquids. Journal of the American Chemical Society. 2004; 126 (29):9142-9147 - 33.
Cui Y, Li C, Yin J, Li S, Jia Y, Bao M. Design, synthesis and properties of acidic deep eutectic solvents based on choline chloride. Journal of Molecular Liquids. 2017; 236 :338-343 - 34.
Alomar MK, Hayyan M, Alsaadi MA, Akib S, Hayyan A, Hashim MA. Glycerol-based deep eutectic solvents: Physical properties. Journal of Molecular Liquids. 2016; 215 :98-103 - 35.
Hayyan A, Mjalli FS, Alnashef IM, Al-Wahaibi YM, Al-Wahaibi T, Hashim MA. Glucose-based deep eutectic solvents: Physical properties. Journal of Molecular Liquids [Internet]. 2013; 178 :137-141. DOI: 10.1016/j.molliq.2012.11.025 - 36.
García G, Aparicio S, Ullah R, Atilhan M. Deep eutectic solvents: Physicochemical properties and gas separation applications. Energy and Fuels. 2015; 29 (4):2616-2644 - 37.
Shah D, Mjalli FS. Effect of water on the thermo-physical properties of reline: An experimental and molecular simulation based approach. Physical Chemistry Chemical Physics. 2014; 16 (43):23900-23907 - 38.
Bara JE, Camper DE, Gin DL, Noble RD. Room-temperature ionic liquids and composite materials: Platform technologies for CO2 capture. Accounts of Chemical Research. 2009; 43 (1):152-159 - 39.
Yadav A, Trivedi S, Rai R, Pandey S. Densities and dynamic viscosities of (choline chloride+glycerol) deep eutectic solvent and its aqueous mixtures in the temperature range (283.15-363.15)K. Fluid Phase Equilibria [Internet]. 2014; 367 :135-142. DOI: 10.1016/j.fluid.2014.01.028 - 40.
Guo W, Hou Y, Ren S, Tian S, Wu W. Formation of deep eutectic solvents by phenols and choline chloride and their physical properties. Journal of Chemical & Engineering Data. 2013; 58 (4):866-872 - 41.
Mjalli FS, Jabbar NMA. Acoustic investigation of choline chloride based ionic liquids analogs. Fluid Phase Equilibria [Internet]. 2014; 381 :71-76. DOI: 10.1016/j.fluid.2014.08.017 - 42.
Florindo C, Oliveira FS, Rebelo LPN, Fernandes AM, Marrucho IM. Insights into the synthesis and properties of deep eutectic solvents based on cholinium chloride and carboxylic acids. ACS Sustainable Chemistry & Engineering. 2014; 2 (10):2416-2425 - 43.
Verma R, Mohan M, Goud VV, Banerjee T. Operational strategies and comprehensive evaluation of menthol based deep eutectic solvent for the extraction of lower alcohols from aqueous media. ACS Sustainable Chemistry & Engineering. 2018; 6 (12):16920-16932 - 44.
Dai Y, Witkamp GJ, Verpoorte R, Choi YH. Tailoring properties of natural deep eutectic solvents with water to facilitate their applications. Food Chemistry [Internet]. 2015; 187 :14-19. DOI: 10.1016/j.foodchem.2015.03.123 - 45.
Widsten P, Tamminen T, Liitiä T. Natural sunscreens based on nanoparticles of modified Kraft lignin (CatLignin). ACS Omega. 2020; 5 (22):13438-13446 - 46.
Nunes RJ, Saramago B, M. Marrucho I. Surface tension of dl-menthol: Octanoic acid eutectic mixtures. Journal of Chemical & Engineering Data. 2019; 64 (11):4915-4923 - 47.
D’Agostino C, Harris RC, Abbott AP, Gladden LF, Mantle MD. Molecular motion and ion diffusion in choline chloride based deep eutectic solvents studied by 1H pulsed field gradient NMR spectroscopy. Physical Chemistry Chemical Physics. 2011; 13 (48):21383-21391 - 48.
Mjalli FS, Naser J, Jibril B, Alizadeh V, Gano Z. Tetrabutylammonium chloride based ionic liquid analogues and their physical properties. Journal of Chemical & Engineering Data. 2014; 59 (7):2242-2251 - 49.
Jibril B, Mjalli F, Naser J, Gano Z. New tetrapropylammonium bromide-based deep eutectic solvents: Synthesis and characterizations. Journal of Molecular Liquids [Internet]. 2014; 199 :462-469. DOI: 10.1016/j.molliq.2014.08.004 - 50.
Reichardt C. Solvatochromic dyes as solvent polarity indicators. Chemical Reviews. 2002; 94 (8):2319-2358 - 51.
Kamlet MJ, Luis Abboud J, Taft RW. The solvatochromic comparison method. 6. The .pi.* scale of solvent polarities. Journal of the American Chemical Society. 2002; 99 (18):6027-6038 - 52.
Kamlet JM, Taft WR. The solvatochromic comparison method. I. The .Beta.-scale of solvent hydrogen-bond acceptor (HBA) basicities. Journal of the American Chemical Society. 2002; 98 (2):377-383 - 53.
Abbott AP, Harris RC, Ryder KS, D’Agostino C, Gladden LF, Mantle MD. Glycerol eutectics as sustainable solvent systems. Green Chemistry. 2011; 13 (1):82-90 - 54.
Valvi A, Dutta J, Tiwari S. Temperature-dependent empirical parameters for polarity in choline chloride based deep eutectic solvents. The Journal of Physical Chemistry. B. 2017; 121 (50):11356-11366 - 55.
Azizi N, Dezfooli S, Hashemi MM. A sustainable approach to the Ugi reaction in deep eutectic solvent. Comptes Rendus Chimie. 2013; 16 (12):1098-1102 - 56.
Mahto A, Mondal D, Polisetti V, Bhatt J, Nidhi MR, Prasad K, et al. Sustainable water reclamation from different feed streams by forward osmosis process using deep eutectic solvents as reusable draw solution. Industrial and Engineering Chemistry Research. 2017; 56 (49):14623-14632 - 57.
Farooq MQ, Abbasi NM, Anderson JL. Deep eutectic solvents in separations: Methods of preparation, polarity, and applications in extractions and capillary electrochromatography. Journal of Chromatography. A [Internet]. 2020; 1633 :461613. DOI: 10.1016/j.chroma.2020.461613 - 58.
Gomez FJV, Espino M, Fernández MA, Silva MF. A greener approach to prepare natural deep eutectic solvents. ChemistrySelect. 2018; 3 (22):6122-6125 - 59.
Rodriguez Rodriguez N, van den Bruinhorst A, Kollau LJBM, Kroon MC, Binnemans K, et al. Degradation of deep-eutectic solvents based on choline chloride and carboxylic acids. ACS Sustainable Chemistry & Engineering. 2019; 7 (13):11521-11528 - 60.
Sekharan TR, Chandira RM, Tamilvanan S, Rajesh SC, Venkateswarlu BS. Deep eutectic solvents as an alternate to other harmful solvents. Biointerface Research in Applied Chemistry. 2022; 12 (1):847-860 - 61.
Zhang M, Tian R, Han H, Wu K, Wang B, Liu Y, et al. Preparation strategy and stability of deep eutectic solvents: A case study based on choline chloride-carboxylic acid. Journal of Cleaner Production. 2022; 345 :131028 - 62.
Espino M, de los Ángeles Fernández M, FJV G, Silva MF. Natural designer solvents for greening analytical chemistry. TrAC Trends in Analytical Chemistry [Internet]. 2016; 76 :126-136. DOI: 10.1016/j.trac.2015.11.006 - 63.
Gutiérrez MC, Ferrer ML, Mateo CR, Del MF. Freeze-drying of aqueous solutions of deep eutectic solvents: A suitable approach to deep eutectic suspensions of self-assembled structures. Langmuir. 2009; 25 (10):5509-5515 - 64.
Hsieh YH, Li Y, Pan Z, Chen Z, Lu J, Yuan J, et al. Ultrasonication-assisted synthesis of alcohol-based deep eutectic solvents for extraction of active compounds from ginger. Ultrasonics Sonochemistry [Internet]. 2020; 63 :104915. DOI: 10.1016/j.ultsonch.2019.104915 - 65.
Dai Y, van Spronsen J, Witkamp GJ, Verpoorte R, Choi YH. Natural deep eutectic solvents as new potential media for green technology. Analytica Chimica Acta [Internet]. 2013; 766 :61-68. DOI: 10.1016/j.aca.2012.12.019 - 66.
Liu YY, Chen W, Xia Q, Guo B, Wang Q, Liu S, et al. Efficient cleavage of lignin–carbohydrate complexes and ultrafast extraction of lignin oligomers from Wood biomass by microwave-assisted treatment with deep eutectic solvent. ChemSusChem. 2017; 10 (8):1692-1700 - 67.
Kumar AK, Parikh BS, Pravakar M. Natural deep eutectic solvent mediated pretreatment of rice straw: Bioanalytical characterization of lignin extract and enzymatic hydrolysis of pretreated biomass residue. Environmental Science and Pollution Research [Internet]. 2016; 23 (10):9265-9275. DOI: 10.1007/s11356-015-4780-4 - 68.
Mankar AR, Pandey A, Modak A, Pant KK. Pretreatment of lignocellulosic biomass: A review on recent advances. Bioresource Technology [Internet]. 2021; 334 (March):125235. DOI: 10.1016/j.biortech.2021.125235 - 69.
Borrero-l AM, Valencia C, Franco JM. Lignocellulosic materials for the production of biofuels, biochemicals and biomaterials and applications of. Polymers (Basel). 2022; 14 (5):881. DOI: 10.3390/polym14050881 - 70.
Kumar B, Bhardwaj N, Agrawal K, Chaturvedi V, Verma P. Current perspective on pretreatment technologies using lignocellulosic biomass: An emerging biorefinery concept. Fuel Processing Technology. 2020; 199 :106244 - 71.
Shah AA, Seehar TH, Sharma K, Toor SS. Biomass pretreatment technologies. Hydrocarb Biorefinery Sustain Process Biomass Hydrocarb Biofuels. 2022:203-228 - 72.
Baruah J, Nath BK, Sharma R, Kumar S, Deka RC, Baruah DC, et al. Recent trends in the pretreatment of lignocellulosic biomass for value-added products. Frontiers in Energy Research. 2018; 6 (Dec):1-19 - 73.
Xu X, Wang K, Zhou Y, Lai C, Zhang D, Xia C, et al. Comparison of organosolv pretreatment of masson pine with different solvents in promoting delignification and enzymatic hydrolysis efficiency. Fuel. 2023; 338 :127361 - 74.
Zhao ZM, Meng X, Scheidemantle B, Pu Y, Liu ZH, Li BZ, et al. Cosolvent enhanced lignocellulosic fractionation tailoring lignin chemistry and enhancing lignin bioconversion. Bioresource Technology. 2022; 347 :126367 - 75.
Tomé LIN, Baião V, da Silva W, Brett CMA. Deep eutectic solvents for the production and application of new materials. Applied Materials Today. 2018; 10 :30-50 - 76.
Malaeke H, Housaindokht MR, Monhemi H, Izadyar M. Deep eutectic solvent as an efficient molecular liquid for lignin solubilization and wood delignification. Journal of Molecular Liquids. 2018; 263 :193-199 - 77.
Zhang CW, Xia SQ, Ma PS. Facile pretreatment of lignocellulosic biomass using deep eutectic solvents. Bioresource Technology [Internet]. 2016; 219 (July):1-5. DOI: 10.1016/j.biortech.2016.07.026 - 78.
Chen Z, Wan C. Ultrafast fractionation of lignocellulosic biomass by microwave-assisted deep eutectic solvent pretreatment. Bioresource Technology. 2018; 250 :532-537 - 79.
Shen XJ, Wen JL, Mei QQ, Chen X, Sun D, Yuan TQ, et al. Facile fractionation of lignocelluloses by biomass-derived deep eutectic solvent (DES) pretreatment for cellulose enzymatic hydrolysis and lignin valorization. Green Chemistry. 2019; 21 (2):275-283 - 80.
Zhao. Organosolv fractionating pre-treatment of lignocellulosic biomass for efficient enzymatic saccharification: Chemistry, kinetics, and substrate structures. Biofuels, Bioproducts and Biorefining. 2017; 8 (6):24 - 81.
Chen Z, Jacoby WA, Wan C. Ternary deep eutectic solvents for effective biomass deconstruction at high solids and low enzyme loadings. Bioresource Technology. 2019; 279 :281-286 - 82.
Xu F, Sun J, Wehrs M, Ho Kim K, Rau SS, Chan AM, et al. Biocompatible choline-based deep eutectic solvents enable one-pot production of cellulosic ethanol. ACS Sustainable Chemistry & Engineering. 2018; 6 (7):8914-8919 - 83.
Procentese A, Johnson E, Orr V, Garruto Campanile A, Wood JA, Marzocchella A, et al. Deep eutectic solvent pretreatment and subsequent saccharification of corncob. Bioresource Technology. 2015; 192 :31-36 - 84.
Chen Z, Bai X, Lusi A, Wan C. High-solid lignocellulose processing enabled by natural deep eutectic solvent for lignin extraction and industrially relevant production of renewable chemicals. ACS Sustainable Chemistry & Engineering. 2018; 6 (9):12205-12216 - 85.
Zhao Z, Chen X, Ali MF, Abdeltawab AA, Yakout SM, Yu G. Pretreatment of wheat straw using basic ethanolamine-based deep eutectic solvents for improving enzymatic hydrolysis. Bioresource Technology. 2018; 263 :325-333 - 86.
Pang B, Yang S, Fang W, Yuan TQ, Argyropoulos DS, Sun RC. Structure-property relationships for technical lignins for the production of lignin-phenol-formaldehyde resins. Industrial Crops and Products. 2017; 108 :316-326 - 87.
Kim KH, Dutta T, Sun J, Simmons B, Singh S. Biomass pretreatment using deep eutectic solvents from lignin derived phenols. Green Chemistry. 2018; 20 (4):809-815 - 88.
Liu Y, Zheng J, Xiao J, He X, Zhang K, Yuan S, et al. Enhanced enzymatic hydrolysis and lignin extraction of wheat straw by Triethylbenzyl ammonium chloride/lactic acid-based deep eutectic solvent Pretreatment. ACS Omega. 2019; 4 (22):19829-19839 - 89.
Tan YT, Ngoh GC, Chua ASM. Evaluation of fractionation and delignification efficiencies of deep eutectic solvents on oil palm empty fruit bunch. Industrial Crops and Products. 2018; 123 :271-277 - 90.
Hou XD, Lin KP, Li AL, Yang LM, Fu MH. Effect of constituents molar ratios of deep eutectic solvents on rice straw fractionation efficiency and the micro-mechanism investigation. Industrial Crops and Products. 2018; 120 :322-329 - 91.
Guo Z, Zhang Q, You T, Zhang X, Xu F, Wu Y. Short-time deep eutectic solvent pretreatment for enhanced enzymatic saccharification and lignin valorization. Green Chemistry. 2019; 21 (11):3099-3108 - 92.
Xing W, Xu G, Dong J, Han R, Ni Y. Novel dihydrogen-bonding deep eutectic solvents: Pretreatment of rice straw for butanol fermentation featuring enzyme recycling and high solvent yield. Chemical Engineering Journal. 2018; 333 :712-720 - 93.
Xu H, Kong Y, Peng J, Song X, Liu Y, Su Z, et al. Comprehensive analysis of important parameters of choline chloride-based deep eutectic solvent pretreatment of lignocellulosic biomass. Bioresource Technology [Internet]. 2021; 319 :124209. DOI: 10.1016/j.biortech.2020.124209 - 94.
Procentese A, Raganati F, Olivieri G, Russo ME, Rehmann L, Marzocchella A. Deep eutectic solvents pretreatment of agro-industrial food waste. Biotechnology for Biofuels and Bioproducts [Internet]. 2018; 11 (1):1-12. DOI: 10.1186/s13068-018-1034-y - 95.
Ma H, Fu P, Zhao J, Lin X, Wu W, Yu Z, et al. Pretreatment of wheat straw lignocelluloses by deep eutectic solvent for lignin extraction. Molecules. 2022; 27 (22):7955 - 96.
Morán-aguilar MG, Costa-trigo I, Ramírez-pérez AM, de Blas E, Calderón-santoyo M, Aguilar-uscanga MG, et al. Development of sustainable biorefinery processes applying deep eutectic solvents to Agrofood wastes. Energies. 2022; 15 (11):1-12 - 97.
Lou R, Ma R, Lin KT, Ahamed A, Zhang X. Facile extraction of wheat straw by deep eutectic solvent (DES) to produce lignin nanoparticles. ACS Sustainable Chemistry & Engineering. 2019; 7 (12):10248-10256 - 98.
Tan YT, Ngoh GC, Chua ASM. Effect of functional groups in acid constituent of deep eutectic solvent for extraction of reactive lignin. Bioresource Technology [Internet]. 2019; 281 :359-366. DOI: 10.1016/j.biortech.2019.02.010 - 99.
Li C, Huang C, Zhao Y, Zheng C, Su H, Zhang L, et al. Effect of choline-based deep eutectic solvent pretreatment on the structure of cellulose and lignin in bagasse. PRO. 2021; 9 (2):1-14 - 100.
Li T, Lyu G, Liu Y, Lou R, Lucia LA, Yang G, et al. Deep eutectic solvents (DESs) for the isolation of willow lignin (Salix matsudana cv. Zhuliu). International Journal of Molecular Sciences. 2017; 18 (11):2266 - 101.
Okur M, Eslek Koyuncu DD. Investigation of pretreatment parameters in the delignification of paddy husks with deep eutectic solvents. Biomass and Bioenergy [Internet]. 2020; 142 (July):105811. DOI: 10.1016/j.biombioe.2020.105811 - 102.
Kumar N, Gautam R, Stallings JD, Coty GG, Lynam JG. Secondary agriculture residues Pretreatment using deep eutectic solvents. Waste and Biomass Valorization [Internet]. 2021; 12 (5):2259-2269. DOI: 10.1007/s12649-020-01176-1 - 103.
Lin W, Xing S, Jin Y, Lu X, Huang C, Yong Q. Insight into understanding the performance of deep eutectic solvent pretreatment on improving enzymatic digestibility of bamboo residues. Bioresource Technology [Internet]. 2020; 306 (March):123163. DOI: 10.1016/j.biortech.2020.123163 - 104.
Xu H, Kong Y, Peng J, Wang W, Li B. Mechanism of deep eutectic solvent delignification: Insights from molecular dynamics simulations. ACS Sustainable Chemistry & Engineering. 2021; 9 (20):7101-7111 - 105.
Zhou X, Huang T, Liu J, Gao H, Bian H, Wang R, et al. Recyclable deep eutectic solvent coupling sodium hydroxide post-treatment for boosting woody/herbaceous biomass conversion at mild condition. Bioresource Technology [Internet]. 2021; 320 (PA):124327. DOI: 10.1016/j.biortech.2020.124327 - 106.
Schutyser W, Renders T, Van Den Bosch S, Koelewijn SF, Beckham GT, Sels BF. Chemicals from lignin: An interplay of lignocellulose fractionation, depolymerisation, and upgrading. Chemical Society Reviews [Internet]. 2018; 47 (3):852-908. DOI: 10.1039/C7CS00566K - 107.
Xu R, Zhang K, Liu P, Han H, Zhao S, Kakade A, et al. Lignin depolymerization and utilization by bacteria. Bioresource Technology. [Internet]. 2018; 269 :557-566. DOI: 10.1016/j.biortech.2018.08.118 - 108.
Zevallos Torres LA, Lorenci Woiciechowski A, de Andrade Tanobe VO, Karp SG, Guimarães Lorenci LC, Faulds C, et al. Lignin as a potential source of high-added value compounds: A review. Journal of Cleaner Production. 2020; 263 :121499 - 109.
Fache M, Boutevin B, Caillol S. Vanillin production from lignin and its use as a renewable chemical. ACS Sustainable Chemistry & Engineering. 2016; 4 (1):35-46 - 110.
Tran MH, Lee EY. Green preparation of bioplastics based on degradation and chemical modification of lignin residue. Journal of Wood Chemistry and Technology [Internet]. 2018; 38 (6):460-478. DOI: 10.1080/02773813.2018.1533978 - 111.
Zdanowicz M, Sałasińska K, Lewandowski K, Skórczewska K. Thermoplastic starch/ternary deep eutectic solvent/lignin materials: Study of physicochemical properties and fire behavior. ACS Sustainable Chemistry & Engineering. 2022; 10 (14):4579-4587 - 112.
Kalami S, Arefmanesh M, Master E, Nejad M. Replacing 100% of phenol in phenolic adhesive formulations with lignin. Journal of Applied Polymer Science. 2017; 134 (30):2-10 - 113.
Xia Q, Chen C, Yao YY, Li J, He S, Zhou Y, et al. A strong, biodegradable and recyclable lignocellulosic bioplastic. Nature Sustainability [Internet]. 2021; 4 (7):627-635. DOI: 10.1038/s41893-021-00702-w